We aimed to characterize the extent and distribution of focal basal left ventricular (LV) hypertrophy in patients with mitral valve prolapse (MVP). Sixty-three patients (mean age: 58 ± 14 years) with MVP and 20 age-matched normal volunteers (mean age: 53 ± 11 years) were assessed using cardiac magnetic resonance imaging. We compared the ratio of basal to mid end-diastolic wall thickness in both groups and correlated it with clinical and imaging parameters. Of the 63 patients, 44 (70%) had posterior leaflet prolapse, 2 (3%) had anterior leaflet prolapse, and 17 (27%) had bileaflet prolapse. There was a significantly increased ratio of basal to mid-ventricular end-diastolic wall thickness in all segments of the left ventricle in those with MVP compared to the controls. The inferolateral (2.1 vs 1.0, p <0.01) and anterolateral (2.1 vs 1.1) ratios (p <0.01) were the greatest compared to the other myocardial segments. The degree of mitral annular excursion had a strong positive correlation with the degree of hypertrophy (r 2 = 0.81, p <0.01) and was an independent predictor in adjusted multivariate analysis (p <0.0001). Age, body mass index, LV end-diastolic volume index, LV end -systolic volume index, LV stroke volume index, degree of prolapse, and mitral regurgitation volume did not have any significant correlation with the degree of hypertrophy. In conclusion, MVP is associated with concentric basal LV hypertrophy and good correlation between the excursion of the mitral valve annulus and the degree of relative LV hypertrophy suggests that locally increased myocardial function could be responsible for this remodeling.
Mitral valve prolapse (MVP) often results in mitral regurgitation, which can lead to left ventricular (LV) dilation and an increase in LV ejection fraction. Progressive LV remodeling can eventually lead to LV systolic dysfunction. Another adaptive mechanism in the setting of MVP, even in the absence of mitral regurgitation, can be LV hypertrophy and increased LV mass. This is evidenced by the observation that performing mitral valve repair in these patients is associated with a greater likelihood of significant regression of LV mass. A confounding problem is that LV hypertrophy in these patients can be attributed to other causes. Recently, a case series of 2 patients described segmental hypertrophy isolated to the posterobasal LV free wall and ascribed it to a novel form of hypertrophic cardiomyopathy. Both patients had MVP and negative genetic testing for known mutations for sarcomeric hypertrophic cardiomyopathy or its phenocopies. Perhaps, the hypertrophy in these patients is secondary to a compensatory mechanism for MVP and not due to a rare genetic condition. The objectives of our study were to (1) characterize the extent and distribution of LV hypertrophy in patients with MVP, and (2) assess the correlation between the degree of LV hypertrophy and myocardial structural parameters.
Methods
We retrospectively analyzed all patients with MVP referred for cardiac magnetic resonance evaluation from January 2008 to August 2011. The study was approved by the institutional review board of St. Luke’s and Roosevelt Hospitals. The patients were classified as having MVP if the mitral valve leaflet displacement >2 mm in the 3-chamber long-axis view. They were further stratified as to having anterior, posterior, or bileaflet prolapse. We also included 20 normal volunteers who served as an age-matched control group.
Each patient was imaged with a 1.5 Tesla scanner using an 8-element, phased-array coil (GE Signa, EXCITE, GE Medical Systems, Milwaukee, Wisconsin). A steady-state free precession pulse sequence was used to acquire short- and long-axis cine images with the following parameters: repetition time/echo time 3.3 ms/1.4 ms, 20 views per segment, field of view 35 × 35 cm, acquisition matrix 192 × 160, slice thickness 8 mm, slice gap 0 mm, flip angle 45°, bandwidth 125 kHz. Phase-contrast images were acquired perpendicular to the proximal pulmonary artery and perpendicular to the proximal aorta to quantify flow in these vessels using the following parameters: repetition time/echo time 7.5 ms/2.9 ms, 6 views per segment, encoded velocity 250 cm/s, field of view 35 × 35 cm, acquisition matrix 256 × 128, slice thickness 4 mm, flip angle 20°, receive bandwidth 31.3 kHz. After the clinical scan was completed, additional phase-contrast images were acquired of a stationary bottle of water (phantom) for baseline correction. If clinically indicated, a contrast-enhanced T 1 -weighted inversion recovery gradient-echo sequence was used to delineate the presence, location, and extent of myocardial fibrosis using the following parameters: repetition time/echo time 5.4/2.5 ms, flip angle 30°, 8 to 10 contiguous slices from base to apex similar to the cine steady-state free-precession sequence, slice thickness 8 mm, acquisition matrix 192 × 128, field of view 35 cm. The inversion time was manually adjusted to a null signal from normal myocardium, and short-axis images were obtained 5 to 10 minutes after intravenous administration of gadolinium contrast (0.2 mmol/kg Magnevist, Berlex Laboratories, Montville, New Jersey, or 0.1 mmol/kg Multihance, Bracco Imaging, Milan, Italy).
The images were reviewed and analyzed using ReportCard, version 4.0, software (GE Healthcare, Waukesha, Wisconsin). LV volumes were determined by manual segmentation of the short-axis images and indexed to the body surface area. Aortic and pulmonary artery flow values were determined using the resident semiautomated algorithm. Correction for baseline offsets was performed using a phantom phase-contrast image, as described previously. The mitral regurgitation volume was defined as the difference between the LV stroke volume and total pulmonary artery flow.
To measure relative LV hypertrophy, we calculated a ratio of basal to mid-ventricular end-diastolic wall thickness in the anterior, anterolateral, inferolateral, inferior, inferoseptal, and anteroseptal segments using long-axis images. A second blinded analysis was done of 20 patients to evaluate the reproducibility of this measurement. The degree of leaflet prolapse was measured for both the anterior and posterior leaflets. Mitral annular excursion was also measured, defined as the distance between the position of the mitral valve annulus in end-diastole and end-systole in the 3-chamber long-axis view.
All analyses were performed using a standard statistical package (SPSS, version 16.0, SPSS, Chicago, Illinois). Continuous data are expressed as the mean ± SD and analyzed using the Student t test. Categorical variables are reported as frequencies and percentages. Correlation analyses using Pearson’s correlation coefficient were performed between the ratio of basal to mid-ventricular end-diastolic wall thickness and clinical and imaging parameters, including age, body mass index, LV ejection fraction, LV end-diastolic volume index, LV end-systolic volume index, LV stroke volume index, mitral regurgitation volume, degree of leaflet prolapse, and mitral annular excursion. A multivariate linear regression analysis of the predictors of relative hypertrophy was also performed. Interobserver variability was measured using Bland-Altman analysis. All tests were 2-tailed, and statistical significance was accepted at p <0.05.
Results
A total of 63 patients with MVP and 20 age-matched controls were identified and analyzed. The baseline characteristics of the MVP and control groups are listed in Table 1 . Of the 63 patients, 44 (70%) had posterior leaflet prolapse, 2 (3%) had anterior leaflet prolapse, and 17 (27%) had bileaflet prolapse. Among the 17 patients with bileaflet prolapse, the mean degree of leaflet prolapse was 4.2 ± 1.2 mm for the anterior leaflet and 5.1 ± 1.5 mm for the posterior leaflet. The 44 patients with posterior leaflet prolapse had a mean prolapse distance of 5.6 ± 2.4 mm.
Variable | MVP Group (n = 63) | Control Group (n = 20) |
---|---|---|
Age (years) | 58 ± 14 | 53 ± 11 |
Men | 35 (56%) | 10 (50%) |
Diabetes mellitus | 4 (6%) | 0 |
Hypertension | 11 (17%) | 0 |
Body mass index (kg/m 2 ) | 27.1 | 26.7 |
Left ventricular ejection fraction (%) | 60 ± 6 | 64 ± 4 |
Left ventricular end-diastolic volume index (ml/m 2 ) | 98 ± 22 | 74 ± 10 |
Left ventricular end-systolic volume index (ml/m 2 ) | 39 ± 11 | 27 ± 6 |
Left ventricular stroke volume index (ml/m 2 ) | 60 ± 17 | 47 ± 6 |
Left atrium (cm) | 3.7 ± 0.7 | 3.4 ± 0.4 |
Mitral regurgitation volume (ml) | 25 ± 22 | 0 |
Strong interobserver agreement was found in the quantification of end-diastolic wall thickness for all myocardial segments in patients with MVP and controls. The combined mean end-diastolic wall thickness for all segments was 7.4 ± 1.2 mm for observer 1 and 7.2 ± 1.1 mm for observer 2, with a strong correlation (r 2 = 0.90, p <0.0001). Bland-Altman analysis revealed a strong agreement between observers 1 and 2, with a mean difference of −0.17 ± 0.4 mm.
The mean end-diastolic wall thickness for each myocardial segment is reported in Table 2 for the patients with MVP and controls. There was a significantly increased ratio of basal to mid-ventricular end-diastolic wall thickness in all segments of the left ventricle in those with MVP compared with the controls ( Figure 1 ). The inferolateral (2.1 vs 1.0, p <0.01) and anterolateral ratios (2.1 vs 1.1, p <0.01) were the greatest amongst all myocardial segments. Figure 2 shows a typical patient with MVP with pronounced basal inferolateral and anterolateral hypertrophy.
Myocardial Segment End-Diastolic Wall Thickness (mm) | MVP Group (n = 63) | Control Group (n = 20) | ||
---|---|---|---|---|
Base | Mid | Base | Mid | |
Anterior | 7.9 ± 2.7 | 5.3 ± 1.4 | 5.4 ± 1.0 | 4.8 ± 1.2 |
Anterolateral | 9.8 ± 2.5 | 4.7 ± 1.4 | 5.4 ± 0.9 | 4.9 ± 1.1 |
Inferolateral | 9.5 ± 2.4 | 4.6 ± 1.4 | 5.0 ± 1.0 | 5.0 ± 1.1 |
Inferior | 9.2 ± 2.6 | 5.3 ± 1.6 | 5.3 ± 1.0 | 5.9 ± 1.6 |
Inferoseptal | 7.2 ± 2.4 | 7.3 ± 2.0 | 5.3 ± 1.2 | 6.4 ± 1.6 |
Anteroseptal | 9.9 ± 2.8 | 5.9 ± 1.6 | 5.9 ± 1.8 | 5.7 ± 1.2 |
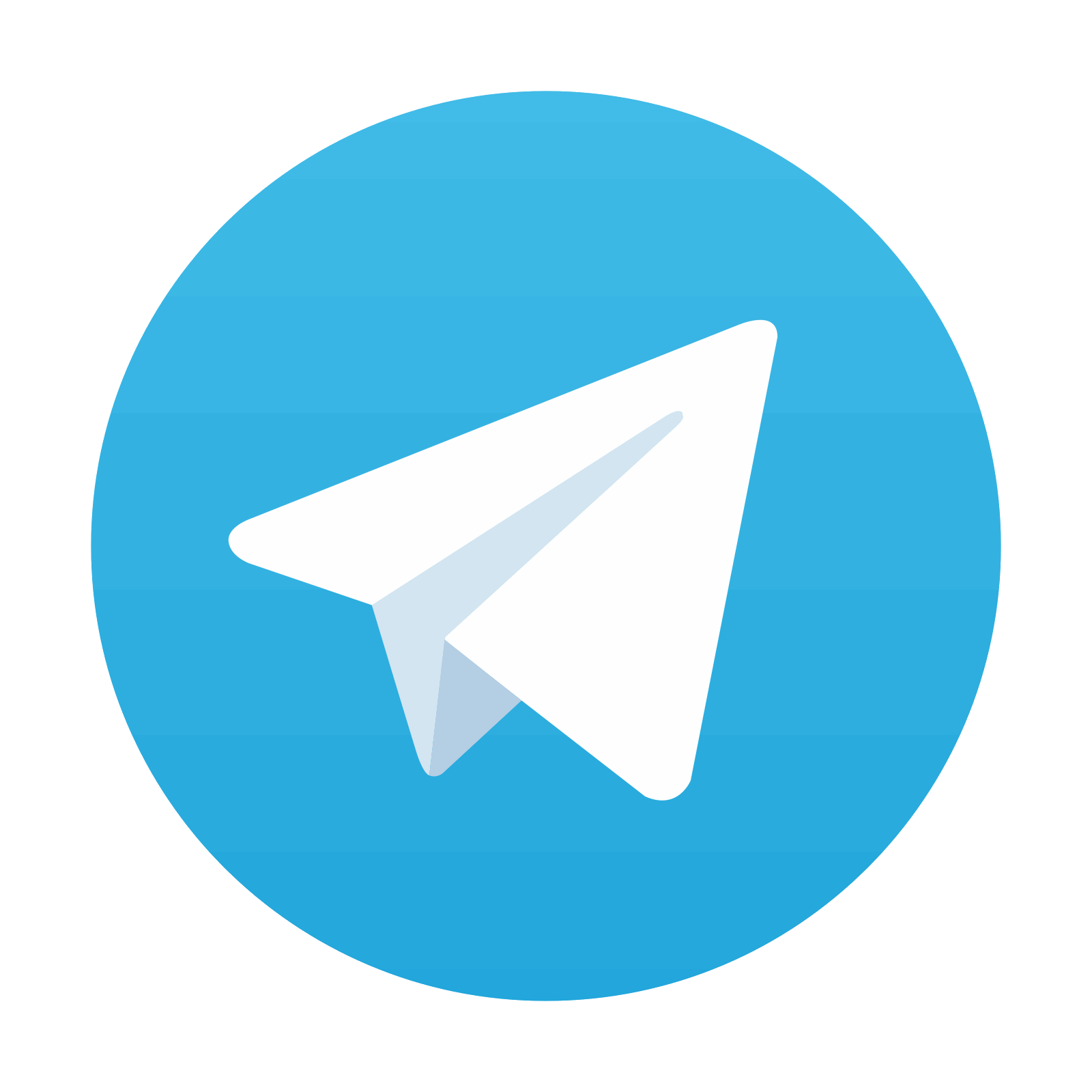
Stay updated, free articles. Join our Telegram channel

Full access? Get Clinical Tree
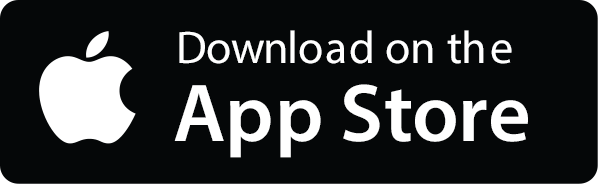
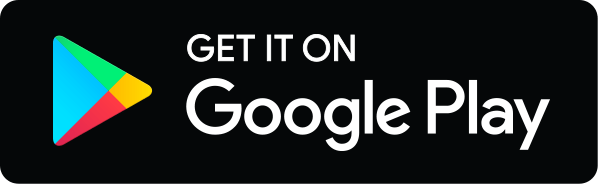
