Methods to Improve Ventilation in Patient-Ventilator Management
Learning Objectives
On completion of this chapter, the reader will be able to do the following:
3 Compare the benefits of closed-suction catheters to the open-suction technique.
4 Discuss the pros and cons of instilling normal saline to loosen secretions before suctioning.
5 List the clinical findings that are used to establish the presence of a respiratory infection.
12 Discuss complications associated with the in-house transport of a mechanically ventilated patient.
Key Terms
• Asynchrony
• Hyperosmolar
• Hyperthyroidism
• Iatrogenic
• Ischemia
• Ketoacidosis
• Permissive hypercapnia
• Transpyloric
Clinicians generally use the first 30 to 60 minutes following initiation of mechanical ventilation to gather information that can be used to evaluate the effectiveness of ventilatory support. As discussed in Chapter 8, these data typically involve vital signs, breath sounds, and assessment of respiratory mechanics (i.e., lung compliance [CL] and airway resistance [Raw]. Ventilator graphics can also be a valuable resource when evaluating the patient-ventilator interaction (see Chapter 9).
This chapter provides an overview of ventilatory strategies that can be used to manage patients with various acid-base disturbances. It also includes a discussion of airway clearance techniques, aerosol administration, flexible fiberoptic bronchoscopy during ventilation, patient positioning, and techniques used to assess fluid balance. The importance of ensuring patient comfort and safety, as well as in-house transport of the ventilated patient, are also discussed.
Correcting Ventilation Abnormalities
Once an initial physical assessment is performed, an arterial blood gas (ABG) sample should be obtained to evaluate the patient’s respiratory and acid-base status. Evaluation of ABG results can be divided into three parts: acid-base status, ventilation, and oxygenation status—pH (alkalinity and acidity), and bicarbonate; PaCO2 (partial pressure of carbon dioxide); and oxygenation status—PaO2 (partial pressure of oxygen), SaO2 (arterial oxygen saturation), CaO2 (arterial content of oxygen), and oxygen delivery (DO2). The following discussion focuses on those factors that can alter PaCO2 during mechanical ventilation, including total ventilation, physiological dead space, and CO2 production (Fig. 12-1). Methods to improve oxygenation are reviewed in Chapter 13.
Common Methods of Changing Ventilation Based on PaCO2 and pH
A change in minute ventilation () is often required after a patient is placed on mechanical ventilation. It is not uncommon to use full ventilatory support initially and then make adjustments after an initial assessment is performed. The examples provided here represent full support of an apneic patient.
During mechanical ventilation, adjusting the set tidal volume (VT) or rate (f) can be used to correct respiratory alkalosis or acidosis. These changes are based on the following equation*:

With this equation, it is assumed that physiological dead space† and CO2 production (resulting from metabolism) do not change significantly during a short period. The equation can therefore be modified to read:

If it is appropriate to keep rate (f) constant and change VT, then the equation becomes:

If it is appropriate to keep VT constant and change f, then the equation is written:
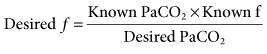
Respiratory Acidosis: Volume and Pressure Ventilation Changes
When PaCO2 is elevated (>45 mm Hg) and pH is decreased (<7.35), respiratory acidosis is present and is inadequate. Acute respiratory acidosis is associated with the following:
• Parenchymal lung problems (e.g., pulmonary edema, pneumonia)
• Airway disease (e.g., severe asthma attack)
• Pleural abnormalities (e.g., effusions)
• Neuromuscular disorders (e.g., spinal cord injury, myasthenia gravis)
• Central nervous system (CNS) problems (e.g., drug overdose).1
See Chapter 4 for additional information.
In patients receiving mechanical ventilation, respiratory acidosis generally can be corrected by altering the set VT or f. Regardless of whether the patient is receiving volume-controlled or pressure-controlled ventilation, increasing will decrease the PaCO2.
Recommended guidelines are to target the VT of 5 to 8 mL/kg ideal body weight (IBW) while assuring that the plateau pressure (Pplateau) is maintained less than 30 cm H2O. As discussed in Chapter 6, tidal volume and breathing frequency adjustments should be based on the patient’s pulmonary pathology. It is important to understand that the 5 to 8 mL/kg range is an average. If the VT is at the upper limit of this range and/or Pplateau is greater than 30 cm H2O, then f should be increased.
With PC-CMV, the set pressure is generally increased to obtain the targeted VT. PC-CMV is time-cycled. If inspiratory time (TI) is short, increasing it may also increase volume delivery, without requiring an increase in pressure (P) (Fig. 12-2).
Respiratory Alkalosis: VC-CMV and PC-CMV Changes
When PaCO2 is decreased (<35 mm Hg) and pH increases (>7.45), then respiratory alkalosis is present and alveolar ventilation is excessive. Common causes of respiratory alkalosis include the following2:
• Hypoxia with compensatory hyperventilation
• Medications (salicylate, xanthines, analeptics)
• Central nervous system disorders (meningitis, encephalitis, head trauma)
• Anxiety
In mechanically ventilated patients, hyperventilation is often the cause of respiratory alkalosis. To correct respiratory alkalosis in this situation, the clinician should decrease minute ventilation during volume-controlled ventilation by decreasing f and, if necessary, by decreasing VT. If pressure-controlled ventilation is used, the clinician should decrease f first and then decrease set pressure, if necessary.
Metabolic Acidosis and Alkalosis
Treatment of metabolic acidosis and alkalosis should focus on identifying those metabolic factors that can cause these acid-base disturbances. Although it may not be apparent, a respiratory component may also be involved, and this possibility should also be addressed.
Metabolic Acidosis
Patients in apparent respiratory distress may present with metabolic acidosis. Blood gases indicate pH = 7.00 to 7.34 and a bicarbonate in the range of about 12 to 22 mEq/L. These patients are often struggling to lower their PaCO2 to achieve some degree of hyperventilation to compensate for the metabolic acidosis. As a consequence, these patients are at risk for developing respiratory muscle fatigue. Thus, in this situation, mechanical ventilation is indicated to meet the minimum goal of compensated hypocapnia.4 Whether or not this can be accomplished with noninvasive ventilation depends on whether the patient meets the criteria for this mode of ventilation (see Chapter 19). If invasive ventilation is required, the risks must be balanced against the temporary benefits.
Causes of metabolic acidosis include the following processes:
• Ketoacidosis (alcoholism, starvation, diabetes)
• Uremic acidosis (renal failure to excrete acid)
• Loss of bicarbonate (diarrhea)
• Renal loss of base following administration of carbonic anhydrase inhibitors (e.g., Diamox)
• Toxins ingested that produce acidosis (salicylate, ethylene glycol [antifreeze], methanol)
Treatment for metabolic acidosis includes initiating effective therapy to deal with the cause of the acidosis and then assessing the need to reverse the acidemia with the administration of an alkalizing agent. Treating the cause and making sure vascular volume and cardiac output are normal, as well as ensuring adequate oxygenation, are essential. These actions allow time for the normal metabolism of organic acids (lactic acid and ketoacids) and allow time for the kidneys to generate bicarbonate to replace losses.4
Controversy abounds regarding the benefit of using alkalinizing agents, such as bicarbonate administration in the treatment of metabolic acidosis. Lowering arterial CO2 is also controversial, but if the patient is losing the struggle to maintain high with spontaneous breathing, assisted ventilation may be necessary to avoid respiratory failure. In this situation, it is appropriate to keep the pH within normal limits (7.35-7.45) (Case Study 12-1).
Metabolic Alkalosis
Metabolic alkalosis is present when pH (7.45-7.70) and bicarbonate (26-48 mEq/L) are elevated above normal. Common causes include the following:
• Loss of gastric fluid and stomach acids (vomiting, nasogastric suctioning)
• Acid loss in the urine (diuretic administration)
• Acid shift into the cells (potassium deficiency)
• Lactate, acetate, or citrate administration
As with metabolic acidosis, treatment involves identifying the underlying cause and reversing those factors leading to the alkalosis. In severe cases, carbonic anhydrase inhibitors, acid infusion, and low bicarbonate dialysis may be required.5
Uncomplicated metabolic alkalosis is not usually associated with alveolar hypoventilation as a compensatory mechanism because of the resulting hypoxemia that occurs with severe hypoventilation. For example, PaCO2 will usually not rise higher than 55 mm Hg. Remember that the PaO2 will fall as the CO2 rises. Thus, hypoventilation is accompanied by hypoxemia in a patient who is breathing room air. The hypoxemia will cause stimulation of the peripheral chemoreceptors, resulting in increased minute ventilation. Only in rare circumstances does full compensation of metabolic alkalosis occur.5
Mixed Acid-Base Disturbances
Some patients with acute respiratory failure have mixed respiratory and metabolic disturbances, such as respiratory acidosis combined with metabolic alkalosis. The pH may actually be near normal. The following case can be used to illustrate this point.
Increased Physiological Dead Space
If pure respiratory acidosis persists even after alveolar ventilation has been increased, the patient may have a problem that is the result of increased alveolar dead space. Increased alveolar dead space can be caused by a pulmonary embolism or low cardiac output resulting in low pulmonary perfusion.
Increased alveolar dead space can also occur when ventilatory support reduces pulmonary blood flow to the lungs by causing high alveolar pressures, such as in the application of high PEEP. Reduced pulmonary perfusion can also be associated with air trapping that results from a high or a low inspiratory gas flow (high inspiratory-to-expiratory [I : E] ratio, such as 3 : 1), or from an uneven distribution of ventilation because of a pathological lung problem.
In the case of air trapping (auto-PEEP), increasing the flow or decreasing the I : E ratio (to 1 : 3 or 1 : 4) may correct the problem. (Increasing inspiratory flow can shorten TI and allow more time for exhalation.) Sometimes repositioning the patient so that the disease-compromised lung receives minimal blood flow (independent position) while the nondiseased lung receives greater blood flow can significantly improve gas exchange and help address the problem. (See section on positioning later in this chapter.)
The normal ratio of dead space to tidal volume (VD/VT) is 0.2 to 0.4. In critically ill patients, this value may be greater than 0.7. Calculation of VD/VT ratio uses the Enghoff modification of the Bohr equation: VD/VT = (PaCO2 − )/PaCO2, where
is the partial pressure of CO2 in the mixed expired gases collected from the patient.* A blood gas sample is collected and the average tidal volume measured while the
is being measured. Note that although this method of measuring VD/VT ratio can provide useful information on mechanically ventilated patients, it typically requires additional equipment and is not routinely performed.
It is more common in ventilated patients to monitor the end-tidal CO2 (normal PETCO2 = 35 to 43 mm Hg) and the gradient between arterial and end-tidal CO2 to determine whether dead space is changing (normal PaCO2-to-PETCO2 gradient = 1-5 mm Hg). A decrease in end-tidal CO2 and an increase in the PaCO2-to-PETCO2 gradient suggest increased dead space. Some clinicians use the mean value of the PETCO2 instead of measuring mixed expired gas to calculate the VD : VT ratio.6 Advances in capnography using single-breath volumetric CO2 monitoring offers still another alternative for estimating VD/VT. (See Chapter 10 for more details on PETCO2 and volumetric CO2 monitoring.)
Increased Metabolism and Increased Carbon Dioxide Production
Metabolic rate and are elevated in patients who have fever, burns, multiple trauma, sepsis, hyperthyroidism, muscle tremors or seizures, agitation, and in those patients who have undergone multiple surgical procedures. Regardless of the cause, it is clear that
is increased and WOB will be elevated. Increasing the machine rate will decrease WOB, but auto-PEEP is likely to occur. It would be beneficial, therefore, to add enough pressure support (PS) for the spontaneous breaths to reduce WOB through the ET and circuit. Other options might include switching to PC-CMV and using sedation to reduce the patient’s work.
Intentional Iatrogenic Hyperventilation
Historically, iatrogenic hyperventilation has been used in patients with acute head injury and increased intracranial pressure (ICP). Hyperventilation reduces CO2 in the blood, which in turn is associated with constriction of cerebral blood vessels and a decrease in blood flow to the brain. Although this approach was believed by many clinicians to help lower increased ICP, therapeutic guidelines for head injuries with increased ICP do not recommend prophylactic hyperventilation (PaCO2 <25 mm Hg) during the first 24 hours. Hyperventilation during the first few days following severe traumatic brain injury may actually increase cerebral ischemia and cause cerebral hypoxemia (see Chapter 7 section on closed head injury).
Hyperventilation may be needed for brief periods when acute neurologic deterioration is present and ICP is elevated. Mild hyperventilation (PaCO2 30-35 mm Hg) may be used for longer periods in situations in which increased ICP is refractory to standard treatment, including sedation and analgesia, neuromuscular blockade, cerebrospinal fluid drainage, and hyperosmolar therapy.7 However, the practice of iatrogenic hyperventilation remains controversial.8 Consider the following example.
Permissive Hypercapnia
Occasionally, it becomes impossible to maintain normal PaCO2 levels in a patient without risking lung damage from high pressures and volumes. Patients with acute respiratory distress syndrome (ARDS) or status asthmaticus and sometimes patients with chronic obstructive pulmonary disease (COPD) who require ventilatory support are at risk for ventilator-induced injury. Inappropriate use of mechanical ventilation can result in severe lung injury, activation of inflammatory mediators, and can even potentially lead to multisystem organ failure.9 (See Chapter 17 for additional information on ventilator-induced lung injury.)
A technique referred to as permissive hypercapnia (PHY) has gained popularity as an alternative form of patient management.10 PHY is a deliberate limitation of ventilatory support to avoid lung overdistention and injury of the lung. During PHY, arterial partial pressures of carbon dioxide (PaCO2) values are allowed to rise above normal (e.g., ≥50-150 mm Hg), and pH values are allowed to fall below normal (e.g., ≥7.10-7.30). Patients who do not have renal failure or cardiovascular problems usually tolerate a pH of 7.20 to 7.25. Younger patients may tolerate even lower pH values. Many clinicians who use PHY allow for a gradual rise in PaCO2 because an abrupt increase in PaCO2 is usually not well tolerated.11–12
Alterations in PaCO2 and pH can affect multiple organ systems. Researchers often focus on the effects of severe acidosis because of serious consequences associated with it. Although most investigators agree that a pH of 7.25 or greater is acceptable, no one is certain whether a lower pH is acceptable. Survival without complications has been demonstrated in isolated cases in which the pH dropped as low as 6.6 and the PaCO2 rose as high as 375 mm Hg when oxygenation was well maintained.11,13 Similar findings have been shown in studies of patients with ARDS and acute asthma.14–17
During hypoventilation, increases in PaCO2 are accompanied by a decrease in PaO2, so O2 administration must be provided and oxygenation status monitored carefully. Increases in PaCO2 and decreases in pH that occur in acute respiratory acidosis also cause a right shift in the oxyhemoglobin dissociation curve. Although this shift in the curve facilitates unloading of O2 at the tissue level, it also reduces O2 loading at the lungs and can further compromise gas exchange.
Increases in carbon dioxide have an additional physiological effect. A higher-than-normal PaCO2 stimulates the drive to breathe. Therefore, it is appropriate to provide sedation to patients with acute lung injury (ALI) in whom permissive hypercapnia is being used. The sedation may improve patient comfort (Key Point 12-1).
Procedures for Managing Permissive Hypercapnia
Efforts to maintain eucapnic breathing (i.e., near a patient’s normal level of PaCO2) might include removing sources of mechanical dead space and increasing the frequency of mandatory breaths.18 When the decision is made to allow PaCO2 to rise above normal, the following strategy may be used19:
Note that use of these buffering agents remains debatable and not well studied in PHY. A short-term increase in PaCO2 might occur when bicarbonate is administered. This is exhaled over time if the level of ventilation is constant. The use of THAM is not associated with an increased PaCO2. It produces intracellular as well as extracellular buffering of pH. Whether or not buffers have any effect on the tolerance of permissive hypercapnia is not known.20A protocol for the implementation of permissive hypercapnia is provided in Box 12-1.21
Contraindications of Permissive Hypercapnia
Carbon dioxide is a powerful vasodilator of cerebral vessels. Thus, increasing CO2 levels can result in cerebral edema and increased ICP, which can aggravate cerebral disorders, such as cerebral trauma or hemorrhage, and cerebral-occupying lesions.19,21 For this reason, the use of PHY is contraindicated in the presence of disorders such as head trauma and intracranial disease. Indeed, it is absolutely contraindicated for those patients who demonstrate intracranial lesions.22
Permissive hypercapnia is relatively contraindicated in patients with preexisting cardiovascular instability. Circulatory effects of PHY can include decreased myocardial contractility, arrhythmias, vasodilation, and increased sympathetic activity. A common finding in patients receiving PHY is increased cardiac output, a normal systemic blood pressure (BP), and pulmonary hypertension.20,23 If the patient’s sympathetic response is impaired or blocked, or if cardiac function is impaired, then an increase in cardiac output might not occur, allowing the vasodilatation to result in hypotension.19
The exact response of the cardiovascular system to permissive hypercapnia is difficult to predict; therefore PHY should be used with caution. This is particularly true when working with patients with any of the following cardiovascular conditions: cardiac ischemia, left ventricular compromise, pulmonary hypertension, and right heart failure.22
Finally, it is worth mentioning that elevated CO2 or decreased pH may affect regional blood flow; skeletal and smooth muscle function; nervous system activity; and endocrine, digestive, hepatic, and renal system functions. Although these effects have not caused significant concern in the clinical setting, further research in these areas is warranted to improve our understanding of this ventilatory strategy.
Airway Clearance During Mechanical Ventilation
During mechanical ventilation, several techniques can be used to help clear secretions from the airway. These procedures differ somewhat from those used with nonintubated, spontaneously breathing patients. Included in this section are discussions of the following topics: suctioning, aerosol delivery, postural drainage and percussions, and fiberoptic bronchoscopy.* High-frequency percussive ventilation can also assist with secretion clearance.
Secretion Clearance from an Artificial Airway
Clearing secretions from the ET or tracheostomy tube of mechanically ventilated patients is an important component of bronchial hygiene therapy. Although it is not uncommon to see a physician’s order read “Suction Q 2 hr,” suctioning at fixed intervals is not appropriate and should be performed only when necessary (i.e., based on patient assessment findings).
Suctioning a patient’s artificial airways involves insertion of a suction catheter into the patient’s trachea and the application of negative pressure as the catheter is gradually withdrawn.25 Suctioning a patient with an artificial airway can involve shallow suctioning, in which the catheter is inserted to a depth that approximates the length of the artificial airways, or deep suctioning, where the catheter inserted until a resistance is met. In the latter case, once the resistance is encountered, the catheter is withdrawn approximately 1 cm before applying negative pressure.25
Two methods of suctioning are typically described based on the type of catheter used: the open suctioning technique and the closed suctioning technique. The open-circuit technique requires disconnecting the patient from the ventilator; the closed-circuit technique can be performed without removing the patient from the ventilator. With the closed-circuit technique, a sterile, inline suction catheter is incorporated into the ventilator circuit, thus allowing passage of the catheter into the ET and trachea without disconnecting the patient from the ventilator (Key Point 12-2).
Suction catheters are generally made of transparent flexible plastic that is rigid enough to allow it to be easily inserted into the artificial airway but flexible enough to negotiate turns and not cause trauma to the airway. Catheters are smooth tipped with two or more side holes near the distal end (Fig. 12-3). (It is thought that these smooth-tipped catheters with side holes may help reduce trauma to the mucosa.)26
The proximal end of the catheter connects to a collecting canister via large-bore plastic tube. A thumb port located at the proximal end of the suction catheter allows the operator to control the suction pressure. When it is covered, suction pressure is applied to the catheter and into the airway. The suction pressure applied should be the lowest possible pressure that is required to effectively clear secretions.25 Box 12-2 provides a list of suggested suction pressure levels. These recommended suction levels are based on current practice. It is important to note that to date no experimental studies are available to support these values.
The catheter length should be long enough to reach a main-stem bronchus. This requires a catheter length of about 22 in. (56 cm).26 Note that in infants and in patients with recent tracheal reconstructive surgery or pneumonectomy, the suction catheter should not be inserted more than 1 cm below the distal tip of the ET.27
Remember that the left main-stem bronchus is narrower and branches at a more acute angle than the right bronchus. Consequently, suction catheters often enter the right rather than the left bronchus. A special-tipped suction catheter is available with a bend at the distal end to help facilitate left bronchial suctioning, particularly if the patient is supine or lying on the left side or if the head is turned to the left. Left bronchus suctioning is easier when the patient has a tracheostomy tube in place rather than an ET.27
The diameter of the suction catheter selected is governed by the internal diameter of the artificial airway. It is generally accepted that the diameter of the suction catheter should not exceed 50% of the internal diameter of the artificial airway for children and adults and 30% of the internal diameters for infants.25 Suction catheter sizes are based on French units. French units refer to the circumference of the tube. (NOTE: Circumference equals diameter multiplied times 3.1416 [π].) Because ETs are sized in centimeters and suction catheters are sized in French units, a conversion is required to estimate the correct size (Box 12-3).
Suctioning should be preceded by hyperoxygenation with 100% O2 for 30 seconds, followed by hyperoxygenation with 100% O2 for 1 minute after suctioning is complete, especially in patients who are hypoxemic before or during suctioning.28 This can be done manually with a resuscitation bag, although this approach does not guarantee VT or pressure, and it has been shown to be ineffective in delivering an FIO2 of 1.0.25 Hyperoxygenation is therefore best accomplished using a temporary oxygen-enrichment program that is available on many microprocessor ventilators.
The duration of suctioning should be brief and must not exceed 15 seconds.25 Shallow suctioning is recommended over deep suctioning, particularly because deep suctioning has not been shown to be superior and may be associated with significantly greater chance of trauma to the tracheal mucosa. Suction should be applied intermittently rather than continually, and the catheter should be rotated as it is withdrawn.29
Hazards and Complications of Suctioning
Loss of suction pressure may be caused by a leak in the system or because the collection canister is full. All connections should be checked, including ensuring that the suction jar is properly seated and screwed on tightly. In cases where the collection canister is full, a float valve at the top of the canister will close the suction line to prevent the transmission of suction to the wall connection line.
Suctioning is a very irritating experience for the patient and can cause a great deal of discomfort and anxiety. Stimulation of the airway with the catheter commonly induces coughing and can result in bronchospasm in patients with reactive airways. Suctioning can also cause hemorrhage, airway edema, and ulceration of the mucosal wall if it is performed improperly.30
The severity of the complications associated with suctioning generally is related to the duration of the procedure, the amount of suction applied, the size of the catheter, and whether or not oxygenation and hyperventilation are done appropriately. Reductions in lung volume can occur with suctioning and lead to atelectasis and hypoxemia. Note that to avoid atelectasis, the clinician should limit the duration of suctioning and the amount of negative pressure applied to the patient’s airways. Hyperoxygenation and hyperventilation of the patient before and after suctioning can also reduce many of the complications associated with suctioning. It is also important to recognize that there is a temporary loss of applied PEEP when a patient is disconnected from the ventilator, which in turn can increase the severity of hypoxemia.
Cardiac arrhythmias can also occur during aggressive suctioning. Tachycardia is generally attributed to hypoxemia and from the irritation of the procedure; bradycardia can occur when the catheter stimulates vagal receptors in the upper airways.31 Hypotension may occur as a result of cardiac arrhythmias or severe coughing episodes. Hypertension may occur because of hypoxemia or increased sympathetic tone resulting from stress, pain, anxiety, or a change in hemodynamics from hyperinflation32,33 (see Case Study 12-2).
Secretion removal is critical in patients with small airways, particularly children, because of the smaller luminal ETs. Suction catheters can even result in pneumothorax in infants if the suction catheter perforates a bronchus.27 Cross contamination of the airway can occur if suctioning is not performed using sterile conditions.34
As previously mentioned, patients with closed head injuries usually have increased ICP. The simple process of inserting the suction catheter without suction being applied in patients with severe brain injury can raise the increased mean intracranial pressure (MICP), the mean arterial pressure (MAP) and the cerebral perfusion pressure (CPP).32,35 This is particularly worrisome in this group of patients. If ICP is being monitored, pressures should be observed before and during suctioning. Oxygenating and hyperventilating the patient are important in this situation. It may even be appropriate to pretreat the patient with topical anesthetic approximately 15 minutes before the procedure to help reduce the risk of increasing ICP.36,37
Closed-Suction Catheters (In-line Suction Catheters)
The closed-suction procedure is considered equally effective as the standard open-suction procedure.38,39 The closed-suction procedure uses in-line catheters that are encased in clear plastic sheaths. The plastic sheaths are attached to special assemblies that connect to a patient’s ventilator circuit, near the Y-connector (Fig. 12-4).40 Notice that inline catheters may add weight and increase the tension on the ET.
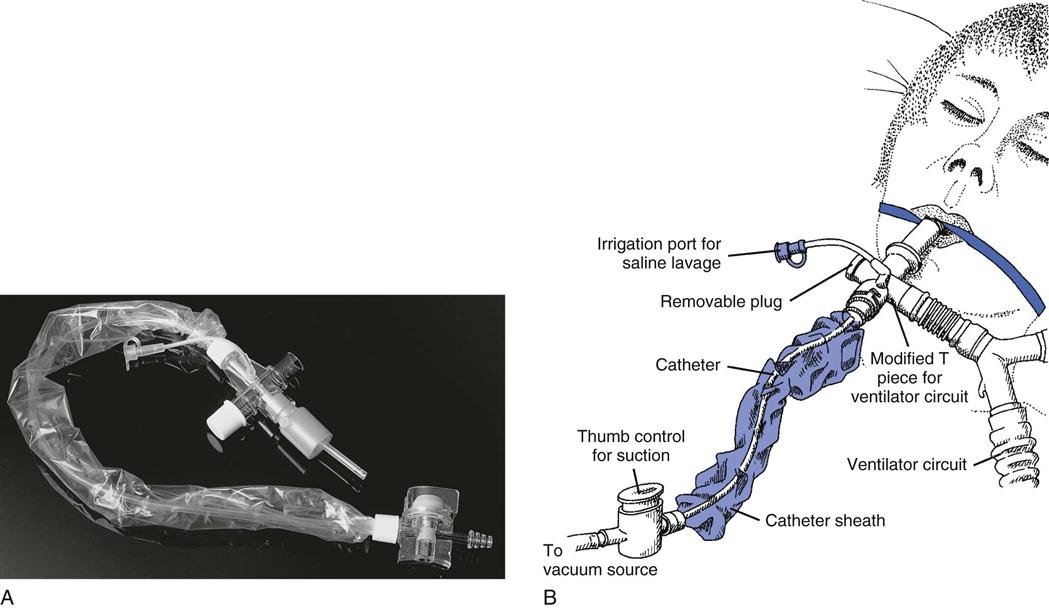
(A from Cairo JM, Pilbeam SP: Mosby’s respiratory care equipment, ed 8, St Louis, 2010, Mosby. B from Sills JR: The comprehensive respiratory therapist exam review: Entry and advanced levels, ed 5, St Louis, 2010, Mosby.)
The advantage of using the closed-suction technique is that disconnecting the patient from the ventilator can be avoided. This is especially important in patients receiving high FIO2s and PEEP because disconnection increases the likelihood of hypoxia and alveolar collapse. Another advantage of this technique is that it reduces the risk for contaminating the airway and lungs when patients are disconnected from the ventilator. For example, using a manual resuscitation bag may introduce contamination into the patient’s lower airways when a single-use disposable suction catheter, which is accidentally contaminated by the handler, is used to suction a patient. Additionally, aerosolized particles from the ventilator circuit can be released into the air during disconnection of the ventilator circuit, thus presenting a potential risk of contamination to the caregiver. Using inline suction avoids these problems and has been shown to reduce the incidence of ventilator-associated pneumonia (VAP).41 Specific indications for closed-suction catheters are listed in Box 12-4.42
