Epidemiology and Mechanisms Linking Sleep-Disordered Breathing and Cardiac Disease: Introduction
Sleep-disordered breathing (SDB) is characterized by repetitive episodes of partial or complete upper airway occlusion occurring during sleep, with resultant intermittent hypoxemia and sleep disruption. Its pathogenesis relates to alteration in upper airway respiratory neuromuscular control and responsiveness during sleep that, in the setting of an anatomically compromised airway with increased critical pressure, is inadequate to maintain nasopharyngeal patency.1 The disorder has a high prevalence, estimated to afflict between 9% and 24% of adults in the general community when using standard polysomnography-based definitions and 2% to 4% when considering polysomnogram findings in addition to daytime somnolence symptoms.2 An explosion of research indicates that SDB is associated with considerable morbidity and mortality,3-5 suggesting that the health burden of SDB is quite substantive.2 Additionally, with the increase in obesity, a major risk factor for SDB, the prevalence of SDB is anticipated to increase, further contributing to cardiovascular disease burden. Although SDB is a treatable condition, at least 75% of severe SDB cases are undiagnosed.6,7 Even in clinical settings such as cardiology or diabetes centers, SDB is often not recognized or addressed as a therapeutic target to improve cardiovascular and metabolic risk factors. In this chapter, risk factors, pathogenesis, and associations of SDB with cardiovascular disease will be reviewed as they relate to adult patients with cardiovascular disease and cardiovascular risk reduction.
Presentation and Pathogenesis
A diagnosis of SDB requires the documentation by polysomnography of an elevated number of apneas and hypopneas per hour of sleep (the apnea-hypopnea index [AHI]), with severity related to the frequency of these events and levels of associated oxyhemoglobin desaturation. In adults, an AHI of <5 is usually considered within the normal range, whereas 15 to 30 events per hour is considered to be of moderate severity and >30 events per hour is considered severe. It is important to note the limitations of this approach. For example, this categorization of disease severity does not take into account length of respiratory events, overall hypoxia burden, or level of accompanying sympathetic nervous system activation.
SDB, with its attendant sleep disruption and fragmentation, is associated with a myriad of signs and symptoms adversely affecting quality of life, including snoring, daytime somnolence, neurocognitive deficits, and irritability. In addition, recurrent upper airway collapse causes a range of physiologic stressors including repetitive intermittent hypoxia, ventilatory overshoot hyperoxia, sympathetic nervous system surges, sleep fragmentation, reduced sleep duration, alterations in intrathoracic pressure, and local airway edema and inflammation. These stressors contribute to a state of enhanced systemic inflammation, oxidative stress, metabolic dysregulation, and increased thrombosis in toto, creating the milieu for adverse cardiovascular sequelae. Substantial morbidity and economic costs are associated with untreated SDB including those related to daytime sleepiness affecting work life/productivity and often resulting in drowsy driving, as well as hypertension and cardiovascular comorbidity.
Risk Factors
The prevalence of SDB varies considerably across the age span. There is some evidence that there is a multimodal distribution of prevalence, with one peak occurring in children ages 4 to 8 years, after which there is a declining prevalence until early adulthood, when the rates of SDB progressively increase.8 Among older individuals, the prevalence of SDB is >20%.9-11 The high prevalence of SDB in older individuals, the population group at highest risk for cardiovascular disease, underscores the importance of recognizing this comorbidity in cardiovascular practice.12
Most population-based data indicate that the prevalence of SDB is two- to three-fold higher in men compared with women.1,13 Men also experience a more rapid progression of the AHI with age than women,8,14 although sex differences decrease after women reach menopause. Sex differences in SDB parallel many sex differences in cardiovascular disease. A male predisposition to SDB is likely multifactorial and reflects the influence of sex hormones on ventilatory control and body fat distribution (with central, or android, obesity associated with pharyngeal fat deposition) and pharyngeal anatomy, with males having generally longer but more collapsible upper airways.1
Differences in the prevalence of SDB among individuals of varied racial and ethnic backgrounds likely reflect genetic factors that influence craniofacial shape, body fat distribution, and physiologic risk factors for SDB, as well as the influence of environmental factors. In the United States, prevalence of SDB among African American children and African American elderly individuals has been reported to be between two- and six-fold higher than European American samples.15-18 One study demonstrated that approximately half of this risk was attributable to neighborhood disadvantage.19 Race-based differences in chemoreceptor and baroreceptor responses also may predispose African Americans to development of SDB.20 Several studies of Asian groups, populations with generally lower rates of obesity than in many Western societies, also have identified a relatively high prevalence of SDB, which is likely attributable to race-based differences in craniofacial morphology.21 For example, one study of a male urban Indian population reported the prevalence of SDB and obstructive sleep apnea-hypopnea syndrome to be 19.5% and 7.5%, respectively, when considering concomitant sleepiness symptoms.22 In an elderly US cohort of men, the highest prevalence of SDB was among the Asian American sample, who, after considering the effects of obesity, had a two-fold higher SDB prevalence compared with whites.10
Approximately 40% to 60% of cases of SDB are attributable to being overweight.1 It is estimated that a 1% increase in body mass index (BMI; kg/m2) is associated with a 3% increase in AHI.23 Excess body weight increases susceptibility to upper airway collapsibility and thus SDB by increased mechanical loading of the upper airway, which causes the upper airway to assume an elliptical, relatively collapsible shape, and by reducing resting lung volume, resulting in a loss of caudal traction on upper airway structures. Additional effects may be related to release of adipokines such as leptin, which can affect ventilatory control.24 Even modest weight loss may improve SDB. Thus, a mainstay of SDB treatment includes weight management with diet and exercise.
Although tobacco smoke exposure may increase upper airway inflammation and snoring, smoking is not a firmly established risk factor for SDB. Alcohol use, however, has been demonstrated to exacerbate SDB, especially during the first 2 hours after ingestion.25,26 In patients with SDB treated with positive airway pressure, alcohol use may increase the required pressure needed to maintain airway patency.25 Thus, patients with SDB need to be cautioned on the use of alcohol and advised to avoid ingestion within several hours of bedtime.
Treatment Approaches
Consistent with other approaches for cardiovascular disease reduction, lifestyle modification to improve activity and diet are important in the management of SDB. Generally conservative but easily implementable treatment approaches also include addressing sleep position, sleep hygiene, and treating nasal problems. Patients in whom SDB occurs either exclusively or predominantly in a supine sleep position should be counseled regarding positional therapy. Avoidance of sleep in a supine position can be accomplished by using a wedge or cushion or by sewing a pocket filled with tennis balls on the back of a pajamas shirt. Individuals with SDB should also be advised to avoid substances that may reduce upper airway muscle tone such as alcohol, sedatives, narcotics, and muscle relaxants. Drowsy driving precautions should be reviewed and documented. Positive airway pressure (PAP) therapy delivered through a nasal or nasal-oral mask stabilizes the airway and thus prevents collapse and is the standard treatment for SDB. An overnight PAP titration study is required to determine the optimal pressure setting that reduces the number of apneas/hypopneas during sleep, improves hypoxia and sleep architecture, and reduces arousals. Adherence with this therapy is highly variable, with average levels ranging from 50% to 80%.27 However, adherence may be positively influenced by patient education reviewing health and quality-of-life benefits of SDB treatment, careful selection of PAP masks that best fit the patient, and supportive management of adverse effects such as nasal congestion and pressure intolerance. Patient perception of symptoms and improvement in sleepiness and daily functioning may be more important in determining patterns of use than physiologic aspects of disease severity.27 Oral appliances, worn during sleep and usually fit by a dentist, may be used to extend the dimensions of the airway and may be effective in select patients, such as those with mild SDB, with positional SDB, or without marked obesity. Upper airway or craniofacial surgical interventions may be an option for SDB treatment; however, they require careful assessment and evaluation including a nasopharyngoscopic examination by an experienced otolaryngologist. Outcomes are often suboptimal, with <50% of patients achieving a 50% reduction in the AHI and reduction to less than critical thresholds.28 Procedures such as those involving injection of the soft palate resulting in stiffening, radiofrequency ablation, laser-assisted uvulopalatoplasty, and palatal implants can improve snoring, but there are minimal data to support their use for SDB. Bariatric surgery, increasingly used to treat multiple obesity-related comorbidities, also may benefit patients with SDB with a BMI >35 kg/m2.
Physiologic Consequences of SDB
Cyclical episodes of hypoxia-reoxygenation occur repetitively in patients with SDB and result in increased inflammation and production of oxygen-derived free radicals with resultant tissue damage.29 Atherogenesis may result as a consequence of hypoxia-reoxygenation–mediated endothelial cell and leukocyte activation and increased expression of adhesion molecules.30-34 The apnea-related recurrent hypoxia-reoxygenation in SDB may be analogous to the well-documented ischemia/reoxygenation injury,35 leading to the formation of oxygen-free radicals through adenosine triphosphate (ATP) depletion and xanthine oxidase activation. This is supported by increased ATP metabolic products such as plasma adenosine and uric acid in SDB.36-38 The severity of oxygen desaturation also is associated with levels of vascular endothelial growth factor, a hypoxia-sensitive glycoprotein that stimulates neoangiogenesis.39 Intermittent hypoxia and reoxygenation also has been demonstrated to result in activation of the proinflammatory transcription factor nuclear factor-κB.40 Other adverse responses to hypoxia include activation of a set of stress genes41 that respond to tissue oxygen deprivation by activating the transcription of genes that influence oxygen delivery,42 such as hypoxia-inducible factor-1.
Endothelial damage may also result from intermittent blood pressure and heart rate surges associated with marked sympathetic activation.43 Augmented sympathetic nervous system activity in SDB is supported by research showing that patients with SDB, compared with controls, have increased muscle sympathetic nervous system activity measured by peroneal microneurography44-46 and elevated levels of urinary norepinephrine levels.46 Levels of urinary norepinephrine have been observed to increase in association with increased AHI levels, increased arousals, and decreased oxygen saturation.47 The specific basis for these sympathetic nervous system surges has not been fully elucidated but may be induced by upper airway closure, hypoxia, hypercarbia, or arousals associated with the respiratory events.48,49 A causal role of SDB in sympathetic activation is supported by studies showing that SDB treatment with PAP therapy lowers urinary norepinephrine46,50,51 and plasma norepinephrine, vanillylmandelic acid, and metanephrine levels.52 Enhanced sympathetic nervous system activity may stimulate the release or expression of inflammatory cytokines.53 Elevated catecholamine levels may undergo auto-oxidation and thus may contribute to conditions such as catecholamine-induced cardiomyopathy.54,55
The repetitive inspiratory efforts during the apneas and hypopneas in SDB lead to exaggerated negative intrathoracic pressure swings (up to –65 mm Hg intrathoracic pressure), causing a cardiac load unique to SDB,56,57 including increased left ventricular transmural pressure, increased afterload and right ventricular venous return, and an abnormal leftward shift of the interventricular septum.58 This leads to increased myocardial oxygen demand, impaired myocardial relaxation, and reduced cardiac output. Progressive increases in intra-atrial pressures lead to atrial myocardial overstretching and dilation, causing cardiac volumetric changes and electrical remodeling that may lead to atrial fibrillation and increased risk of stroke.59,60 Adverse effects on intrathoracic pressure may exacerbate hemodynamic status in heart failure. Several studies have shown that left ventricular diastolic function declines in association with SDB especially with aging.61-63
SDB likely exerts its negative physiologic effects in part due to reduced quantity of sleep and excessive sleep fragmentation as a result of repetitive upper airway obstruction–induced sleep disruption. Reduced sleep duration has been associated with cardiovascular morbidity and all-cause mortality in several epidemiologic surveys.64-68 The proposed pathophysiologic mechanisms likely include increased inflammation as evidenced by research showing elevations in interleukin-6 (IL-6),69 high-sensitivity C-reactive protein (CRP),70 and leukocyte counts71,72 occurring with sleep deprivation. Because sleep is a complex neurophysiologic process, reductions in specific stages of sleep, as may occur in SDB, may be particularly deleterious. For example, reduction in rapid eye movement (REM) sleep may contribute to activation of lipid peroxidation and inhibition of antioxidants,73 and reduction in slow wave sleep, a state highly associated with endocrine functions, may decrease insulin sensitivity, increase cortisol, and increase central body fat deposition.74,75
Intermediate Mechanisms Linking SDB and Cardiovascular Disease
Many of the inflammatory pathways implicated in atherogenesis are also dysregulated in SDB. IL-6, a proinflammatory cytokine secreted by T cells and macrophages, increases in response to experimental intermittent hypercapnic hypoxia76 and is increased with degree of sleep apnea severity.77 Soluble IL-6 receptor, a marker with more expansive physiologic effects than IL-6,78,79 may be particularly sensitive to the more immediate physiologic effects of SDB.80 CRP, an acute-phase reactant produced by the liver and adipocytes and influenced by IL-6 levels, has also been reported to be increased with SDB in individuals without multiple comorbidities and also in children,81,82 although studies of more heterogeneous samples suggest this association may be largely related to obesity.83,84 Two small interventional trials have demonstrated reductions in CRP levels with PAP used to treat SDB consistent with sleep apnea representing a reversible trigger for increased systemic inflammation.85,86 Tumor necrosis factor-α (TNF-α), a cytokine involved in systemic inflammation, has been shown to be significantly elevated in sleep apnea independent of BMI,87,88 and levels are associated with the degree of nocturnal sleep disturbance.88
Oxidative stress, a state of imbalance between increased production of free radicals such as reactive oxygen species (ROS) and reduced antioxidant activity, is considered to play a fundamental role in the pathogenesis of cardiovascular disease.89 Levels of oxidative stress may be assessed using direct measurements of ROS including superoxide, hydrogen peroxide, and the hydroxyl radicals, as well as by measuring enzymes involved with regulation of ROS production such as reduced nicotine adenine dinucleotide phosphate (NADPH) oxidases, superoxide dismutase, and glutathione peroxidase. Indirect measurements of oxidative stress also may be ascertained by measurement of oxidized products of lipids, proteins, or DNA. Malondialdehyde, thiobarbituric acid reactive substances, oxidized low-density lipoprotein (LDL) levels/autoantibodies, and F2-isoprostanes from urinary, plasma, exhaled condensate, or sputum samples have been the most studied measures of oxidative stress in SDB. Measurement of F2-isoprostane is currently considered to be one of the most accurate methods to quantify global lipid peroxidation. Increased levels of overnight urinary 8-isoprostane levels have been reported in SDB, with levels correlated with degree of hypoxemia and with evidence of reduction in these levels following PAP treatment.90 Morning plasma malondialdehyde levels have been reported to be significantly higher in SDB subjects compared with controls.91 Malondialdehyde levels also have been reported to be elevated in the intercostal muscles of individuals with sleep apnea, with elevations persisting even after long-term sleep apnea treatment.92 Inconsistent associations with SDB have been reported when oxidative stress was quantified using measures of thiobarbituric acid reactive substances,93-95 oxidized LDL,95,96 and circulating free nitrotyrosine.97 However, many of these assays are difficult to measure accurately and are prone to artifact, and most studies have suffered from small sample sizes. Neutrophil superoxide generation has been observed to be increased in SDB, and superoxide generation has been reversed with treatment.34 Oxidized DNA, measured by urinary 8-hydroxy-2′-deoxyguanosine levels, was significantly higher in subjects with severe sleep apnea than controls and correlated with degree of intermittent hypoxemia.89 Individuals with sleep apnea also have been reported to have lower total antioxidant capacity, which increased after SDB treatment.98 A microarray study has shown that sleep apnea is associated with increased expression of genes involved in modulation of ROS, including heme oxygenase 1, superoxide dismutase 1 and 2, and catalase, in addition to genes involved in cell growth and proliferation.99 These studies support the notion that oxidative stress associated with sleep apnea may serve as an intermediate mechanism to atherothrombosis.
There is some evidence for a hypercoagulable state in sleep apnea, which also may contribute to the increased prevalence of vascular diseases in this population. Sleep apnea has been associated with increased levels of plasminogen activator inhibitor-1 (PAI-1),100 fibrinogen,101 activated coagulation factors XIIa and VIIa, thrombin/antithrombin III complexes, and soluble P selectin.102 Most studies have identified levels of hypoxemia to correlate with these levels. PAI-1, a molecule that inhibits fibrinolysis by inactivation of tissue plasminogen activator, has been shown to contribute to atherothrombotic events and an increased risk of recurrent myocardial infarction.103-105 PAI-1 levels have been shown to vary with measures of hypoxia106,107 and sympathetic nervous system activation.108 PAI-1 levels have been reported to be elevated in individuals with SDB and to improve with SDB treatment.109 Fibrinogen plays a role in clot formation and has been identified as a predictor of coronary artery disease.110 Several small studies have identified an association between fibrinogen and SDB111,112; however, potential confounders such as obesity were not considered in these studies. D-dimer is a cross-linked fibrin degradation fragment that is highly sensitive in the detection of venous thromboembolic disease and may identify abnormalities of thrombosis in both the venous and arterial systems.113-115 The literature regarding an association between SDB and D-dimer is inconsistent,100,109,116 and it is unclear whether this marker varies with SDB.
Several observational studies have demonstrated associations between sleep apnea and insulin resistance that are independent of obesity.117 An uncontrolled interventional study demonstrated improved insulin sensitivity in patients with moderate to severe sleep apnea with treatment, with the best responses occurring in those with a BMI <30 kg/m2.118 Using frequently sampled intravenous glucose tolerance testing, SDB was found to be associated with impairments in insulin sensitivity, glucose effectiveness, and pancreatic β-cell function.119 Glucose intolerance and insulin resistance in SDB may be mediated by upregulation of inflammatory cytokines.117 Intermittent hypoxia associated with SDB may lead to glucose intolerance and insulin resistance by promoting the release of proinflammatory cytokines, such as IL-6 and TNF-α. IL-6 levels have been correlated with indices of insulin resistance, and higher levels have been associated with an increased risk of type 2 diabetes mellitus.120-122 Increased levels of TNF-α also likely contribute to the development of insulin resistance.123-126 Two clinic-based studies have shown that plasma levels of IL-6 and TNF-α are higher in patients with SDB than in control subjects.77,127 Animal studies showing an increase in insulin levels with exposure to hypoxemic conditions provide further evidence implicating SDB in the pathogenesis of metabolic dysfunction.128-130
Increased sympathetic activity associated with SDB also may affect glucose homeostasis by increasing glycogen breakdown and gluconeogenesis.45,131,132 Further predisposition toward metabolic dysfunction may occur through effects of SDB on the hypothalamic-pituitary-adrenal axis. Experimental partial or total sleep deprivation has been shown to increase levels of plasma cortisol on the following evening at a time when the circadian rhythm of the hypothalamic-pituitary-adrenal axis is at its nadir.133 The increase in evening cortisol can result in pronounced increases in serum glucose levels and insulin concentrations and increased insulin secretion.134
Endothelial dysfunction may occur in SDB as a result of systemic inflammation, oxidative stress, and sympathetic nervous system activation. Individuals with SDB have impaired resistance vessel (small vessel) endothelium-dependent dilation, a measure that is associated with the pathogenesis of hypertension and heart failure.135 A controlled study in normotensive men with SDB demonstrated that endothelium-dependent, flow-mediated dilation significantly increased in men who were compliant with 6 months of SDB treatment.136 Two studies suggested that the effects of SDB on endothelial function may be stronger in women than men.137,138
A number of the SDB exposures described earlier, including effects on systemic blood pressure, may be expected to adversely influence myocardial structure and function.139,140 Left ventricular hypertrophy and diastolic dysfunction in SDB may occur even in the absence of hypertension and may possibly be mediated by responses to cytokines, endothelin, and/or growth factors.141 As shown in Fig. 74–1, physiologic mechanisms also include repetitive exposure to changes in intrathoracic pressure and increased pre- and afterload, increased myocardial oxygen demand, reduction of myocardial oxygen supply, catecholamine-induced myocyte injury,142 or direct influences of hypoxia on cardiac contractility.143
Figure 74–1.
Pathophysiology of central sleep apnea in heart failure (HF). HF leads to increased left ventricular (LV) filling pressure. The resulting pulmonary congestion activates lung vagal irritant receptors, which stimulate hyperventilation and hypocapnia. Superimposed arousals cause further abrupt increases in ventilation, triggering a central apnea. Central sleep apneas are sustained by recurrent arousals resulting from apnea-induced hypoxia and the increased effort to breathe during the ventilatory phase because of pulmonary congestion and reduced lung compliance. Although central apneas have a different pathophysiology than obstructive apneas and are not associated with the generation of exaggerated negative intrathoracic pressure, they both increase sympathetic nervous system activity (SNA). The consequent increases in blood pressure (BP) and heart rate (HR) increase myocardial oxygen (O2) demand in the face of reduced supply. This chain of events contributes to a pathophysiologic vicious cycle. PaCO2, arterial partial pressure of carbon dioxide; PaO2, arterial partial pressure of oxygen. Reproduced with permission from Bradley TD, Floras JS. Sleep apnea and heart failure: part II: central sleep apnea. Circulation. 2003;107(13):1822-1826.
Animal models have shown the evolution of hypertension, left ventricular hypertrophy, and reduced left ventricular ejection fraction to occur as a result of experimentally manipulated long-term SDB exposure.144,145 In humans, a progressive increase in left ventricular mass index with AHI level, independent of BMI, has been reported in the Sleep Heart Health Study, an observational study investigating cardiovascular outcomes in SDB.146 More severe SDB, as defined by higher AHI and more hypoxemia, was associated with larger left ventricular systolic dimensions, lower left ventricular ejection fraction, and a trend toward lower left ventricular fractional shortening. Another report identified early diastolic velocity, an index of left ventricular diastolic dysfunction, to be associated with severity of SDB independently of BMI, diabetes mellitus, and hypertension.147 After considering the effects of obesity and hypertension, SDB also has been associated with functional and structural left atrial changes, including maximal left atrial volume and higher active atrial ejection fraction; these changes also were correlated with degree of impaired diastolic function as assessed by a minute tissue Doppler analysis.60 SDB may more adversely affect myocardial function in patients with underlying coronary artery disease than in those without coronary artery disease.148 A small randomized controlled trial of sleep apnea treatment in heart failure reported treatment-associated reductions in blood pressure and heart rate as well as a 9% improvement in the left ventricular ejection fraction,149 suggesting that treatment of SDB may lead to improved cardiac function.
SDB-Related Cardiovascular Consequences
SDB-related physiologic perturbations leading to the development of systemic hypertension include repetitive upper airway obstruction–associated sympathetic nervous system activation, intermittent negative intrathoracic pressure swings, sleep deprivation, and recurrent episodes of hypoxemia and hypercapnia resulting in arterial chemoreceptor activation and increased sympathetic activity, which individually or interactively may result in acute blood pressure increases and additionally contribute to sustained sympathetic activation and chronically elevated blood pressure even during nonsleep periods. Animal studies implicate a central role of hypoxemia occurring with upper airway obstruction,144,150 whereas a human study suggests a critical role of upper airway–associated arousal in the pathogenesis of chronic hypertension.151 Increases in blood pressure and heart rate occur approximately 5 to 7 seconds after the termination of an apnea or hypopnea, coincident with arousal, peak ventilation, and nadir oxygen saturation.152,153 The hemodynamic profile of blood pressure patterns in SDB is characterized by a nondipping blood pressure pattern,150 a profile also associated with an increase in cardiovascular disease.154
Abundant cross-sectional data support an association between SDB and hypertension even independent of obesity,11,155-162 including data from large cohorts such as the Wisconsin Sleep Cohort, a Pennsylvania-based cohort, and the Sleep Heart Health Study. In a cross-sectional analysis of 709 Wisconsin Sleep Cohort participants, an independent association between blood pressure and SDB (AHI ≥5) was observed.11 In a Pennsylvania-based study involving 1741 participants, a dose-response pattern was observed for SDB and hypertension (ie, the odds ratios [ORs] for hypertension were 6.8, 2.3, and 1.6 for moderate or severe SDB, mild SDB, and snoring, respectively). Additionally, this association was strongest among individuals who were young and of normal weight.163 In a cross-sectional analysis of data from 6132 participants in the Sleep Heart Health Study, mean systolic and diastolic blood pressure and prevalence of hypertension increased significantly with increasing severity of SDB, measured by the AHI and percentage of sleep time spent below 90% oxygen saturation, and a 40% to 50% increased adjusted odds of hypertension was observed for those with an AHI ≥30 or upper quartile of hypoxemia.162 In a sample of 2677 adults referred to a sleep clinic with suspected SDB, the mean blood pressure level and prevalence of hypertension were reported to increase linearly with severity of SDB; each additional apneic event per hour of sleep was estimated to increase the odds of hypertension by approximately 1%, and each 10% decrease in nocturnal oxygen saturation increased the odds by 13%.160 Overall, these studies demonstrate associations between SDB and hypertension even with mild levels of SDB, show dose-response relationships between hypertension and increasing SDB severity, and demonstrate persistence of statistical significance of these associations even after taking into account obesity measures.
A causal association between SDB and hypertension is supported by longitudinal data from the Wisconsin Sleep Cohort Study, which showed significant dose-response relationships, such that relative to the reference category (baseline AHI = 0), the ORs for the presence of hypertension at the 4-year follow-up were 1.42 for an AHI of 0.1 to 4.9, 2.03 for an AHI of 5.0 to 14.9, and 2.89 for an AHI of ≥15.0.164 Recent findings from the Sleep Heart Health Study also demonstrated a significant longitudinal relationship between SDB and the development of hypertension among individuals who were normotensive at baseline examination; however, this relationship was attenuated to borderline significance after taking into account obesity.165 The estimated effect in this study, however, was similar to the cross-sectional findings, and the reduction in power due to restricting the sample to those who were normotensive at baseline may have accounted for these marginal findings.166
Several randomized controlled trials have been performed to assess the effect of SDB treatment on blood pressure measures (Table 74–1).167-175 These studies used various types of placebos including sham continuous positive airway pressure (CPAP),169 low CPAP,167,170,171 and oral placebo. A randomized placebo-controlled trial involving 68 participants with moderate to severe SDB not on antihypertensive medication reported a small statistically significant reduction (1.4 mm Hg) in 24-hour diastolic blood pressure with the greatest decrease occurring between 2:00 am and 9:59 am. The observed decrease in 24-hour diastolic blood pressure was greater in the group with CPAP use ≥3.5 hours per night (1.9 mm Hg) and those with >20 4% desaturations per hour (5 mm Hg). A significant reduction in systolic pressure of 4 mm Hg was also reported in the latter group. Desaturation frequency was identified as the best predictor of diastolic blood pressure decrease with CPAP in this study.168 Another randomized trial (n = 60) involving subjects with moderate to severe SDB demonstrated a larger reduction in blood pressure (reduction of mean, diastolic, and systolic pressures of ∼10 mm Hg).170 Of note, this study included the highest percentage of participants with hypertension of all the randomized controlled studies, suggesting that hypertensive individuals may derive more benefit from SDB treatment than normotensive individuals.170 This finding was further corroborated by a secondary analysis of another randomized trial evaluating SDB treatment effects on blood pressure.169
Citation | Sample Size (n, % of men) | Source of Recruitment | Intervention/Placebo | Sleep Apnea Definition | Outcome and Monitoring Type | Conclusions and Limitations |
---|---|---|---|---|---|---|
Studies supporting a relationship between sleep apnea treatment and blood pressure improvement | ||||||
Becker et al170 (2003) | n = 32 (91%) | Sleep center | CPAP/sham CPAP | Mean AHI = 64 | Ambulatory blood pressure monitoring (19 h) | A significant reduction in blood pressure was noted (mean decrease of 9.9 mm Hg) in subjects treated with therapeutic CPAP compared with subtherapeutic CPAP. These subjects had severe sleep apnea, and the majority were on antihypertensives. |
Pepperell et al169 (2002) | n = 118 (100%) | Sleep center | CPAP/sham CPAP | Mean 4% oxygen saturation dip rate = 37 | Ambulatory blood pressure monitoring (24 h) | Demonstrated statistically significant 3.3-mm Hg decrease in blood pressure in subjects with severe sleep apnea (defined by hypoxia) treated with CPAP compared with those receiving subtherapeutic treatment. Subgroup analyses showed greatest decrease in blood pressure in those with severe sleep apnea or on antihypertensives. |
Faccenda et al168 (2001) | n = 68 (80.9%) | Sleep center | CPAP/oral placebo | Median AHI = 35 | 48-h ambulatory blood pressure during the first 2 d on CPAP or placebo | A 1.4-mm Hg reduction in diastolic blood pressure with CPAP relative to placebo was noted, with the greatest decrease occurring between 2:00 am and 9:59 am. This decrease in diastolic blood pressure was slightly greater in those compliant with CPAP (1.9-mm Hg reduction) and those with >20 4% desaturations per hour (5-mm Hg reduction). Systolic pressure was reduced by 4 mm Hg in those with >20 4% desaturations per hour. Desaturation frequency was the best predictor of diastolic blood pressure decrease with CPAP. |
Dimsdale et al167 (2000) | n = 39 (80%) | Community sample | CPAP/sham CPAP | Mean AHI = 48 | Ambulatory blood pressure monitoring | Demonstrated a 5-mm Hg nocturnal blood pressure decrease in the group titrated with CPAP compared to the sham CPAP group over 1 wk of follow-up. |
Engleman et al172 (1996) | n = 13 (85%) | Sleep center | CPAP/oral placebo | Mean AHI = 60 | Ambulatory blood pressure monitoring (24 h) | Systolic, diastolic, and mean arterial blood pressures for 24-h, daytime, and nighttime periods were not significantly different on placebo compared with CPAP administered over a 3-wk period. However, those with no overnight decrease in blood pressure on placebo (“nondippers”) had improvement in daytime mean arterial blood pressure on CPAP (4-mm Hg reduction) compared with placebo. |
Studies that do not support a relationship between sleep apnea treatment and blood pressure improvement | ||||||
Campos-Rodriguez et al173 (2006) | n = 55 (64%) | Sleep center | CPAP/sham CPAP | Mean AHI = 60 | Ambulatory blood pressure monitoring (24 h) | CPAP did not reduce blood pressure in patients with sleep apnea and hypertension who were treated with antihypertensive medication compared with the placebo group. |
Robinson et al174 (2006) | n = 35 (89%) | Sleep center | CPAP/sham CPAP | Mean 4% oxygen saturation dip rate = 28 | Ambulatory blood pressure monitoring (24 h) | There was no significant difference in mean blood pressure on therapeutic CPAP versus subtherapeutic CPAP, with a reduction of 0.7 mm Hg. |
Barnes et al171 (2002) | n = 28 (83%) | Sleep center (2 Australian centers) | CPAP/oral placebo | Mean AHI = 13 | Ambulatory blood pressure monitoring (24 h) | No difference noted in 24-h blood pressure in those treated with CPAP for 8 wk versus sham CPAP in those with mild sleep apnea. |
Barbe et al175 (2001) | n = 54 (89%) | Sleep center (multicenter, 6 teaching hospitals in Spain) | CPAP/sham CPAP | Mean AHI = 54 (without daytime sleepiness) | Ambulatory blood pressure monitoring (24 h) | Patients with severe sleep apnea treated with CPAP for 6 wk did not have a significant change in blood pressure compared with those treated with subtherapeutic CPAP for 6 wk. |
SDB may increase resistance to antihypertensive medications as supported by findings from a study demonstrating a high prevalence of SDB in individuals with refractory hypertension and marked reduction in blood pressure subsequent to SDB treatment with CPAP.176 A study involving participants with mild SDB, however, failed to show significant improvements in blood pressure in this setting.171 A meta-analysis of these randomized controlled trial data showed that overall, among individuals with SDB, CPAP reduces 24-hour ambulatory mean blood pressure, with greater treatment-related reductions among those with a more severe degree of SDB and a better effective nightly use of the CPAP device.177
There is likely a bidirectional relationship between congestive heart failure and SDB, particularly central sleep apnea. Significant risk factors identified for central sleep apnea in heart failure include male sex (OR = 3.50), atrial fibrillation (OR = 4.13), age >60 years (OR = 2.37), and hypocapnia (partial pressure of carbon dioxide [PCO2] <38 mm Hg during wakefulness; OR = 4.33).178 Heart failure may contribute to SDB development by precipitating periodic breathing and upper airway neuromotor instability as well as through effects that include accumulation of fluid or edema of the upper airway, thereby enhancing collapsibility. Patients with congestive heart failure are at increased susceptibility for periodic breathing, most specifically recognized as Cheyne-Stokes respiration, due to a combination of factors including hyperventilation, enhanced chemosensitivity to carbon dioxide, low cardiac output, and prolonged circulation time.179-181 This breathing disturbance reflects abnormal oscillations of the feedback loop controlling respiration, leading to waxing and waning of breathing patterns.182,183 Chronic hyperventilation is due to stimulation of pulmonary vagal irritant receptors by pulmonary vascular congestion,181,184,185 which is exacerbated in the supine position with increased venous return186 and enhanced peripheral and central chemosensitivity.187,188 During sleep, central apneas are induced when ventilation causes the PCO2 level to fall below the threshold level (apneic threshold) that is required to stimulate breathing, decreasing the central drive to respiratory muscles.186 Sleep-related reductions in pulmonary function residual capacity may contribute to periodic breathing by reducing oxygen stores.189-191 Obstructive apneas and hypopneas can occur during nadirs of neuromuscular output when the relative innervations of the upper airway muscles are insufficient to maintain airway patency.
Conversely, SDB may adversely affect cardiac function through mechanisms described earlier (see Cardiac Morphology section), including adverse effects of chronic hypertension, nondipping blood pressure, atherosclerosis and ischemic injury, hypoxemia- and catecholamine-related myocyte injury, and cardiac remodeling. Brain natriuretic peptide (BNP) has been associated with the level of overnight hypoxemia (the sleep time spent with oxygen saturation <90%) in patients with heart failure; each 10% increase in duration of hypoxemia increased BNP by 9.6%, suggesting that hypoxemia may be an important factor that underlies the impact of sleep abnormalities on hemodynamic stress in patients with systolic heart failure.192
SDB has been reported to be common in patients with heart failure due to systolic dysfunction. Of 450 patients with a mean left ventricular ejection fraction of 27%, 38% were observed to have obstructive sleep apnea, and 72% had central sleep apnea,178 which is much higher than the reported 5% to 10% prevalence of SDB in the healthy middle-aged population.2 Another study of 81 patients with systolic dysfunction found a high prevalence of central sleep apnea, occurring in 40% of the sample, with another 11% having obstructive sleep apnea.193 Cross-sectional results from the Sleep Heart Health Study demonstrated that modest levels of SDB (ie, AHI ≥11) were associated with 2.38 higher odds of self-reported heart failure independent of other risk factors.194
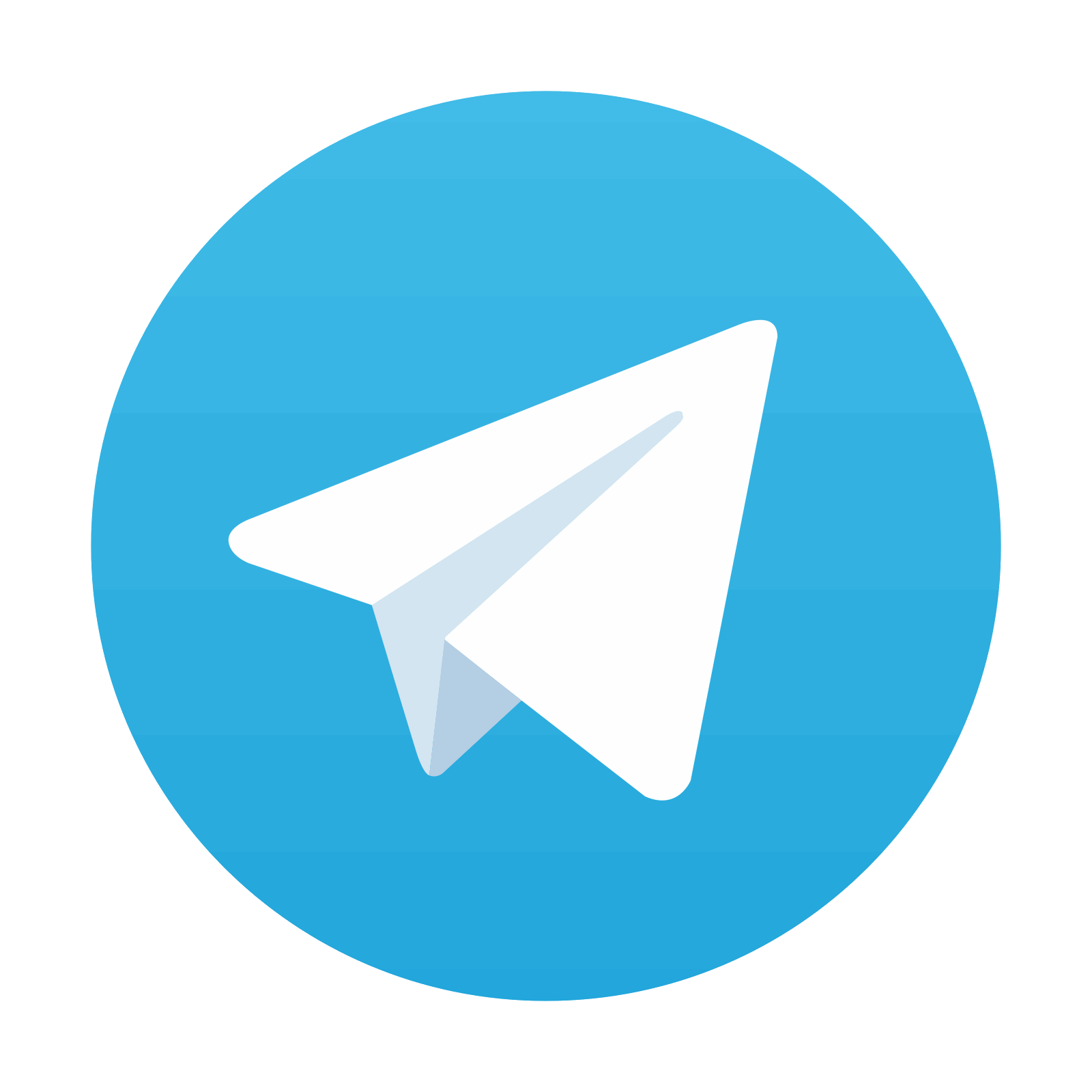
Stay updated, free articles. Join our Telegram channel

Full access? Get Clinical Tree
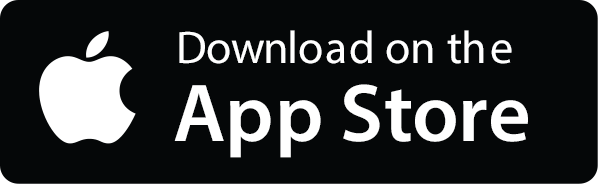
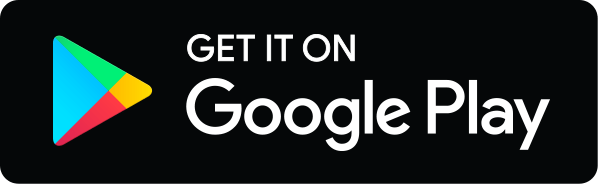
