Table 22.1 Use of Echocardiography in the Intensive Care Unit | |||||||||||||||||||||||||||||||||||||||||||||||||||||||||
---|---|---|---|---|---|---|---|---|---|---|---|---|---|---|---|---|---|---|---|---|---|---|---|---|---|---|---|---|---|---|---|---|---|---|---|---|---|---|---|---|---|---|---|---|---|---|---|---|---|---|---|---|---|---|---|---|---|
|
Table 22.2 Appropriateness Criteria for Transthoracic and Transesophageal Echocardiography | |||||||||||||||||||||
---|---|---|---|---|---|---|---|---|---|---|---|---|---|---|---|---|---|---|---|---|---|
|
hypotension in whom the use of intravenous pressors results in no improvement or further deterioration. There is a subset of patients, many of whom have a history of hypertension, who with volume depletion develop acquired dynamic left ventricular outflow tract obstruction which mimics obstructive hypertrophic cardiomyopathy. Systolic anterior motion of the mitral valve with secondary mitral regurgitation also may be seen. The overall hemodynamic result of this syndrome is progressive hypotension with the development of a prominent systolic murmur (due to outflow tract obstruction and/or mitral regurgitation). The etiology of the hypotension in this situation is the relatively low left ventricular stroke volume due to hypovolemia, complicated by outflow tract obstruction. Left ventricular outflow tract gradients exceeding 100 mm Hg have been noted because of this phenomenon. In this instance, right heart catheterization reveals an elevated pulmonary capillary wedge pressure that is then assumed to reflect left ventricular filling volume. When the syndrome of significant mitral regurgitation with outflow tract obstruction is identified, one should recognize that the elevated pulmonary capillary wedge pressure is the result of a hyperdynamic but noncompliant left ventricle and mitral regurgitation. Failure to appreciate this phenomenon results in the inappropriate course of increasing pressor support and diuretics, which obviously has the effect of worsening rather than improving the clinical situation. Figure 22.8 was recorded in a patient with this syndrome. Recognition of hypovolemia with dynamic outflow tract obstruction should lead to the appropriate management decision to resuscitate the patient with fluids and withdraw agents, which increase contractility and/or reduce vascular resistance.
elderly and have a history of hypertension. In the operative or postoperative period, overly aggressive fluid resuscitation may result in congestive heart failure. The echocardiogram will typically reveal normal systolic function and left ventricular hypertrophy (Fig. 22.9). Mitral inflow patterns may be highly variable and show either delayed relaxation or a restrictive filling pattern. If intravascular volume overload is present, a pseudonormal inflow pattern is not uncommon.
with major trauma, hemorrhagic shock is a consideration, in which case echocardiography can quickly document a small, underfilled ventricle. Additionally, in patients with major blunt chest trauma, such as after a high-speed motor vehicle accident, echocardiography can be instrumental in documenting cardiac involvement including myocardial contusion, pericardial effusion, or aortic trauma. By confirming the absence of significant cardiac involvement, echocardiography allows the clinician to redirect efforts to alternate explanations for hypotension.
residual gradients and paravalvular regurgitation. Preoperative echocardiography is instrumental in assessing the indications for, and the likelihood of success of virtually all forms of valve surgery.
Table 22.3 Echocardiography in the Operating Room | ||||||||||||||||||||||||||||||||||||||||||||||||||||||||||||
---|---|---|---|---|---|---|---|---|---|---|---|---|---|---|---|---|---|---|---|---|---|---|---|---|---|---|---|---|---|---|---|---|---|---|---|---|---|---|---|---|---|---|---|---|---|---|---|---|---|---|---|---|---|---|---|---|---|---|---|---|
|
even in the beating heart, the ventricle will appear globally hypokinetic (Fig. 22.21). Once fully removed from bypass and after appropriate volume resuscitation, ventricular size and function should return to baseline. Depending on the nature of the surgery and its success, and the use of inotropic agents, ventricular function may be improved compared with baseline. Partial bypass, or incomplete volume restoration, results in intermediate levels of ventricular performance. While on complete bypass, continuous nonphasic flow will be seen in the aorta, related to the cardiopulmonary bypass cannula flow (Fig. 22.22).
the medial commissures to the right. When viewed with either transesophageal or transthoracic echocardiography, this orientation will be reversed (assuming traditional recommended viewing formats on a video screen). Also, depending on whether the reference is a transthoracic or transesophageal echocardiogram, the anterior and posterior leaflets of the mitral valve will vary in position compared with the surgical perspective.
of the mitral valve from a perspective within the left atrium (Fig. 22.26). Experience suggests that real-time three-dimensional imaging confers an advantage with respect to complete evaluation of mitral valve pathology, including isolated mitral flail chordae and precise localization of flail scallops compared with routine, two-dimensional imaging, although the true clinical impact of this has yet to be demonstrated. Figures 22.27, 22.28 and 22.29 were recorded in patients with mitral pathology and demonstrate the unique capabilities of this type of imaging. Similar images can be obtained from reconstructed images but are
limited by artifacts inherent in stitching subvolumes and the fact that they do not provide true real-time images. Sophisticated on- and off-line analysis systems have been developed for quantitation of the three-dimensional mitral valve data set allowing determined quantitation of the actual amount of mitral valve tissue involved with a flail leaflet as well as the overall area of the mitral valve (Fig. 22.29). Experience to date suggests that this imaging technique confers substantial clinical value with respect to accuracy and speed of anatomical diagnosis, both before and following surgery for mitral valve disease. As current ultrasound platforms are still limited with respect to color Doppler imaging when operating in a threedimensional scanning mode, standard two-dimensional imaging with color flow Doppler is still essential for a complete evaluation.
![]() FIGURE 22.28. Real-time three-dimensional transesophageal imaging in a patient with myxomatous mitral valve disease and pronounced buckling of the posterior leaflet. This image was recorded from the perspective of the surgeon’s view within the left atrium. Note the large, bulky myxomatous posterior leaflet protruding into the left atrium in systole (arrows). The smaller inset is a real-time image of the same patient revealing the myxomatous posterior leaflet buckling into the left atrium (arrow). The intraoperative anatomy is also illustrated for comparison (see Figs. 22.35 and 22.36 for the postoperative images). |
![]() FIGURE 22.31. Color Doppler flow images corresponding to the images presented in Figure 22.30 are shown. A: Note the severe mitral regurgitation arising centrally with the vena contracta location identified by the area of noncoaptation in Figure 22.30. B: A systolic frame recorded after placement of a mitral ring. Note the absence of mitral regurgitation after ring placement. |
valve tissue, this may result in the valve being converted to a nearly unicuspid valve with the anterior leaflet providing the majority of functional valve tissue. More complex repairs may include transposition of a portion of a leaflet and its attached chordae to the opposite leaflet to provide intact chordae to the previously flail leaflet. Finally, prosthetic chords can be attached to a flail mitral leaflet and subsequently to a papillary muscle to replace chordal structures that are damaged beyond repair. The goal of mitral valve repair is to reduce the severity of mitral regurgitation to no more than mild without creating iatrogenic mitral stenosis. In the examples presented, note the smaller annular dimensions due to an annular ring as well as the areas of thickening on the mitral valve that represent areas of resection (Figs. 22.36 and 22.37).
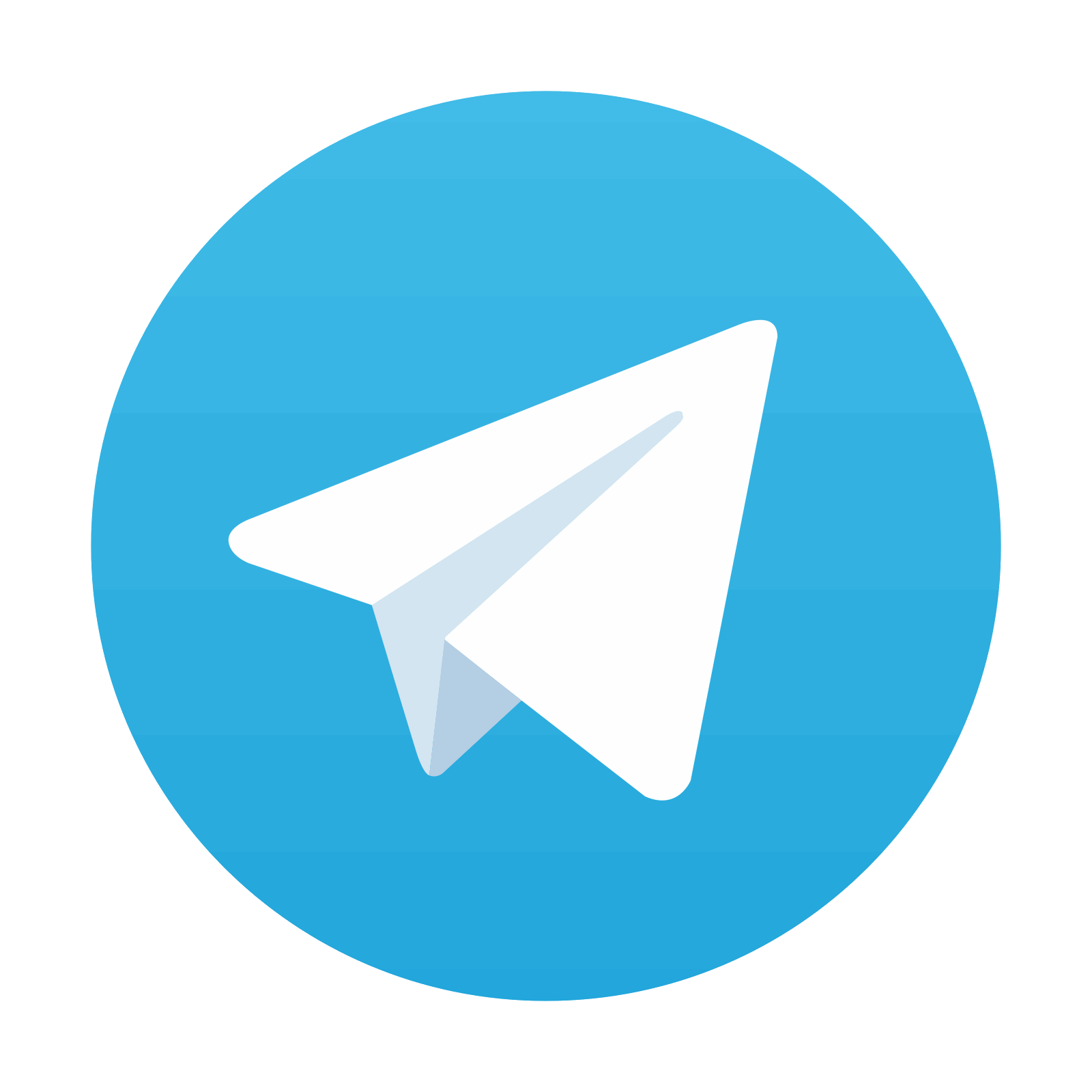
Stay updated, free articles. Join our Telegram channel

Full access? Get Clinical Tree
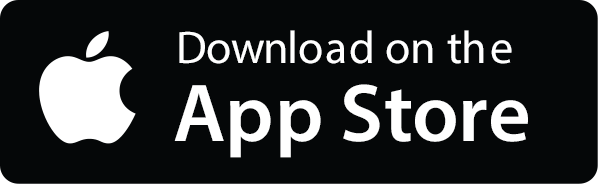
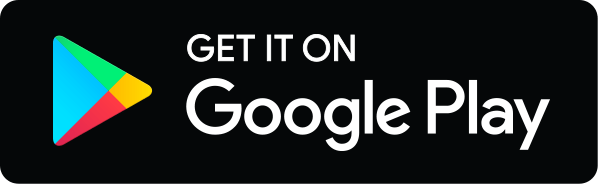