Establishing the Need for Mechanical Ventilation
Learning Objectives
On completion of this chapter, the reader will be able to do the following:
1 Differentiate between acute respiratory failure (ARF) and respiratory insufficiency.
2 Identify goals and objectives of mechanical ventilation.
3 Describe three categories of disorders that may lead to respiratory insufficiency or ARF.
Key Terms
• Acute respiratory failure
• Biot respirations
• Cheyne-Stokes respirations
• Homeostasis
• Status asthmaticus
• Residual volume
• Functional residual capacity
• Vital capacity
• Respirometer
The ability to recognize that a patient requires an artificial airway and mechanical ventilation is an essential skill for clinicians. Although ventilators have been used for more than half of a century, surprisingly little evidence and few precise criteria are available to guide clinicians about when to initiate ventilatory support. Originally, mechanical ventilation was instituted because respiratory failure was seen as a “derangement” of gas exchange in the lungs.1,2 Indeed, clinicians traditionally have relied heavily on arterial blood gas analysis to identify the presence of respiratory failure and the need for ventilatory support.3 More recently, clinicians have used ventilatory measurements (e.g., respiratory muscle strength) to support their decision to initiate mechanical ventilation. Interestingly, many of these threshold measurements actually reflect criteria clinicians use to determine when to wean a patient from ventilation.
Decisions made in the acute care setting must be supported by evidence-based criteria. The evidence should clearly demonstrates that a particular intervention is beneficial and is associated with good outcomes, such as improved quality of life, reduced length of stay, or a lower mortality rate.3 This chapter provides information to help clinicians recognize the signs of respiratory distress and respiratory failure. Specific pathologies and methods used to identify the need for an artificial airway and ventilatory support are discussed. Noninvasive positive-pressure ventilation (NIV), an important alternative to the invasive positive-pressure ventilation, is also reviewed. Five patient cases are presented to demonstrate how clinicians can apply various criteria to patients with respiratory failure.
Acute Respiratory Failure
The primary purpose of ventilation is to maintain homeostasis. Mechanical ventilation is indicated when a person cannot achieve an appropriate level of ventilation to maintain adequate gas exchange and acid-base balance. Box 4-1 lists the physiological and clinical objectives of mechanical ventilation.4
Recognizing the Patient in Distress
Left untreated, acute respiratory failure can lead to coma and eventually death. Early recognition of impending respiratory failure can significantly improve the outcomes for these patients. A number of simple and direct observations can be used to identify when a patient is experiencing respiratory distress and guide the selection of an appropriate therapeutic strategy.
The initial assessment of the patient in respiratory distress should focus on several physical findings. First, determine the patient’s level of consciousness. Is the patient awake or asleep? If the patient is asleep or unconscious, can the patient be awakened, and if so, to what extent? Second, assess the appearance and texture of the patient’s skin? Do the nail beds or lips show evidence of cyanosis? Is the patient pale and diaphoretic (sweating)? Third, evaluate the patient’s vital signs (e.g., respiratory rate, heart rate, blood pressure, body temperature, and oxygenation status).
The sudden onset of dyspnea is typically accompanied by physical signs of distress (Fig. 4-1). For example, patients in distress appear anxious, with eyes wide open, the forehead furrowed, and the nostrils flared. These patients may be diaphoretic and flushed. They also may try to sit upright or, if seated, lean forward with their elbows resting on a bedside table or their knees. If they are in respiratory or cardiac distress, they may be ashen, pale, or cyanotic and using their accessory muscles of respiration (e.g., the sternocleidomastoid, scalene, and trapezius muscles). In severe respiratory distress, the intercostal spaces and the supraclavicular notch may appear indented (retracted) during active inspiration. The patient may complain of not getting enough air. Paradoxical or abnormal movement of the thorax and abdomen may be noted, and abnormal breath sounds may be heard on auscultation. Tachycardia, arrhythmias, and hypotension also are common findings.5 Pulse oximetry is a quick and cost-effective method of assessing arterial oxygen saturation and pulse rate (see Chapter 10). (NOTE: Anemia and reduced cardiac output can compromise oxygen delivery to the tissues. In such cases, reduced pulse pressures and blood flow may prevent the pulse oximeter from accurately estimating the patient’s actual arterial oxygen saturation and heart rate.)
It is worth mentioning that in some cases, the signs of respiratory distress are the result of the person experiencing a “panic attack.” Respiratory distress in this type of patient can usually be relieved simply by calming the person and questioning him or her about the distress. (The use of both verbal and nonverbal communication with a patient is vital to effective patient assessment.)
Definition of Respiratory Failure
In acute respiratory failure (ARF), respiratory activity is absent or is insufficient to maintain adequate oxygen uptake and carbon dioxide clearance. Clinically, ARF may be defined as the inability to maintain Pao2, Paco2, and pH at acceptable levels. These levels generally are considered to be (1) a PaO2 below the predicted normal range for the patient’s age under ambient (atmospheric) conditions, (2) a Paco2 greater than 50 mm Hg and rising, and (3) a falling pH of 7.25 and lower.1–3
Two forms of ARF have been described: hypoxemic respiratory failure and hypercapnic respiratory failure.6 Hypoxemic respiratory failure is a result of severe ventilation/perfusion () mismatching. It can also occur with diffusion defects, right-to-left shunting, alveolar hypoventilation, aging, and inadequate inspired oxygen. A good working definition of acute hypoxemic respiratory failure is acute life-threatening or vital organ–threatening tissue hypoxia.3 Hypoxemic respiratory failure can be treated with oxygen or in combination with positive end-expiratory pressure (PEEP) or continuous positive airway pressure (CPAP) (see Chapter 13). Mechanical ventilation may also be necessary if hypoxemic respiratory failure occurs along with acute hypercapnic respiratory failure and an increased work of breathing.
Acute hypercapnic respiratory failure, or acute ventilatory failure, occurs when a person cannot achieve adequate ventilation to maintain a normal Paco2. The ventilatory pump consists of the respiratory muscles, thoracic cage, and nerves that are controlled by respiratory centers in the brainstem. Three types of disorders can lead to pump failure (Box 4-2):
Recognizing Hypoxemia and Hypercapnia
As shown in Table 4-1, the clinical signs of hypoxemia and hypercapnia closely resemble the signs seen in patients with respiratory distress (see Fig. 4-1 and Key Point 4-1). Tachycardia and tachypnea are early indicators of hypoxia. In some cases of hypoxemic respiratory failure, the patient’s condition can be treated successfully by administering enriched oxygen mixtures. However, some hypoxemic conditions, such as severe shunting, are refractory to oxygen therapy (i.e., administering enriched oxygen mixtures does not significantly reduce the level of hypoxemia).
TABLE 4-1
Conditions Seen with Hypoxemia and Hypercapnia
In patients with hypercapnic respiratory failure, PaCO2 levels are elevated with accompanying hypoxemia unless the patient is receiving oxygen therapy. Elevation of PaCO2 leads to an increase in cerebral blood flow as a result of dilation of cerebral blood vessels. Severe hypercapnia eventually leads to CO2 narcosis, cerebral depression, coma, and death.
Untreated hypoxemia, hypercapnia, and acidosis can lead to cardiac dysrhythmias, ventricular fibrillation, and even cardiac arrest.7 The potential for these consequences underscores the importance of recognizing that a patient is in acute or impending respiratory failure and the need to initiate therapy in a timely manner. The elements required to achieve a successful outcome are (1) use of supplemental oxygen therapy, (2) maintenance of a patent airway, and (3) continuous monitoring of oxygenation and ventilatory status with pulse oximetry and arterial blood gas (ABG) analysis.
Patient History And Diagnosis
The various types of pathologic conditions that increase the risk of a patient developing respiratory failure were mentioned previously (see Box 4-2). The following is a brief discussion of some of these conditions. Several case studies are presented to illustrate the clinical findings associated with respiratory failure.
Central Nervous System Disorders
Central nervous system (CNS) disorders that decrease respiratory drive, such as depression of the respiratory centers induced by drugs or trauma, can lead to significant reductions in minute ventilation () and alveolar ventilation (
) and, ultimately, to hypercapnia and hypoxemia. In otherwise normal individuals, an increase in Paco2 greater than 70 mm Hg has a CNS depressant effect, which reduces respiratory drive and ventilation. Hypoxia, which accompanies this process, normally acts as a respiratory stimulant (through stimulation of the peripheral chemoreceptors) to increase breathing. However, because the CNS already is compromised, the body’s response to hypoxia is diminished.
Other CNS disorders associated with tumors, stroke, or head trauma can alter the normal pattern of breathing. For example, a head injury might result in cerebral hemorrhage and increased intracranial pressure (ICP). If significant bleeding occurs with these types of injuries, abnormal breathing patterns such as Cheyne-Stokes respirations or Biot respirations may occur. In many cases, cerebral abnormalities can also affect normal reflex responses, such as swallowing. In these cases endotracheal intubation may be required to protect the airway from aspiration or from obstruction by the tongue (Case Study 4-1).
There is considerable debate about whether controlled hyperventilation should be used as a ventilatory technique in patients with a closed head injury. Controlled hyperventilation lowers the PaCO2 and increases the pH, resulting in reduced cerebral perfusion and reduced ICP. It is important to understand that this effect is temporary, lasting only about 24 hours, because the body eventually adapts to the change through renal compensatory mechanisms.8 Although controlled hyperventilation is still used by some clinicians to lower sudden increases in ICP, clinicians must keep in mind that the desire to use this technique for patients with traumatic brain injury is not by itself an indication for intubation and mechanical ventilation.3 Furthermore, patients with traumatic brain injury have a better long-range outcome (3-6 months) when controlled hyperventilation is not used.8
Neuromuscular Disorders
Neuromuscular disorders that can lead to respiratory failure usually are the result of one of the following:
• Problems with transmission of nerve impulses at the neuromuscular junction
• Central nervous system disorders
The onset of respiratory failure can vary considerably depending on the cause of the neuromuscular dysfunction. Drug-induced neuromuscular failure usually has a rapid onset (Case Study 4-2), whereas the onset of respiratory failure in disease states like myasthenia gravis may not occur for days or years, or it might not happen at all. Regardless of the cause, intubation and mechanical ventilation are indicated if respiratory fatigue occurs rapidly in a patient with a neuromuscular disorder and ARF is imminent.9
The maximum inspiratory pressure (MIP) and vital capacity (VC) can be used to assess respiratory muscle strength of patients with neuromuscular disorders. These measurements are noninvasive, relatively easy to obtain, and inexpensive. Respiratory therapists can measure MIP and VC every 2 to 4 hours to monitor changes in respiratory status. Commonly cited threshold values are an MIP of −20 to −30 cm H2O or less (i.e., 0 to −20 cm H2O) and a VC lower than 10 to 15 mL/kg. Note that although these measures are often used, their effectiveness in improving outcomes has not been established (Table 4-2).3 (Techniques for measuring MIP and VC are discussed later in this chapter.)
TABLE 4-2
Indications of Acute Respiratory Failure and the Need for Mechanical Ventilatory Support in Adults
Criteria | Normal Values | Critical Value |
Ventilation* | ||
pH | 7.35-7.45 | <7.25 |
Arterial partial pressure of carbon dioxide (Paco2) (mm Hg) | 35-45 | >55 and rising |
Dead space to tidal volume ratio (VD/VT) | 0.3-0.4 | >0.6 |
Oxygenation† | ||
Arterial partial pressure of oxygen (Pao2) (mm Hg) | 80-100 | <70 (on O2 >0.6) |
Alveolar-to-arterial oxygen difference P(A-a)o2 (mm Hg) | 3-30 | >450 (on O2) |
Ratio of arterial to alveolar PO2 (Pao2/PAo2) | 0.75 | <0.15 |
Pao2/FIo2 | 475 | <200 |
FIo2, fraction of inspired oxygen.
*Indicates the need for ventilatory support.
Determination of baseline ABG values, along with periodic measurement of oxygen saturation by pulse oximetry (Spo2) is also appropriate when caring for patients with neuromuscular problems. Repeating the ABG analysis may be indicated if the patient’s clinical status changes significantly. Furthermore, if the patient’s condition progressively worsens, the practitioner should not wait until an acute situation develops to intervene (Case Study 4-3). Clinicians generally agree that invasive positive-pressure ventilation should be initiated before acute respiratory acidosis develops.3
Increased Work of Breathing
An increase in the WOB can lead to respiratory failure secondary to respiratory muscle fatigue. WOB normally accounts for 1% to 4% of total oxygen consumption at rest.10 In patients experiencing respiratory distress, the WOB can increase to as much as 35% to 40% of total oxygen consumption.11,12 Increased WOB usually is associated with an increased rate or depth of breathing (or both). More work is required to move the same VT when the patient has obstructed airways, a restrictive lung disorder, or both (Case Study 4-4). A patient’s tolerance to maintain the increased WOB is limited by the eventual fatigue of respiratory muscles. Increased WOB can induce hypoventilation, respiratory insufficiency, and eventually respiratory failure.
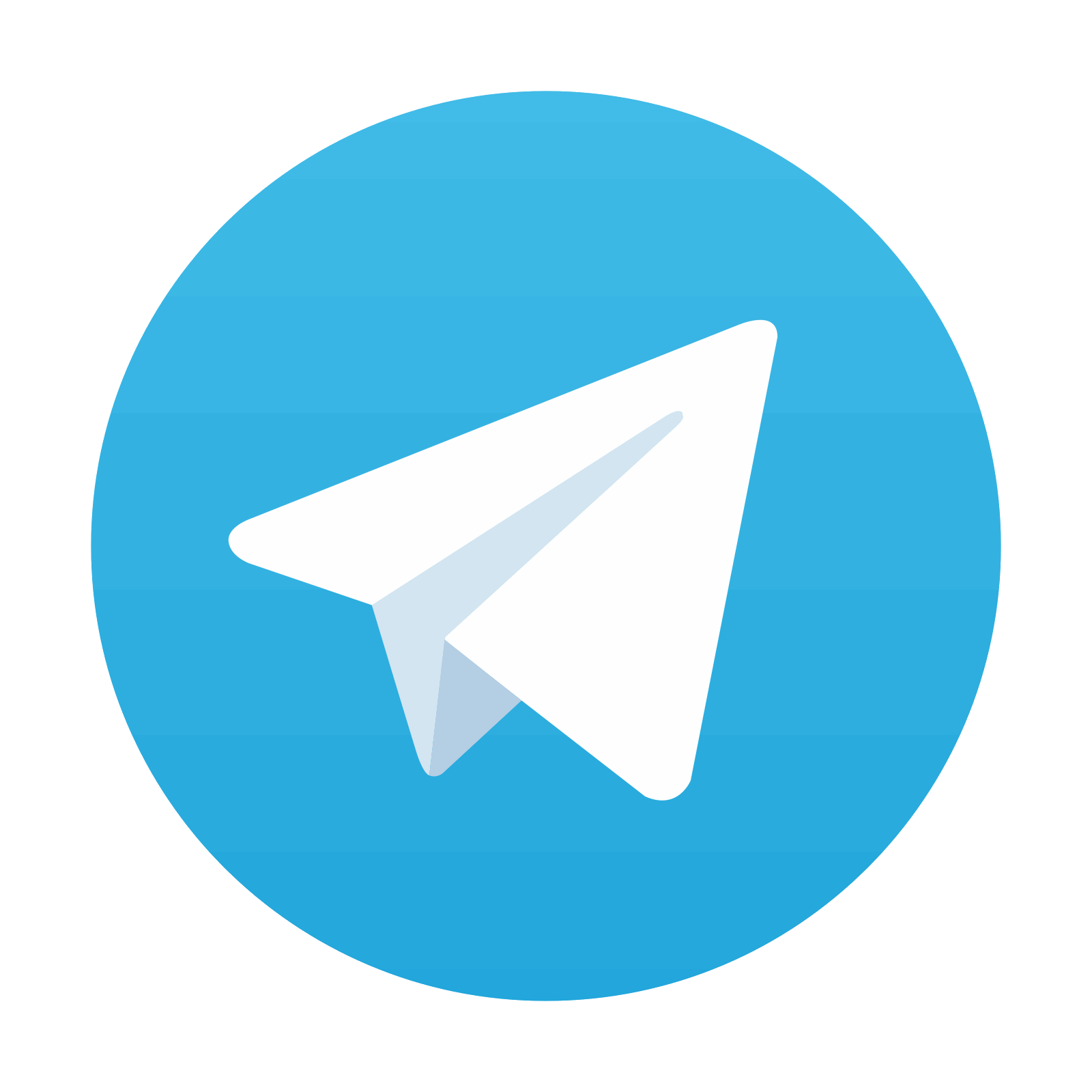
Stay updated, free articles. Join our Telegram channel

Full access? Get Clinical Tree
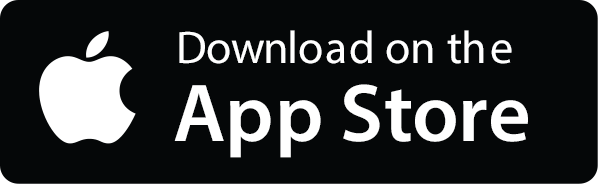
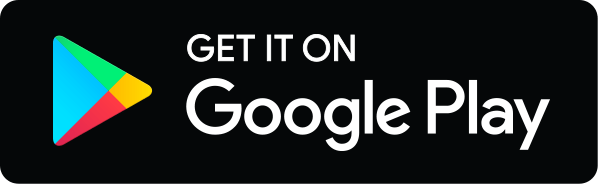