The Central Airways
Computed Tomography Techniques
Imaging of the upper airways provides information that can lead to safer bronchoscopic evaluation, airway dilatation, or surgical repair for patients with airway compromise. Occasionally, imaging may preclude the need for bronchoscopy as a diagnostic tool. Although multidirectional tomography used to be the mainstay for imaging the upper airways, this equipment is rarely found now in most modern hospitals, and planar tomography is currently used (Fig. 16.1). This type of equipment is best suited for renal and musculoskeletal applications but is not optimal for evaluating the thorax. Moreover, because of its infrequent use in the thorax, there is a general lack of expertise on the part of the radiologic technologists obtaining the studies and the radiologists interpreting the examinations. High kilovoltage (150 kVp or greater) radiography with heavy filtration (using aluminum, brass, or copper) can provide high quality tracheal images, although in practice it is rarely used. Fluoroscopy is sometimes a useful dynamic method to evaluate for tracheomalacia, particularly in pediatric patients (1).
Ultrasound may be helpful in patients who are unable to breath-hold or lie flat for computed tomography (CT) evaluation. However, limitations of this technique include nonvisualization of the intrathoracic trachea, poor visualization of posterior wall lesions, and suboptimal evaluation of calcium. One report suggested that ultrasound may be useful as a follow-up investigation in some patients (2). Magnetic resonance imaging (MRI) produces excellent soft tissue contrast of structures and has the capability of imaging in multiple planes. Typically, T1-weighted images, before and after contrast, are most helpful (3). Unfortunately, limitations of MRI include potentially long imaging times, motion artifact, suboptimal evaluation of calcifications, and suboptimal spatial resolution as compared with CT (Fig. 16.1). Therefore, MRI currently has limited usefulness in this setting. For all intents and purposes, CT has virtually replaced all other modalities for imaging the tracheobronchial tree at the current time. In addition to its ability to image the airways, CT also enables visualization of extraluminal spread of disease.
CT is the modality of choice for evaluating the tracheobronchial tree.
Most published studies concerning CT evaluation of the central airways have used conventional CT scanners. On such equipment, a limited number of sections (typically three to six) can be obtained during a single breath-hold, necessitating pauses in scanning to allow the patient to breath. There may be significant misregistration between sections obtained on different breath-holds, leading to overlap and/or gaps between adjacent images. Unfortunately, such misregistration leads to highly suboptimal reconstruction of the CT data in other planes, and therefore the data are usually displayed only in the axial plane. In general, due to their orientation along the long axis of the body, the trachea and
main bronchi are better suited to demonstration in the coronal or oblique planes rather than the axial plane. In addition, the necessity for frequent pauses for breathing leads to the use of relatively thick (5 to 10 mm) sections to cover the area of interest in a timely fashion. Despite these limitations, conventional CT is useful for evaluating focal and diffuse tracheal stenoses and masses and for assessing disease involvement of adjacent structures.
main bronchi are better suited to demonstration in the coronal or oblique planes rather than the axial plane. In addition, the necessity for frequent pauses for breathing leads to the use of relatively thick (5 to 10 mm) sections to cover the area of interest in a timely fashion. Despite these limitations, conventional CT is useful for evaluating focal and diffuse tracheal stenoses and masses and for assessing disease involvement of adjacent structures.
Recent technologic advances have led to the development of helical CT scanners (both single and multislice) that enable rapid aquisition of a large number of thin sections. Thus, an entire study can be performed during a single breath-hold, thereby eliminating misregistration artifacts from varying degrees of respiration. The technique can be particularly useful in patients with multiple stenoses, in whom a proximal stricture is not traversable by a bronchoscope. An entire set of images can be obtained in expiration and in inspiration to look for dynamic changes suggestive of tracheomalacia or bronchomalacia. Helically acquired CT data can be used to produce high quality multiplanar reconstructions (MPRs) (two-dimensional images) oriented along any desired axis and three-dimensional reconstructions and virtual reality bronchoscopic views of the airway lumen. MPRs occasionally provide information that is not evident on the axial images, although generally the addition of MPRs does not lead to significant increase in diagnostic accuracy when assessed by experienced radiologists. However, MPRs may be helpful in detecting mild stenoses, in more accurately depicting the length of tracheal lesions, and in detecting tracheal webs; MPRs are
extremely useful in demonstrating findings to referring clinicians (Fig. 16.2). Three-dimensional images, including shaded surface displays, minimum intensity projections, volume rendering, and virtual bronchoscopy (endoluminal three-dimensional views) (Fig. 16.3), provide additional ways to display the helically acquired CT data.
extremely useful in demonstrating findings to referring clinicians (Fig. 16.2). Three-dimensional images, including shaded surface displays, minimum intensity projections, volume rendering, and virtual bronchoscopy (endoluminal three-dimensional views) (Fig. 16.3), provide additional ways to display the helically acquired CT data.
![]() Figure 16.2 Tracheal web. Multiplanar reformat in the coronal plane demonstrates a fine tracheal web that was not apparent on axial images. |
All these techniques have potential pitfalls in interpretation, require accurate setting of thresholds to provide a true representation of the data, and may be time consuming to produce at a workstation (4,5,6,7). The virtual bronchoscope has the advantage of passing bronchial obstructions and stenoses, and both endobronchial and peribronchial anatomy can be studied. Unlike fiberoptic bronchoscopy, however, virtual bronchoscopy is unable to depict mucosal detail and true color. In general, three-dimensional postprocessing methods are time consuming to perform and are rarely required in routine clinical practice, although they may be complementary in some situations. The true incremental yield of such display techniques has yet to be established. Axial images with the addition of two-dimensional
multiplanar reformats are generally sufficient and are quick to produce from the data set (8,9,10,11,12) (Fig. 16.4).
multiplanar reformats are generally sufficient and are quick to produce from the data set (8,9,10,11,12) (Fig. 16.4).
![]() Figure 16.3 Virtual bronchoscopy. A. Axial computed tomography and (B) virtual bronchoscopic image demonstrate a small adenoid cystic carcinoma. |
One drawback of helical CT is the necessity for full patient cooperation, including the ability to breath-hold for at least 15 to 20 seconds (using a single slice scanner) and abstain from swallowing or other gross movement. Hyperventilation before scanning often aids the patient in accomplishing a sufficiently long breath-hold. The new, rapid, multislice helical scanners are useful in reducing the necessary breath-hold time. Examinations performed during free breathing are generally of limited diagnostic usefulness, and patients unable to suspend respiration during scanning are usually better imaged with conventional tomography. Cardiac motion causes some artifacts, although it usually does not compromise the diagnostic quality of the examination.
A suggested helical imaging protocol would include 1 to 3 mm sections from epiglottis to carina (tracheal study) or from mid-trachea to lower lobe bronchi (central bronchial study), depending on the area of interest. Overlapping images are reconstructed and used to create reformatted planar and three-dimensional images. The patient should be scanned with the neck hyperextended for a tracheal study. If the suspected region of interest is in the neck, the patient’s arms should be down; otherwise, the arms should be up. Initially, the patient is scanned in suspended inspiration, using a single breath-hold, if possible, after hyperventilation. The scan is then repeated during expiration. Intravenous contrast material may be given if a mass is suspected and/or if evaluation of the adjacent mediastinal and hilar structures is required (5). Increased pitch leads to increased stair step artifacts, especially in airways that course obliquely, such as the left main bronchus. Thin sections are essential to reduce partial volume averaging, and overlapping reconstructions help to reduce stair-step artifact (13).
Anatomy
The trachea is a midline structure. On the posteroanterior radiograph there is a slight deviation to the right and a smooth indentation on the left wall of the trachea due to indentation of the aortic arch. Location in the anteroposterior axis is variable but is usually midway between the sternum and spine (14). There is marked variation in cross-sectional shape. The most common shapes are round or oval; less commonly the trachea may be horseshoe shaped with a flat posterior wall, an inverted pear shape, or almost square. The length of the trachea as measured on CT is 6 to 9 cm (15). On chest radiography, the upper limits of normal coronal and sagittal diameter in men is 25 and 27 mm, respectively, and in women is 21 and 23 mm, respectively (16). The lower limit of normal is 13 mm for men and 10 mm for women.
The lower limit of normal tracheal diameter is 13 mm in men and 10 mm in women.
The trachea is made up of horseshoe-shaped bands of hyaline cartilage that support the anterior and lateral walls. Posteriorly is the trachealis muscle, which makes up the posterior tracheal membrane. The layers are the mucosa, submucosa, cartilage or muscle, and adventitia. The tracheal wall consists of 1 to 3 mm of soft tissue on CT. Cartilage may calcify; this is most common in older females. The posterior wall is thicker and may be flat, concave, or convex. The intrathoracic portion of the trachea starts at manubrium (17) and divides into the left and right main bronchi at the carina. The carinal angle, measured as the angle of divergence of the main bronchi along their inferior borders, can vary, with a range between 35 and 91 degrees (average, 55 to 60 degrees). In adults, the right main bronchus has a more vertical course than the left. In children up to age 15 years, the course is similar bilaterally. The right main bronchus is wider (15.3 mm on the right as compared with 13 mm on the left) (18) and shorter (2.2 cm on the right as compared with 5 cm on the left) than the left main bronchus (19,20). Although there are variations in the normal bronchial branching patterns that may be noted incidentally at bronchoscopy, these are mostly of no clinical significance. Generally, the locations of the bronchopulmonary segments are constant. Classification of the bronchial segments is presented in Table 16.1.
Table 16.1: Jackson and Huber Classification of Bronchial Segments | ||||||||||||||
---|---|---|---|---|---|---|---|---|---|---|---|---|---|---|
|
Congenital Anomalies
There may be either agenesis or aplasia of bronchi (i.e., with either absent or rudimentary lung tissue) with involvement of an entire lung, a lobe, or a single segment. This may be a primary phenomenon or secondary to factors such as a space-occupying lesion, thoracic cage abnormality, oligohydramnios, or reduced perfusion. In pulmonary agenesis, the carina is absent; in aplasia, there is a blind ending pouch. Congential bronchial anomalies are often associated with VACTERL (Vertebral dysgenesis, Anal atresia, Cardiac anomalies, Tracheo Esophageal fistula, Renal anomalies, Limb anomalies) abnormalities (21).
Focal mucoid impaction with a tubular soft tissue structure surrounded by hyperaerated lung should suggest bronchial atresia.
There are many variants of lobar and segmental agenesis, aplasia, and hypoplasia. Bronchial atresia is uncommon; it is possibly related to a vascular insult after 15 weeks’ gestation. There is focal obliteration of a segmental bronchus with normal distal structures. A distal mucoid impaction is generally present, showing an ovoid, round, or branching shape and sometimes containing an air–fluid level (Fig. 16.5). The distal lung, which is aerated via collateral air drift, may be hyperinflated. The most common site is the left upper lobe; 64% involve the left apicoposterior segmental bronchus, 14% the left lower lobe, and 8% the right middle and lower lobes (22). Bronchial atresias are often discovered incidentally, and bronchoscopy is used to exclude an endobronchial lesion (23).
The most congenital variant in tracheobronchial anatomy is the right tracheal (or “pig”) bronchus.
Other congential abnormalities include those of bronchial division. Right-sided isomerism is associated with asplenia, and left-sided isomerism is associated with polysplenia and cardiac abnormalities. The right tracheal bronchus is either a displaced right apical bronchus or a supernumerary bronchus; the incidence of this finding is approximately 0.1% to 2% (24). There may or may not be associated lung tissue; it may be a blind ending pouch. A left tracheal bronchus is rarer (0.3% to 1%) and is always an early origin of the left apicoposterior segmental bronchus. It is often associated with distal lung abnormality such as bronchiectasis, lymphangiectasia, and hyperinflation, possibly due to vascular compression by the left pulmonary artery (25). The bridging bronchus is an ectopic bronchus arising from the left main bronchus, crossing the midline and supplying the right lower lobe; the right main bronchus supplies the right upper and right middle lobes only. The accessory paracardiac bronchus has an incidence of 0.09% to 0.5% (26).
Although often asymptomatic, it may cause hemoptysis, infection, cough, or dyspnea. This is a true supernumerary anomalous bronchus and may or may not ventilate a rudimentary or accessory lobe, separated by a fissure. It arises from the medial wall of the bronchus intermedius (Fig. 16.6). Other minor bronchial branching variations are common (23).
Although often asymptomatic, it may cause hemoptysis, infection, cough, or dyspnea. This is a true supernumerary anomalous bronchus and may or may not ventilate a rudimentary or accessory lobe, separated by a fissure. It arises from the medial wall of the bronchus intermedius (Fig. 16.6). Other minor bronchial branching variations are common (23).
Inflammatory Conditions
Postintubation Stenosis
Tracheal stricture or stenosis is most commonly a complication of tracheal intubation with a cuffed endotracheal tube; tracheostomy tube placement and trauma are less common etiologies. Postintubation stenosis may occur at the level of the tracheostomy stoma or at the level of the balloon for an endotracheal or tracheostomy tube. A stenosis is more likely with higher balloon cuff pressures, when the capillary pressure may be exceeded, leading to necrosis and fibrosis. Occasionally, stenosis is seen at level of the tube tip. The stricture
generally results from pressure necrosis, causing ischemia and scarring. Stenosis can also be caused by inflammation with weakening of the tracheal wall (tracheomalacia) or by granulation tissue, especially at the level of the tube tip (27).
generally results from pressure necrosis, causing ischemia and scarring. Stenosis can also be caused by inflammation with weakening of the tracheal wall (tracheomalacia) or by granulation tissue, especially at the level of the tube tip (27).
The most common cause of focal tracheal stenosis is prior intubation.
![]() Figure 16.6 Accessory paracardiac bronchus. (A and B) Axial computed tomography and (C) coronal reformat shows accessory bronchus arising from the medial wall of the bronchus intermedius. |
Acute stenoses are generally secondary to mucosal edema, granulation tissue, and necrotic pseudomembranes. Mucosal thickening is internal to the cartilage, and there is no change in the size of the tracheal lumen on expiration. Such stenoses may be single or multiple (28) and concentric, eccentric, web-like, or A-shaped, secondary to laterally impacted fractures of cartilage after tracheostomy. Chronic strictures may show cartilage and posterior membrane deformities and associated tracheomalacia (17,29,30).
CT using axial images alone is very accurate (approximately 90%) in detecting benign tracheal stenoses, although thin webs or short segment strictures may be missed. There is little gain to the addition of reformats. Reformats are useful, however, in more accurately depicting the longitudinal extent of the stenosis and may be helpful in detecting thin webs or short segment strictures (31) (Figs. 16.4 and 16.7).
Tracheobronchomalacia
Tracheobronchomalacia is a weakness of the tracheal and bronchial walls and cartilage. Wall collapse is present during forced expiration and may be focal or diffuse. The many causes include primary congenital or secondary to tracheal intubation, chronic obstructive pulmonary disease, trauma, infection, relapsing polychondritis, radiation, tumors, surgery, or tracheobronchial fistula. The incidence increases with age, and it is said to be the fifth most common cause of chronic cough (32). Other symptoms are wheeze or unexplained dyspnea. Complications, secondary to the reduced efficiency of the cough mechanism, are retained mucus, infection, and bronchiectasis (33).
Relapsing polychondritis is a rare disease (fewer than 500 cases have been reported) characterized by recurrent inflammation and destruction of cartilage of the ears, nose, larynx, trachea, and peripheral joints; cardiovascular manifestations (aortitis, vasculitis, valvular insufficiency, and aneurysms) occur in 30% of cases. Respiratory involvement, occurring in 50% to 70% of cases, imparts a poor prognosis and causes approximately 50% of deaths from this disease, generally from recurrent pneumonia (31,33,34,35). The cause of the disease is unknown but appears to be immune mediated. Histologically, the normal collagen is replaced by fibrous tissue. Airway obstruction can occur by three mechanisms. First, inflammatory swelling of the glottic or subglottic area is the most common cause of upper airway obstruction in this disorder. Second, encroachment of the glottic or tracheal lumen due to cicatricial contraction may occur late in the course of the disease. Finally, with dissolution of the cartilaginous supporting structure of the trachea, there is dynamic collapse of the airway, especially with forced inspiration and expiration. Granulation tissue and fibrosis may also lead narrowing of the tracheal lumen (17,34,35). Tracheal stenosis with relapsing polychondritis is usually a late manifestation of the disease and reflects diffuse tracheal involvement; however, localized stenosis occurring in the proximal, middle, or distal trachea may also occur. CT findings include thickening of the tracheal wall with or without calcifications; the posterior membranous wall of the trachea is spared
(Fig. 16.8). There may be focal or diffuse tracheal narrowing, with or without expiratory airway collapse (28).
(Fig. 16.8). There may be focal or diffuse tracheal narrowing, with or without expiratory airway collapse (28).
![]() Figure 16.8 Relapsing polychondritis. Axial computed tomography demonstrates thickening of the tracheal wall with sparing of the posterior membrane. |
Tracheobronchomalacia can be evaluated using bronchoscopy, fluoroscopy, and CT. Dynamic CT performed during inspiration and expiration may offer a useful alternative to bronchoscopy and fluoroscopy (Fig. 16.9). CT is performed either through the entire trachea with table movement or at a fixed point with the table stationary (note the trachea moves cranially on expiration; therefore, table position needs to compensate for this).
Images during full inspiration, followed by dynamic expiration (after patient coaching in the technique) are obtained (36,37,38). Aquino et al. (39) demonstrated that a reduction in tracheal cross-sectional area of greater than 18% in the upper trachea and 28% in the mid-trachea gave a high probability for malacia (89% to 100%).
Images during full inspiration, followed by dynamic expiration (after patient coaching in the technique) are obtained (36,37,38). Aquino et al. (39) demonstrated that a reduction in tracheal cross-sectional area of greater than 18% in the upper trachea and 28% in the mid-trachea gave a high probability for malacia (89% to 100%).
A decrease in airway diameter from inspiration to expiration is a sign of tracheomalacia.
Wegener Granulomatosis
Wegener granulomatosis (WG) is a vasculitis involving the upper and lower respiratory tract. The kidneys and other organs are usually also involved. It involves the nasal sinuses and less commonly the nasal septum, uvula, subglottic larynx, trachea, and bronchi (Chapter 13) (33). Clinically, patients present with hoarseness, stridor, and upper airway obstruction. The tracheobronchial tree is affected by an ulcerating tracheobronchitis, subglottic stenoses, inflammatory pseudotumors, tracheal and bronchial stenoses without inflammation, bronchiectasis, and hemorrhage. Diffuse involvement is rare and late. Stenoses in the subglottic region may involve the adjacent vocal cords. Tracheal stenoses may extend into the main bronchi and are typically circumferential, either concentric or eccentric; there may be mucosal irregularity or ulceration (Figs. 16.10 and 13.9). Cartilage and paratracheal soft tissues are less commonly involved (40,41,42).
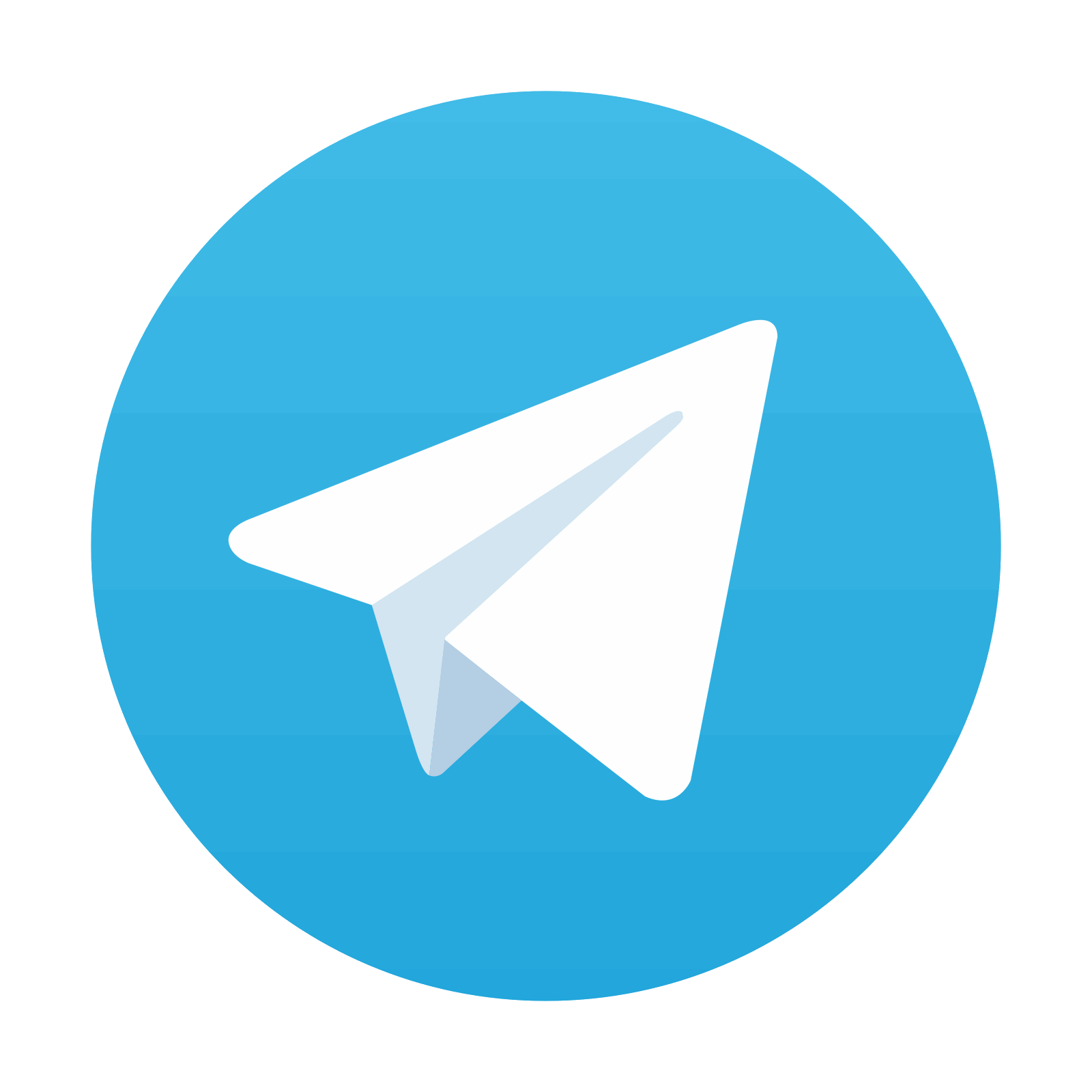
Stay updated, free articles. Join our Telegram channel

Full access? Get Clinical Tree
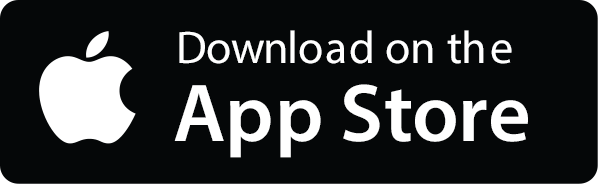
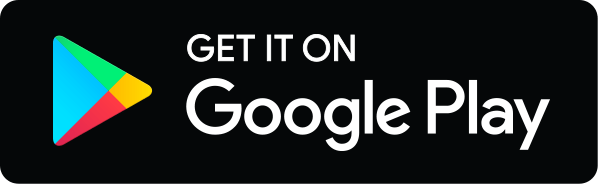