Robert M. DiBlasi
Neonatal and Pediatric Mechanical Ventilation
Learning Objectives
On completion of this chapter, the reader will be able to do the following:
1. Discuss the clinical manifestations of respiratory distress in neonatal and pediatric patients.
2. Identify differences in the level of noninvasive ventilatory support.
3. Describe device function and settings for different mechanical respiratory support strategies.
4. Identify the primary and secondary goals of ventilatory support of newborn and pediatric patients.
7. Describe the basic design of nasal devices used to deliver CPAP to an infant.
10. Identify the essential features of a neonatal and pediatric mechanical ventilator.
Key Terms
• Bronchomalacia
• Bronchopulmonary dysplasia
• Choanal atresia
• Cleft palate
• Extracorporeal membrane oxygenation
• Meconium aspiration syndrome
• Neonate
• Patent ductus arteriosus
• Pediatric
• Prophylactic therapy
• Rescue therapy
• Tracheoesophageal fistula
• Tracheomalacia
Recognizing the Need for Mechanical Ventilatory Support
Mechanical ventilation of newborn and pediatric patients involves the use of devices that recruit and maintain lung volumes, improve gas exchange and lung mechanics, assist in overcoming the resistive properties of an artificial airway, and reduce the work of breathing. These devices may provide continuous positive airway pressure (CPAP), assist spontaneous ventilation (e.g., noninvasive positive-pressure ventilation [NIV], bilevel positive airway pressure [BiPAP] units), or support part or all of the patient’s ventilatory requirements (e.g., invasive mechanical ventilation).
Currently, there are no well-defined, disease-specific criteria available to guide the decision on when to initiate mechanical vemtilatory support in newborns and pediatric patients in respiratory distress. In fact, many institutions with desirable outcomes prefer to implement ventilatory support before the onset of severe respiratory illness, making the process of initiating support even more complicated. The ongoing clinical management of patients requiring ventilatory support also remains an elusive practice that is based more on experience and clinician preference and less on experimental data obtained from large randomized controlled trials.
This chapter focuses on the best available clinical and experimental evidence for initiating and managing neonatal and pediatric respiratory support. For the purpose of this discussion, a neonate will be defined as any infant born less than 44 weeks of age and pediatric will include any patient beyond the neonatal period and up to adolescence.
Clinicians caring for neonatal and pediatric patients must understand the etiology and pathophysiology of the various diseases and conditions that affect the airways and lung parenchyma of these patients.
They must also be knowledgeable of the theory of operation and limitations of the different ventilatory support devices used. Additionally, clinicians must be able to interpret physiologic data derived from the history and physical assessment, laboratory studies, and radiographic findings to evaluate properly the effectiveness of the ventilatory support provided. Keep in mind that although these data are a critical part of the decision-making process, other factors must be considered when initiating mechanical ventilation in these patients. In many cases, the approach to initiating ventilatory support may have to be individualized for neonates and pediatric patients because anatomic structure, size, and disease severity can vary widely from one patient to the next.
Clinical Indications for Respiratory Failure
Respiratory failure is defined as the inability to establish or maintain adequate gas exchange. Respiratory failure can present at birth and persist throughout the neonatal period or following a catastrophic event. Many pediatric patients encounter respiratory failure as a chronic condition (e.g., bronchopulmonary dysplasia). Although lung disease is the most common cause of respiratory failure, there are many extrinsic factors that can predispose patients to this “life-threatening” event. For example, hemodynamic conditions and congenital cardiac anomalies can also contribute to respiratory failure. Neonatal and pediatric patients have smaller lungs, greater airway resistance, lower lung compliance, less surface area for gas exchange, and lower cardiovascular reserve than do adults, making them more vulnerable to rapid deterioration. In fact, respiratory failure is a major cause of cardiac arrest in neonatal and pediatric patients. As such, clinicians must act quickly to limit the potential adverse outcomes associated with respiratory failure by observing clinical signs and symptoms. These factors can guide timely intervention well before respiratory failure develops into cardiopulmonary arrest.
Neonate
Neonates experiencing respiratory distress present with tachypnea, nasal flaring, and intercostal, substernal, and retrosternal retractions. The chest wall during infancy is composed primarily of cartilage, making the chest wall compliance much greater than that of the lungs. As the resistance and compliance worsen, neonates have to generate higher pleural pressures during inhalation, causing the “floppy” chest wall to collapse inward creating retractions. On exhalation, the neonatal chest wall lacks the necessary recoil to counteract the inward forces of the lungs, and thus the lungs are prone to premature collapse. Infants will attempt to maintain a back pressure in the lungs, to preserve the functional residual capacity, by narrowing the glottis and maintaining respiratory muscle activity (active exhalation). This results in vocalization during exhalation or “grunting,” which is often mistaken for infants’ crying. Grunting can usually be heard without auscultation and is a useful clinical sign of impending respiratory failure. The Silverman Anderson respiratory scoring system is a useful clinical tool to assess the degree of respiratory distress in neonates (Fig. 22-1).
Although this tool has been available in the clinical setting for nearly three decades, it has recently been reintroduced in a number of institutions to better evaluate patient response to settings changes during CPAP and mechanical ventilation.
Premature neonates can become apneic due to underdeveloped neural respiratory centers and may or may not be stimulated to reestablish spontaneous breathing. Infants that do not respond to gentle stimulation and caffeine therapy, often require immediate respiratory assistance using a manual resuscitator and when necessary, invasive mechanical ventilation.
Pediatric
Pediatric patients experiencing respiratory distress can present with some of the same clinical manifestations as neonates. However, larger pediatric patients have ossified or “stiffer” chest walls and are able to sustain longer periods of higher work of breathing (WOB) than neonates. Nonetheless, clinicians should be well versed in recognizing age-specific normal and abnormal respiratory and hemodynamic parameters prior to implementing mechanical respiratory support.
Determining Effective Oxygenation and Ventilation
Arterial blood gas (ABG) analysis is considered the gold standard for determining oxygenation, ventilation, and acid-base balance in neonates and pediatrics with respiratory failure. It is important to recognize that frequent ABGs can deplete the circulating blood volume of small patients. Noninvasive techniques (i.e., pulse oximetry [SpO2] and transcutaneous CO2 measurements) are alternative methods for trending gas exchange in most patients. Interpretation of ABG or noninvasive gas exchange values must also be coupled with data obtained from physical assessment and other clinical and laboratory data. For example, observing the color of the skin and mucous membranes can be used to assess tissue oxygenation; oxygen delivery and tissue perfusion can be evaluated clinically by noting capillary refill. Indeed, close attention to vital signs and physical assessment findings can help prevent deterioration of ABG/acid-base status.
Patients with certain congenital heart defects often require a high pulmonary vascular resistance to prevent excessive pulmonary blood flow and maintain adequate systemic circulation and cardiac output; thus, abnormal values are acceptable in this patient population before surgical correction. Additionally, allowing carbon dioxide levels to rise and pH levels to fall to abnormal levels has become a common standard for lung protection during mechanical ventilation. If necessary, an individualized or standardized approach for managing gas exchange during ventilatory support should be identified early in management.
Chest radiographic evaluation is another important tool that can add to the overall clinical assessment of patients with respiratory failure or those receiving respiratory support. Because lung volumes are difficult to measure in neonatal and pediatric patients, chest radigraphs can provide valuable insight into the approximate level of lung expansion in patients suscepetible to developing atelectasis or hyperinflation. Many clinicians use the chest radiograph to guide setting ventilator parameters. It is important to realize the limitations of chest radiographs and understand that frequent radiography may expose patients to unnecessary high levels of radiation. The following sections provide more in-depth information about clinical and laboratory indications for mechanical ventilatory support.
Goals of Newborn and Pediatric Ventilatory Support
The goals of mechanical ventilatory support in newborn and pediatric patients are:
One may argue that avoiding mechanical ventilatory support altogether or minimizing the duration of support should be the first goal because even short-term ventilation can result in ventilator induced lung injury (VILI).
Maintenance of an appropriate functional residual capacity (FRC) ensures optimum lung mechanics, which leads to reduced WOB and reduced lung injury. To move gas into and out of the lungs, the patient must generate relatively high intrathoracic pressures to balance the resistive and elastic components that resist lung inflation. Positive-pressure ventilation can significantly reduce this burden and improve recovery in patients with lung disease. In fact, some patients receive chronic mechanical ventilatory support as a means of reducing caloric utilization by the respiratory muscles. These patients often are able to breathe spontaneously but at a significant caloric cost. Mechanical ventilatory support can help promote normal function and development of the respiratory system, especially in neonatal patients with lung disease.
Noninvasive respiratory Support
Continuous positive airway pressure (CPAP) is used in spontaneously breathing patients and may be applied with or without an artificial airway. CPAP provides a continuous distending pressure to the lungs, which increases FRC and thus helps improve lung compliance (CL). Often airway resistance (Raw) is also reduced and the patient’s WOB dramatically decreases with the use of CPAP.1 CPAP is most commonly applied noninvasively to the nasal airway opening and has become very popular over the last decade in the neonatal population as a strategy to avoid intubation and invasive mechanical ventilation. In this discussion, the term CPAP refers to nasal CPAP in neonates. CPAP often is recommended for patients who have adequate alveolar ventilation, and yet are hypoxemic despite receiving an F1O2 greater than 0.5. CPAP may be used to prevent atelectasis and to reduce WOB in patients who have been weaned and extubated from the ventilator.
Nasal Continuous Positive Airway Pressure in Neonates
Indications and Contraindications
When introduced in 1971, CPAP was touted as the “missing link” because it could provide oxygen treatment while avoiding mechanical ventilation in the neonate.2 Used appropriately, CPAP is a less invasive and less aggressive form of therapy than other forms of ventilatory support. Newborns with retained lung fluid, atelectasis, insufficient surfactant production, or respiratory distress syndrome (RDS) are good candidates for CPAP. Such patients include very low-birth-weight (VLBW) and premature infants.3 CPAP also can be used successfully in infants with respiratory distress arising from other causes, including transient tachypnea of the newborn, meconium aspiration syndrome, primary pulmonary hypertension, pulmonary hemorrhage, paralysis of a hemidiaphragm, and following surgical repair of diaphragmatic hernias and congenital cardiac anomalies, congenital pneumonias, respiratory syncitial virus (RSV) bronchiolitis, apnea of prematurity, and congenital and acquired airway lesions.4
Box 22-1 lists the indications for CPAP in neonates originally established by the American Association for Respiratory Care (AARC).5
Compared with standard oxygen therapy, CPAP reduces grunting, and tachypnea, increases FRC and arterial oxygen partial pressure (PaO2), decreases intrapulmonary shunting, improves lung compliance, aids in the stabilization of the floppy infant chest wall, improves distribution of ventilation, and reduces inspiratory WOB.4 CPAP is believed to reduce the severity and duration of central and obstructive apneas by mechanically splinting the upper airway, promoting better alveolar recruitment, oxygenation, and stimulation of the infant to breath.6
Although there is no consensus on how best to manage neonates on CPAP, two general approaches are currently being used to minimize the use of invasive mechanical ventilation and better protect the fragile neonatal respiratory system. Early CPAP involves implementing therapy in the delivery room or neonatal intensive care unit (NICU) only after the infant is stabilized and is effectively breathing on his or her own. This is performed prophylactically even if the neonate is not exhibiting respiratory distress or apnea. The goal is to recruit air spaces and maintain lung volumes early to promote gas exchange and reduce the likelihood that respiratory failure and apnea will occur. This approach is beneficial for premature infants that lack lung surfactant and are at risk for developing atelectasis. Breathing at low lung volumes can result in unnecessary lung injury (atelectotrauma), which can hinder surfactant production. Many premature neonates can be managed successfully using CPAP without ever requiring endotracheal intubation and mechanical ventilation. If the patient does develop respiratory failure, then he or she is intubated, given lung surfactant, and then promptly weaned from the ventilator and extubated. This approach is a drastic departure from the previous approach that has been used over the last 20 years, in which neonates would be intubated and placed on a ventilator for weeks or even months until they were a certain size or weight. Centers that implement early CPAP report a very low incidence of infants developing chronic lung disease or bronchopulmonary dysplasia (BPD) because the lungs are not being subjected to the relatively large inflation pressures that are observed during mechanical ventilation.7,8 A recent clinical trial in premature neonates demonstrated that this early CPAP approach resulted in less need for intubation and fewer days of mechanical ventilation, and infants were more likely to be alive and free from the need for mechanical ventilation after a week than were neonates intubated for surfactant and supported with mechanical ventilation for at least 24 hours.9
Another clinical approach implements elective intubation, prophylactic surfactant administration, short-term lung-protective ventilation, and rapid extubation to CPAP within hours of birth. (NOTE: This approach is also known as InSURE (Intubate, SURfactant, Extubation) and is implemented shortly following birth.10) The InSURE approach assures that all premature infants will receive at least one dose of surfactant, but it does not eliminate the potential that even short-term ventilation can result in some degree of lung injury. The InSURE approach has been associated with lower incidences of mechanical ventilation, air-leak syndromes, and BPD than an approach that administers surfactant and embraces prolonged mechanical ventilation support.11
The major question that remains is whether these two disparate approaches impact long-term survival and the development of chronic complications (e.g., BPD). Another important outcome related to these different approaches is the incidence of CPAP failure and subsequent ventilation requirements among neonates. Approximately 25% to 40% of infants with birth weights between 1000 and 1500 g may fail early CPAP and require intubation and mechanical ventilation, whereas 25% to 38% of infants with similar birth weights may fail CPAP using the InSURE approach.4 Some institutions use a combination of these approaches wherein smaller premature neonates (<28 weeks’ gestational age), with lower surfactant production, will be supported initially using InSURE and all other larger neontates are supported using the early CPAP strategy. Both these strategies strive to minimize invasive mechanical ventilation and have redefined the approach to supporting premature infants at risk for developing respiratory failure. Minimally invasive ventilation strategies, such as CPAP, have likely been a major reason why premature neonates are able to survive at a lower gestational age and with fewer complications than ever before.
Any neonate that has recently been extubated from mechanical ventilation is at risk for developing hypoxemia, respiratory acidosis, and apnea. Extubation to CPAP, regardless of whether surfactant was administered, has been associated with a reduction in the incidence of respiratory failure and the need for additional ventilatory support in neonates.12
Infants with certain congenital heart diseases reportedly benefit from CPAP. Cardiac anomalies that increase pulmonary blood flow can reduce lung compliance (CL) and FRC, thus increasing WOB and worsening hypoxia. The most common defects associated with increased pulmonary blood flow are ventricular septal defects, atrial septal defects, atrioventricular (AV) canal, and patent ductus arteriosus. Positive intrathoracic pressure produced by a CPAP system can mechanically reduce pulmonary blood flow while restoring FRC.13,14
Use of CPAP is not appropriate and is potentially dangerous in infants who show signs of nasal obstruction or severe upper airway malformation, such as choanal atresia, cleft palate, or tracheoesophageal fistula.13 CPAP has been used in patients with bronchiolitis, but its use in these patients has been controversial and may be contraindicated in some cases.5,15,16 More recent evidence suggests, however, that CPAP can result in favorable outcomes in infants affected with bronchiolitis by reducing carbon dioxide levels and eliminating the need for mechanical ventilation.17
Patients who have severe cardiovascular instability, severe ventilatory impairment (pH < 7.25, PaCO2> 60), refractory hypoxemia (PaO2 < 50 torr on >0.6 FIO2), frequent apnea that does not respond to stimulation or intravenous caffeine therapy, or are receiving high levels of sedation may require intubation and mechanical ventilation rather than CPAP.13 CPAP or any form noninvasive positive pressure to the airway should not be used in infants with untreated congenital diaphragmatic hernia; these infants should be intubated to prevent gastric insufflations and distention and further compromise of the heart and lungs.15 Some surgeons discourage the use of CPAP in infants after any surgical procedure involving the gastrointestinal tract.
Most commonly, CPAP is applied via nasal prongs; however, some clinicians prefer to use CPAP in intubated neonates while weaning from mechanical ventilation to observe whether the infant is experiencing apnea. Prolonged support using this approach should be discouraged whenever possible because it is has been associated with increased need for reintubation after the breathing tube has been removed.12
Application of Nasal CPAP
Because newborns are obligate nose breathers, nasal CPAP can be applied in several ways. Previously, a short endotracheal tube (ET) was placed into one of the nares and taped to the face to provide nasopharyngeal tube CPAP. A snug-fitting set of short binasal prongs is the most commonly used interface. Neonatal nasal masks are also available and are gaining popularity among some clinicians. It is common practice to alternate between these two nasal airway interfaces. Both devices are effective, but the distending pressure they provide can be lost when the infant cries or breathes through the mouth. Binasal prongs and masks may be more beneficial than a nasopharyngeal tube because they are less invasive and provide the least amount of resistance to gas flow and, hence, lower imposed (or resistive) WOB, and facilitate mobilization and oral feeding.14,18 Additionally, short binasal prongs were found to be more effective than using single nasopharyngeal tube in reducing the rate of re-intubation in premature neonates supported with CPAP.19
Nasal prongs and masks must be fitted correctly so that they do not leak or cause trauma to the patient. Nasal prongs and masks usually are made of a latex-free material, such as silicone. Molded into a manifold or attached to one, the prongs are placed just inside the infant’s nares (Fig. 22-2). Prongs are available in a variety of sizes. The fit of the prongs is critical; they must fit snugly into the nares but must not be so tight as to cause skin blanching. If a nasal mask is used instead of prongs, it, too, must be carefully selected for proper size and fit. Masks can cause pressure injury to the skin if improperly fitted, and they may not seal if they are too large or small.
The entire CPAP apparatus is stabilized on the patient’s head with headgear or a “fixation” system consisting of a bonnet, cap, or straps (Fig. 22-3). Many commercial system configurations are available. The correct size of straps and head coverings must be carefully chosen and adjusted so that no part of the infant’s head is subjected to squeezing or occlusive pressure points. After the CPAP delivery device has been attached to the CPAP manifold, the CPAP level and FIO2 are set. The CPAP system is assessed frequently to ensure effective delivery. The patient’s nose is checked regularly for signs of pressure necrosis, and the prongs must be checked routinely for patency.
The CPAP system functions primarily to regulate gas flow during inhalation and exhalation and to maintain a consistent pressure at the nasal airway opening. The CPAP system consists of five essential components:
1. A heated/humidified blended gas source
4. The pressure regulation mechanism
5. A means for monitoring and limiting the airway pressures4
Numerous studies have compared differences in gas exchange, WOB, and requirement for (re)intubation with the available CPAP devices; however, it is important to note that no single device has been shown to be superior to another when major outcomes (i.e., mortality and morbidity) in neonates are considered (Key Point 22-1).4
Over the last three decades, the most common CPAP system that has been applied noninvasively has been accomplished using a mechanical ventilator. Ventilator CPAP has also been referred to as conventional CPAP in the clinical setting. Ventilator CPAP is convenient because it has traditionally been used following extubation from mechanical ventilation and does not rely on having to use a separate device to apply therapy. Many ventilator manufacturers have designed specific noninvasive CPAP modes into the ventilator platform exclusively for neonates. The clinician can set the CPAP level, and the exhalation valve regulates the pressures accordingly. Another advantage of these modes is that the ventilator may be able to deliver noninvasive “backup breaths,” based on a preset apnea interval when the neonate has stopped breathing. One potential limitation of ventilator CPAP is that the demand flow system may not be able to respond adequately to changes in patient respiratory efforts because pressure is being measured back at the ventilator and not at the patient’s airway. Additionally, new evidence suggests that the expiratory resistance of newer neonatal ventilator’s exhalation valves may impose additional resistance and hence increase the WOB during spontaneous breathing.20
Two commercially available, freestanding CPAP devices that use fluidic gas principles are widely used to provide CPAP to infants. The Hamilton Arabella (Fig. 22-3, A and B) and the CareFusion Infant Flow SiPAP System (Fig. 22-4) are favored by some clinicians. The overall function of these fluidic devices to generate CPAP at the airway is similar. Both devices have a fully integrated flow controller, a delivery circuit, different-sized nasal prongs that attach to a gas delivery manifold, and a bonnet with Velcro straps to secure the manifold and prongs. The manifold incorporates a fluidic flip-flop mechanism at the infant’s nasal airway opening to regulate flow to match the infant’s inspiratory demand and provide a consistent pressure level (see Fig. 22-3, B). The controller provides gas flow with an adjustable FIO2 and also monitors CPAP pressure. Turbulence caused by continuous flow has been eliminated in these systems to make exhalation easier and reduce the WOB. The manufacturers have attempted to provide better-fitting, easier-to-secure nasal prongs to make CPAP more effective and patient care easier. These devices are particularly effective at reducing WOB in VLBW infants. CPAP is better tolerated in these patients, which helps to avert the need for positive-pressure ventilation.21
Bubble CPAP (B-CPAP) is a technique for delivering CPAP via a simple freestanding system (Fig. 22-5). It has been used in certain forms for more than 30 years and is again gaining favor over other CPAP techniques. In the United States, two B-CPAP devices are now available (B&B Medical, Fischer Paykel), but most centers that implement B-CPAP have done so using homemade systems consisting of a blended and humidified gas source, ventilator circuits, nasal prongs, pressure manometer, and a water column. The blended gas flow is adjusted at 5 L/min, and the CPAP level is regulated by varying the depth of the distal ventilator circuit below the water surface (i.e., 5 cm = 5 cm H2O CPAP). Higher pressures than those anticipated by the submersion depth of the distal tubing have been observed when using higher flows22,23; thus clinicians will use the lowest flow possible to maintain constant bubbling throughout the respiratory cycle. An additional safeguard involves measuring the pressure at the nasal airway interface using a pressure manometer and limiting excessive pressures with a safety “pop-off” valve during B-CPAP.
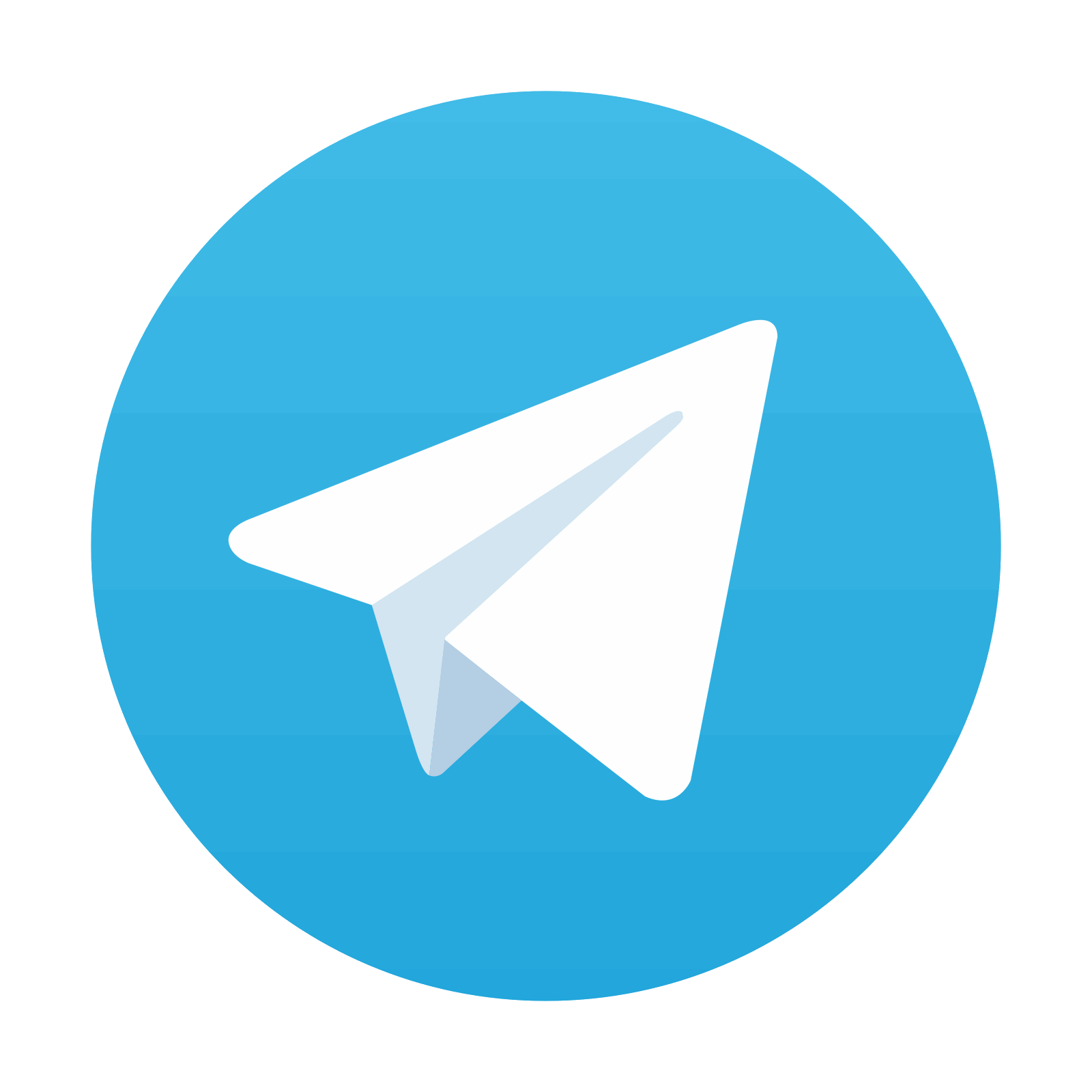
Stay updated, free articles. Join our Telegram channel

Full access? Get Clinical Tree
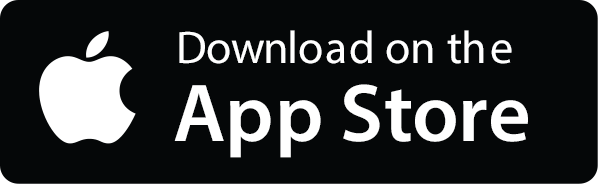
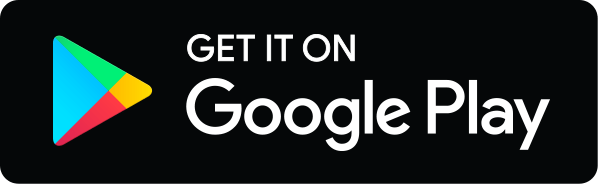