Epidemiology
Globally, diabetes mellitus is a major threat to human health. The number of people with diabetes has increased alarmingly since 1985, and the rate of new cases is escalating. In 1985, an estimated 30 million people worldwide had diabetes; by 2003, it was estimated that approximately 194 million people had diabetes, and this figure is expected to increase to almost 350 million by 2025.1
The prevalence of diabetes is higher in developed countries than in developing countries, but the developing world will be hit the hardest by the diabetes epidemic in the future. Increased urbanization, westernization, and economic growth in developing countries have already contributed to a substantial increase in diabetes. Although diabetes is most common among the elderly in many populations, prevalence rates are increasing among young populations in the developing world.
Diabetes mellitus, whether type 1 or type 2, is a very strong risk factor for the development of coronary heart disease (CHD) and stroke2 (Table 91–1). Eighty percent of all deaths among diabetic patients are a result of atherosclerosis, compared with approximately 30% among nondiabetic persons. A large National Institutes of Health (NIH) cohort study revealed that heart disease mortality in the general US population is declining at a much greater rate than it is in diabetic subjects. In fact, diabetic women suffered an increase in heart disease mortality over that period.2 Among all hospitalizations for diabetic complications, >75% are a consequence of atherosclerosis. An increase in the prevalence of diabetes has been noted, which, in part, can be attributed to the aging of the population and an increase in the rate of obesity and sedentary lifestyle in the United States.
Cigarette smoking |
Assess pack-years |
Blood pressure |
Duration (if known); current and previous medications; assess presence of orthostatic hypertension |
Serum lipids and lipoproteins |
Dietary habits, alcohol intake, amount of exercise and whether aerobic |
Family history of dyslipidemia, eruptive xanthoma, lipemia, retinalis, xanthelasma; thyroid function tests |
LDL, HDL, cholesterol, fasting triglycerides |
Spot albumin-to-creatinine ratio (in micro- and macroalbuminuria) |
Serum creatinine |
Do not rely on dipstick protein because negative results may reflect lack of sensitivity of test |
Glycemic status |
Duration of diabetes; family history of diabetes; vascular, renal, and retinal complications |
Laboratory: FPG, hemoglobin A1c every 3 mo; diagnosis: FPG >126 × 2, hemoglobin A1c > 6.5%,3 impaired fasting glucose 110-126 × 2; when in doubt, have patient undergo 2-h oral glucose tolerance test |
Diabetes accelerates the natural course of atherosclerosis in all groups of patients and involves a greater number of coronary vessels with more diffuse atherosclerotic lesions (Fig. 91–1). Cardiac catheterizations in diabetic patients have shown significantly more severe proximal and distal CHD. In addition, plaque ulceration and thrombosis have been found to be significantly higher in diabetic patients. Cardiovascular complications include CHD, peripheral artery disease, nephropathy, retinopathy, cardiomyopathy, and possible neuropathy (involvement of vasa vasorum). These observations underscore the heightened risks of a diabetic patient to develop vascular disease and compel the physician to monitor and correct all of the risk factors (see Table 91–1). By understanding the mechanisms underlying all these risks, physicians will be poised to prevent them.
Figure 91–1.
Schematic of staging (phases and lesion morphology of the progression of coronary atherosclerosis according to the gross pathologic and clinical findings). See text for more details. END.ADH.MOL., endothelial adhesion molecule; HDL, high-density lipoprotein; LDL, low-density lipoprotein; Lp(a), lipoprotein a; MCP-1, monocyte chemoattractant protein-1; M-CSF, macrophage colony-stimulating factor; MM-LDL, minimally modified low-density lipoprotein; NF-kB, nuclear factor-κB; Numbers 1-5, stages of progression of coronary atherosclerosis; OX-LDL, oxidized low-density lipoprotein.
The metabolic syndrome is considered to be an aggregation of various established cardiovascular risk factors. Multiple attempts have been made to define the metabolic syndrome encompassing what each investigator deems the relevant clusters. The most widely used definitions come from the National Cholesterol Education Program Adult Treatment Panel (NCEP-ATP) III and the World Health Organization (WHO).4,5 The NCEP-ATP III definition requires any three of the following five criteria: a triglyceride level ≥150 mg/dL or receiving a triglyceride-lowering agent; high-density lipoprotein (HDL) cholesterol <40 mg/dL in men and <50 mg/dL in women or receiving an HDL-raising agent; blood pressure ≥130/≥85 mm Hg or receiving antihypertensive therapy; fasting glucose ≥100 mg/dL; and a waist circumference >40 inches in men and >35 inches in women, with some variations for different ethnicities. The WHO definition requires the identification of insulin resistance by one of the following: type 2 diabetes; impaired fasting glucose, impaired glucose tolerance, or, for those with normal fasting glucose levels <110 mg/dL, glucose uptake below the lowest quartile for background populations under investigation under hyperinsulinemic euglycemic conditions; plus any two of the following: antihypertensive medication and/or high blood pressure (≥140 mm Hg systolic or ≥90 mm Hg diastolic), plasma triglycerides ≥150 mg/dL, HDL cholesterol <35 mg/dL in men or <39 mg/dL in women, body mass index >30 kg/m2 and/or waist-to-hip ratio >0.9 in men or >0.85 in women, and urinary albumin excretion rate ≥20 μg/min or albumin-to-creatinine ratio ≥30 mg/g. Other definitions from the International Diabetes Federation and American Association of Clinical Endocrinologists have also been proposed.6,7 Isomaa et al8 showed, using WHO criteria, a cardiovascular mortality of 12% in patients with metabolic syndrome versus 2% in patients without metabolic syndrome over a 6.9-year follow-up. In the National Health and Nutritional Examination Surveys (NHANES) III study, metabolic syndrome was identified in 5% of normal weight, 22% of overweight, and 60% of obese individuals.9 In the Framingham study, an increase in weight of 2.25 kg (5 lb) over 16 years was associated with a 21% to 45% increase in risk of developing metabolic syndrome. A large waist circumference identifies 46% of individuals who are at risk of developing metabolic syndrome in the next 5 years. The 10-year risk of a cardiovascular event (per Framingham data) in men with metabolic syndrome ranged from 10% to 20% and was 10% in women. The diagnosis of metabolic syndrome was associated with a significant three-fold increase in risk of CHD and stroke and a highly significant increase in cardiovascular mortality.10
Five landmark studies11-15 have established beyond any reasonable doubt the strong relationship between metabolic syndrome and cardiovascular disease. The Strong Heart Study11 of 890 Pima Indians showed that 144 (16.2%) developed type 2 diabetes over 4 years and that metabolic syndrome increased the risk for type 2 diabetes by 2.1-fold (NCEP-ATP definition) and 3.6-fold (WHO definition). The Beaver Dam Study12 involved 4423 white patients with metabolic syndrome but not type 2 diabetes and demonstrated a 9- to 34-fold increase in type 2 diabetes at 5-year follow-up. The West of Scotland Coronary Prevention Study (WOSCOPS)13 evaluated 5974 patients without type 2 diabetes. At the 5-year follow-up, patients with four to five metabolic syndrome features had a 24.5-fold increased risk of type 2 diabetes, and their CHD risk was 3.7-fold higher. The Finland study14 of 1200 patients and an 11-year follow-up demonstrated that patients with metabolic syndrome had a 4.2-fold increased relative risk of coronary artery disease and a two-fold increase in all-cause mortality. Finally, the Air Force/Texas Coronary Atherosclerosis Prevention Study (AFCAPS/TexCAPS)15 study confirmed a 1.5-fold increase in major adverse cardiovascular events in metabolic syndrome patients.
Complications of Diabetes
Nephropathy occurs in 40% of patients with type 1 and type 2 diabetes. Risk factors include poor glycemic control, hypertension, and ethnicity (eg, blacks, Mexicans, Pima Indians).16Table 91–2 summarizes the key points for the assessment of renal status in a diabetic patient. The earliest clinical finding of diabetic kidney disease is microalbuminuria, which may occur at a time when renal histology is essentially normal (Fig. 91–2). The Diabetes Control and Complications Trial (DCCT) and the United Kingdom Prospective Diabetes Study (UKPDS) showed that the development and progression of microalbuminuria can be prevented through strict glycemic control.
Urine albumin and protein |
Yearly screen for microalbumin in type 1 and type 2 diabetes; microalbumin-to-creatinine ratio collected in a spot urine, ideally first morning urine specimen (normal <30 mg/g creatinine); must rule out other diseases that cause proteinuria |
If urine albumin-to-creatinine ratio is >300 mg/g in first morning specimen, macroalbuminuria is present and is usually not reversible with ACE inhibitors; nephrology consult |
Nephrotic syndrome: urine protein >3 g/d; nephrology consult |
Other reasons to consult nephrologists are diabetic patients with increasing creatinine from 1.4 to >2.0 mg/dL, elevated creatinine, and symptoms of uremia and microalbuminuria not responding to ACE inhibitor |
Urinalysis |
Red blood cells, pyuria, and casts require nephrology consult |
Blood pressure evaluation |
If hypertension is present, exclude secondary causes, including with advancing renal insufficiency |
Treatment with an ACE inhibitor is preferred first choice even in African Americans (except if precluded by hyperkalemia or other complications) |
Blood urea nitrogen, serum creatinine, and glomerular filtration rate |
Yearly creatinine clearance should be obtained with 24-h urine collection and serum creatinine; most accurate way to estimate kidney function without using a radioisotope |
Figure 91–2.
Coronary artery disease risk in patients with diabetes mellitus. A subset of genetically predisposed patients develops diabetic nephropathy. In these patients, the risk for coronary artery disease (CAD) increases dramatically. AGE, advanced glycosylation end-product; GFR, glomerular filtration rate; FVII, factor VII; HDL, high-density lipoprotein; LDL, low-density lipoprotein; Lp(a), lipoprotein(a); PAI, plasminogen activator inhibitor; VLDL, very-low-density lipoprotein; vWF, von Willebrand factor. Reprinted from Aronson D, Bloomgarden Z, Rayfield EJ. Potential mechanisms promoting restenosis in diabetes mellitus. J Am Coll Cardiol. 1996;27:528-535, Copyright (1996), with permission from Elsevier.
The UKPDS of type 2 diabetics and studies of patients with type 1 diabetes using captopril have shown that control of hypertension slows the progression of nephropathy. The blood pressure should be maintained at <130/85 mm Hg, and angiotensin-converting enzyme (ACE) inhibitors are the preferred antihypertensive agents. The UKPDS, however, showed no difference in blood pressure control with captopril versus atenolol. The benefit of antihypertensive therapy with an ACE inhibitor in type 1 diabetes can be shown early in the course of disease, when microalbuminuria is the only abnormality.17
There is insufficient evidence to recommend ACE inhibitors in normotensive patients without microalbuminuria. Nonetheless, physicians should still recommend screening on at least a yearly basis because the risk-to-benefit ratio of diagnosing microalbuminuria justifies treatment with an ACE inhibitor, if not for renal disease alone, then for reducing the incidence of myocardial infarction (MI).
Patients on ACE inhibitors should be monitored for potassium because they may develop hyperkalemia in the presence of a type 4 renal tubular acidosis. Sodium restriction reduces hypertension and therefore is advised. Dietary protein should be adjusted to 0.8 g/kg/d to decrease intraglomerular pressure.
More recently, clinical trials evaluating angiotensin receptor blockers (ARBs), including losartan and irbesartan, have demonstrated a significant renal protective effect in the diabetic patient with nephropathy. There were no differences between the ARB and usual care groups with regard to cardiovascular outcomes.18
An optimal approach toward diabetic nephropathy combines control of hypertension, preferably with an ACE inhibitor or ARB; glycemic control; sodium restriction; and adjustment of protein intake.
If increasing macroalbuminuria occurs or if renal insufficiency is progressive despite these measures, the patient should be referred to a nephrologist. It is strongly recommended that renal arteriography be avoided. Dietary protein restriction in patients who have progressive renal insufficiency will reduce accumulation of nitrogen-containing waste products and can have a beneficial influence on progression of renal insufficiency.
Diabetic retinopathy is the most prevalent microvascular complication, affecting nearly 50% of the diabetic population at a given time and eventually occurring in all diabetic patients. Diabetes remains the leading cause of visual loss in adults. Visual loss from diabetes occurs either as a result of proliferative retinopathy or macular edema.19
CHD is strongly associated with type 2 diabetes mellitus and is the leading cause of death regardless of the duration of disease. There is a two- to four-fold increase in the relative risk ratio of cardiovascular disease in type 2 diabetes patients compared with the general population. This increase is particularly disproportionate in diabetic women when compared with diabetic men. The protection that premenopausal women have against CHD is not seen if they suffer from diabetes. The degree and duration of hyperglycemia are strong risk factors for the development of microvascular and macrovascular complications. Even impaired glucose tolerance increases cardiovascular risk, although there is minimal hyperglycemia.20
The first detectable sign of a problem in people genetically prone to develop type 2 diabetes is insulin resistance, which can be seen for as long as 15 to 25 years before the onset of diabetes. Several atherogenic factors are associated with insulin resistance, which can start the atherosclerotic process years before clinical hyperglycemia ensues. It is unclear whether the compensatory hyperinsulinemia plays a role in atherosclerosis generation in insulin-resistant patients. A number of prospective studies have shown an association between fasting or postprandial hyperinsulinemia and the future development of CHD. However, this association has been demonstrated in middle-aged white men but not in women or in other ethnic groups.21
Hyperglycemia itself plays an important role in enhancing the progression of atherosclerosis in type 2 diabetes. The threshold above which hyperglycemia becomes atherogenic is not known but may be in the range defined as impaired glucose tolerance (ie, fasting plasma glucose level <126 mg/dL with 30-, 60-, or 90-minute plasma glucose concentrations >200 mg/L and a 2-hour plasma glucose level of 140-200 mg/dL during an oral glucose tolerance test). Population-based studies show that the degree of hyperglycemia increases the risk for CHD and cardiovascular events.
Diabetic patients represent a high-risk group for developing and surviving acute MI. In particular, patients with type 1 diabetes have a worse outcome than patients with type 2 disease, and diabetic women have almost twice the risk of mortality of diabetic men.
Reperfusion therapy is the cornerstone of the management of acute MI. In a meta-analysis of the major trials comparing thrombolytic therapy to percutaneous coronary intervention (PCI), diabetic patients had significantly higher 30-day mortality when compared with nondiabetic patients (9.4% vs 5.9%, respectively; P < .001).22 The advantages of angioplasty over thrombolytic therapy in the diabetic population are comparable to those observed in nondiabetic patients.
New treatment strategies are emerging. The use of insulin and glucose infusion for at least 24 hours after admission followed by intensive long-term insulin was compared with usual care in the Diabetes Mellitus, Insulin Glucose Infusion in Acute Myocardial Infarction (DIGAMI) trial. A total of 620 diabetic patients were randomized, and the trial demonstrated a 30% reduction in mortality at 12 months for the group treated under the intensive regimen.23
However, the follow-up DIGAMI-2 trial, which compared three strategies—intensive insulin infusion followed by long-term insulin therapy, intensive insulin infusion followed by long-term standard glucose control, and regular metabolic control—in 1253 patients was unable to show a difference in short- and long-term mortality and morbidity between any of the arms. DIGAMI-2 concluded that intensive glucose control was of great importance in the peri-ischemic period irrespective of the strategy used to achieve it.24
The association between CHD and diabetes is strong and has led to screening strategies in diabetic patients even before they are symptomatic. In addition, diabetic patients often are unaware of myocardial ischemic pain, and thus, silent MI and ischemia are markedly increased in this population. There is a heightened concern for the development of sudden cardiac death in those with diabetes. Therapeutic modalities in diabetic patients with CHD revolve around standard therapy with aspirin, β-blockers, calcium channel blockers, and nitrates.
Epidemiologic evidence from the Bezafibrate Infarction Prevention Study registry shows almost a 50% reduction in mortality for type 2 diabetes patients with chronic CHD who were treated with β-blockers compared with controls. Other randomized trial evidence has demonstrated that diabetes is a strong predictor of death and that diabetic patients may benefit more from β-blocker therapy than nondiabetics. In general, β-blockers are extremely well tolerated, and masking or prolonging of hypoglycemic symptoms appears to be highly infrequent, particularly with cardioselective β-blockers.25
Diabetic cardiomyopathy is a term used by clinicians to encompass the multifactorial etiologies of diabetes-related left ventricular failure characterized by both systolic and diastolic function.26 The Framingham Heart Study showed that men with diabetes are twice as likely to develop congestive heart failure compared with their nondiabetic counterparts and females with diabetes had a five-fold increase in the rate of congestive heart failure. The spectrum of heart failure ranges from asymptomatic to overt systolic failure. Diabetes complicated by hypertension represents a particularly high-risk group for the development of congestive heart failure.27 Diastolic dysfunction is exceedingly common (>50% prevalence in some studies) and may be linked to diabetes without the presence of concomitant hypertension.
The etiology of impaired left ventricular function may involve any of the following mechanisms: (1) coronary atherosclerotic disease, (2) hypertension, (3) left ventricular hypertrophy, (4) obesity, (5) endothelial dysfunction, (6) coronary microvasculature disease, (7) autonomic dysfunction, and (8) metabolic abnormalities.
The diabetes and cardiovascular communities have embraced the concept of diabetic cardiomyopathy as a distinct entity independent of ischemic heart disease and hypertension. This was first described in the early 1970s when autopsy specimens of diabetic patients with nephropathy demonstrated a myopathic process in the absence of epicardial CHD.
Echocardiographic studies confirm that diastolic abnormalities occur in young diabetic patients who have no known diabetic complications.28 Diabetic patients who are hypertensive have increased left ventricular mass when compared with their nondiabetic counterparts, and left ventricular function may in fact be hyperdynamic. An Australian group has confirmed that diabetic patients demonstrate early findings of systolic dysfunction preceding any change in the left ventricular ejection fraction. The management of heart failure with preserved left ventricular systolic function usually includes β-blockers and ACE inhibitors, but evidence for this is sparse.
Once CHD develops in the diabetic patient, the left ventricular systolic dysfunction that ensues responds to all the same therapies as in the nondiabetic population. The findings of the Heart Outcomes Prevention Evaluation (HOPE) trial suggest that early initiation of ACE inhibition retards the progression to overt congestive heart failure.29
Compared with nondiabetic subjects, the mortality from stroke in diabetic patients is almost three-fold higher. The small paramedial penetrating arteries are the most common sites of cerebrovascular disease. In addition, diabetes increases the likelihood of severe carotid atherosclerosis. Diabetic patients are likely to suffer increased brain damage with carotid emboli that would result in a transient ischemic attack in a nondiabetic individual.30
Mechanisms Linking Diabetes and Cardiovascular Disease
The spectrum of metabolic disturbances associated with diabetes and insulin resistance extends beyond hyperglycemia and includes dyslipidemia, hypercoagulability, and inflammation.
Adipose tissue is an active organ that produces multiple adipokines, which play an important role in the development and progression of atherosclerosis.31Table 91–3 shows the prominent adipokines with their actions.
Adipokine | Actions |
---|---|
Adiponectin | Anti-inflammatory and antiatherogenic properties |
Low levels characteristic of persons at increased risk of diabetes | |
Decreases foam cell formation | |
Decreases uptake of oxidized LDL | |
Decreases monocyte adhesion to endothelial cells | |
Decreases expression of adhesion molecules | |
Decreases proliferation and migration of vascular smooth muscle cells | |
Leptin | Regulates energy intake and expenditure |
Enhances cellular immune responses | |
Increases blood pressure levels | |
Angiotensinogen and angiotensin II | Vasoconstrictive |
Enhances the formation of foam cells | |
Stimulates intracellular adhesion molecule-1, vascular cell adhesion molecule-1, MCP-1, and M-CSF expression in the cells of the vessel wall | |
Increases monocyte-macrophage platelet activity in the vessel wall | |
Endothelial dysfunction | |
Tumor necrosis factor (TNF) | Expression of adhesion molecules on the surface of the endothelial cells and VSMCs |
Plasminogen activator inhibitor-1 (PAI-1) | Inhibits the breakdown of fibrin clots Promotes thrombus formation |
The normal endothelium regulates vasomotor tone and keeps the coagulation cascade in balance. Insulin resistance, itself, appears to be an endothelial dysfunction risk equivalent (Fig. 91–3).32
Figure 91–3.
Generation of a dysfunctional endothelium caused by diabetes. See text for further details. AGE, advanced glycosylation end-product; EDRF, endothelium-derived relaxing factor; NF-kB, nuclear factor-κB; PAI-1, plasminogen activator inhibitor-1; TF, tissue factor; VCAM, vascular cell adhesion molecule. Reprinted from Aronson D, Bloomgarden Z, Rayfield EJ. Potential mechanisms promoting restenosis in diabetes mellitus. J Am Coll Cardiol. 1996;27:528-535, Copyright (1996), with permission from Elsevier.
In healthy endothelium, the stimulation of insulin receptors activates the phosphoinositol-3 kinase (PI3-K) pathway. The production of nitric oxide by endothelial cells stimulated by this pathway leads to a host of anti-inflammatory and antithrombotic effects that are antiatherogenic. The endothelial dysfunction of insulin resistance is a result of decreased levels of nitric oxide and thus impaired blood flow due to downregulation of the PI3-K pathway.
A parallel pathway downregulates the production of endothelin-1. In response to hyperglycemia, endothelial cells secrete endothelin-1, which induces proatherogenic effects such as vasoconstriction, increased vascular permeability, and vascular smooth muscle cell (VSMC) proliferation. Elevated glucose levels stimulate the production of interleukin-6 by endothelial cells and monocytes and increased proteoglycan synthesis by VSMCs.
The insulin receptors on VSMCs are structurally and functionally similar to those in skeletal muscle and adipocytes. Insulin signaling in VSMCs initiates proatherogenic cellular events such as proliferation and migration. Insulin acts synergistically with other atherogenic growth factors, such as platelet-derived growth factor, to promote the proliferation and migration of bovine aortic smooth muscle cells.
Circulating monocytes are important inflammatory cells involved in the immune response.33 Insulin and insulin-like growth factor-1 receptors are present on circulating monocytes and macrophages. Defective insulin signaling is implicated in macrophage foam cell formation. Under conditions of insulin resistance, the protective effect of insulin to reduce macrophage apoptosis may be lost. Macrophage apoptosis in atherosclerotic lesions may contribute to further monocyte recruitment by the release of cytokines and may thus accelerate the development of the vascular lesion and contribute to plaque rupture.
The insulin resistance syndrome is a composite of dyslipidemia, hypertension, and hypercoagulability.34 It is only now being recognized that insulin resistance is the predominant defect in >90% of type 2 diabetes patients and the major pathologic mechanism for the susceptibility to premature cardiovascular disease. Insulin resistance and hyperinsulinemia accelerate the development of atherosclerosis. Hyperinsulinemia is an independent risk factor when adjusted for lipid profile, hypertension, and family history. Studies of multiple ethnic groups show increased carotid intima-medial thickness (a reliable marker for coronary disease) in subjects with insulin resistance. Impaired glucose tolerance can increase the risk of heart disease. Because insulin resistance precedes clinically diagnosed type 2 diabetes by 10 to 15 years in as many as 90% of patients, this extensive period of atherogenic exposure may account for the higher rates of cardiovascular disease in type 2 diabetics.35,36
Although the euglycemic insulin clamp remains the gold standard for the measurement of insulin resistance, simpler formulae are used clinically to quantify insulin resistance using the Homeostasis Model Assessment for insulin resistance (HOMA-IR = Serum insulin × Serum glucose/22.5).35 Insulin resistance as assessed by HOMA-IR also has been shown to be predictive of cardiovascular disease. In long-term follow-up of type 2 diabetic patients, insulin resistance was independently predictive of cardiovascular disease, with a 1-unit increase in HOMA-IR associated with a 5.4% increased risk of cardiovascular disease.35 The Insulin Resistance and Atherosclerosis Study (IRAS) also demonstrated the relationship between insulin resistance and atherosclerosis in the carotid artery.
Management of Diabetes and Its Complications
The American Diabetes Association (ADA) and American Heart Association (AHA) have published a combined scientific statement to address primary prevention of cardiovascular disease in patients with diabetes.37
Steno-238 demonstrated that a comprehensive multifactorial strategy (including lifestyle and pharmacologic interventions) to reduce cardiovascular risk in type 2 diabetic patients with microalbuminuria was highly effective (hazard ratio [HR] = 0.47; 95% confidence interval [CI], 0.24-0.73) when compared with usual care after a mean time of 7.8 years (Table 91–4). The number needed to treat to prevent a major cardiovascular event was only five patients. The approach included targets of hemoglobin A1c (HbA1c) <6.5%, blood pressure <130/80 mm Hg, total cholesterol <175 mg/dL, and triglycerides <150 mg/dL. Patients were prescribed aspirin and an ACE inhibitor or ARB. This study validates the multidisciplinary approach to the cardiovascular care of the diabetic patient.
Figure 91–4.
A. Steno-1: incidence of cardiovascular events in diabetics comparing conventional versus intensive therapy. Cumulative events included major cardiovascular events, death due to cardiovascular causes, nonfatal cerebrovascular accident, nonfatal acute myocardial infarction, revascularization, and amputation. B. Steno-2: incidence of death due to any cause in diabetics comparing conventional versus intensive treatment. Reproduced with permission from Gaede P, Lund-Andersen H, Parving HH, et al. Effect of a multifactorial intervention on mortality in type 2 diabetes. N Engl J Med. 2008;358(6):580-589.
Trial | Treatment | Outcome | Events in Control Group | Events in Treatment Group | Relative Risk Reduction (%) | Number Needed to Treat | P |
---|---|---|---|---|---|---|---|
Steno-239; n = 160 | Intensive, comprehensive (includes hypertension, dyslipidemia, and glycemic control) therapy vs standard therapy | Macrovascular events: death, myocardial infarction, stroke, vascular ischemia | 42/78 (53.8%) | 26/77 (33.7%) | 37.3 | 5 | .03 |
When evaluating a multifactorial medical intervention focusing on glycemic control, lipid reductions, and aggressive blood pressure targets, it is clear that the control of macrovascular complications in patients with diabetes extend far beyond glucose levels. This strategy was analyzed in the Steno-2 study, in which a multifactorial approach was associated with a tight glycemic control versus conventional therapy in 160 patients with type 2 diabetes and microalbuminuria with a mean follow-up period of 7.8 years. After 13 years of follow-up, patients randomized to intensive medical management experienced a significant decrease in overall mortality (46%; 95% CI, 32%-89%; P = .02), cardiovascular death (57%; 95% CI, 19%-94%; P = .01), and combined cardiovascular events (59%; 95% CI, 25%-67%; P < .001), in addition to experiencing a decrease in the risk of nephropathy and microvascular complications (Fig. 91–4).
Weight loss is an important therapeutic strategy in all overweight or obese individuals who have type 2 diabetes or are at risk for developing diabetes.39,40 The primary approach for achieving weight loss, in the vast majority of cases, is therapeutic lifestyle change, which includes a reduction in energy intake and an increase in physical activity. A moderate decrease in caloric balance (500-1000 kcal/d) will result in a slow but progressive weight loss (1-2 lb/wk). For most patients, weight loss diets should supply at least 1000 to 1200 kcal/d for women and 1200 to 1600 kcal/d for men. In selected patients, drug therapy to achieve weight loss as an adjunct to lifestyle change may be appropriate. However, it is important to note that regain of weight commonly occurs on discontinuation of medication.
Physical activity is an important component of a comprehensive weight management program. Regular moderate-intensity physical activity enhances long-term weight maintenance. Regular activity also improves insulin sensitivity, glycemic control, and selected risk factors for cardiovascular disease (ie, hypertension and dyslipidemia), and increased aerobic fitness decreases the risk of CHD. Initial physical activity recommendations should be modest, based on the patient’s willingness and ability, gradually increasing the duration and frequency to 30 to 45 minutes of moderate aerobic activity 3 to 5 days per week, when possible. Greater activity levels of at least 1 h/d of moderate (walking) or 30 min/d of vigorous (jogging) activity may be needed to achieve successful long-term weight loss. The American College of Sports Medicine now recommends that resistance training be included in fitness programs for adults with type 2 diabetes. Resistance exercise improves insulin sensitivity to about the same extent as aerobic exercise.
In patients with severe/morbid obesity, surgical options, such as gastric bypass and gastroplasty, may be appropriate and allow significant improvement in glycemic control with reduction or discontinuation of medications. A recent randomized trial of gastric banding compared with conventional therapy showed a greater remission rate of type 2 diabetes through a surgical approach. In the surgical group, 73% of patients experienced a remission of type 2 diabetes compared with 13% in the conventional group.41 It is important to fully evaluate the patient for existing risk for cardiovascular disease and to improve glycemic control preoperatively so as to decrease the risk of complications. It is also important to counsel patients on the risks of surgery, including mortality, depression, hypoglycemia, nutritional deficiencies, osteoporosis, and weight regain over the long term. Little data are currently available on the long-term consequences of surgery for weight loss in people with diabetes. The potential benefits should be weighed against short- and long-term risks.39
Every patient with diabetes should be provided with diabetes education. Recommendations for glucose testing vary among individuals and depend on the current degree of control and whether the patient is taking a medication that would potentially cause hypoglycemia. It is often useful to have patients test at different times on different days, such as in the morning prior to eating (fasting glucose), at bedtime, before lunch or dinner, 2 hours after meals, and when the patient feels sick. The patient will want to know the reason for frequent testing because, unlike patients with type 1 diabetes, insulin cannot be administered to acutely lower blood glucose levels if they are receiving oral hypoglycemic agents. For patients to adhere to frequent testing regimens, they must understand what foods, exercise, and circumstances will have an impact on blood glucose levels, as well as how they should modify their behaviors for optimal glycemic control (Table 91–5 and Fig. 91–5).
The San Antonio Heart Study showed a proportional increase in cardiovascular-related deaths with higher fasting blood glucose levels in type 2 diabetics.42 Although there are abundant data linking both fasting glucose and impaired glucose tolerance to adverse events, the data demonstrating an improvement in cardiovascular outcomes with an aggressive glucose-lowering treatment strategy have been lacking among patients with type 2 diabetes. Although data from the UKPDS study43 clearly demonstrated a reduction in microvascular complications with intensive glucose control, there was not a concomitant significant reduction in macrovascular complications, despite a disproportionate 25% risk of suffering a nonfatal MI or stroke (compared with a 3.4% incidence of developing blindness or a 1% incidence of developing renal failure) during a 10-year period. However, it is important to remember that UKPDS only studied patients with new-onset diabetes mellitus. Recent data from the Prospective Pioglitazone Clinical Trial in Macrovascular Events (PROACTIVE) study failed to show a significant reduction in the composite macrovascular outcomes with glucose reduction, although the secondary outcome of rate of major adverse cardiac and cerebrovascular events was reduced by a significant 16%.44
The medical community has progressively become more and more aggressive about risk factor modification. Multiple trials in cardiovascular prevention have shown that modification of traditional risk factors shows more benefit than glycemic control. Practitioners, however, must realize that because of the importance of glycemic control in preventing microvascular complications, cardiologists have adopted measures to control blood glucose levels.
The AHA and ADA have come to consensus for goals of targets. The HbA1c goal for patients in general is <7%.45 The American College of Clinical Endocrinology goal is an HbA1c of <6.5%.46 However, the ideal HbA1c goal for the individual patient is as close to normal (<6%) as possible without significant hypoglycemia. The debate presently is also whether getting to the goal is enough or whether how one gets there is important as well. The Bypass Angioplasty Revascularization Investigation (BARI) 2D study provided more data on whether management of insulin resistance with insulin-sensitizing therapies led to better outcomes than insulin-replacing therapies.47 The study showed that there was no difference in mortality at 5 years whether they used insulin-sensitizing or insulin-replacing therapies (see p. 2051).
The guidelines currently recommend treating to target and acknowledge that most type 2 diabetic patients may require more than one antidiabetic agent. Most agents have been studied to measure efficacy as monotherapy and across the board demonstrate a 40% to 60% reduction in fasting plasma glucose level. The following discussion outlines the mechanisms, advantages, and disadvantages of each of these therapies as individual agents.
Insulin is used in the management of type 1 or type 2 diabetes mellitus as monotherapy or in combination with oral agents. Insulins currently available are synthetic human insulins or analogs of human insulin, which differ in their rate of absorption and duration of action. There are also products that are mixtures of rapid short-acting and intermediate-acting insulins (Table 91–6). Purified animal insulins are no longer used.
Type | Name | Onset of Action | Time to Peak Activity | Duration of Action |
---|---|---|---|---|
Rapid acting | Aspart | 15 min | 1 h | 3-4 h |
Glulisine | 15 min | 30-90 min | 3-5 h | |
Lispro | 15 min | 1 h | 3-4 h | |
Short acting | Regular | 30-60 min | 2-4 h | 6-8 h |
Intermediate acting | NPH | 1-3 h | 6-8 h | 12-16 h |
Long acting | Detemir | 1 h | No peak | About 12 h |
Glargine | 1-2 h | No peak | About 24 h |
Recombinant human insulin (RHI) is structurally identical to human insulin and is synthesized by Escherichia coli bacteria. It consists of zinc insulin crystals dissolved in clear fluid. There are four rapid-acting insulins currently available. Insulin lispro is a rapid-acting insulin analog in which the amino acids at positions 28 and 29 on the human insulin B chain are reversed. Insulin aspart is a second rapid-acting insulin analog with a substitution of aspartic acid for proline in position 28 on the B chain. Insulin glulisine is the newest rapid-acting analog in which the asparagine at position 3 on the B chain is replaced by glutamic acid. These amino acid changes result in a reduced propensity for insulin molecules to form aggregates (dimers and hexamers), providing a more rapid onset and shorter duration of action than RHI. These insulins are used to cover carbohydrates at mealtime to correct for an elevated glucose level and in insulin pumps. The latest rapid-acting insulin is Exubera (a human insulin of rDNA origin), which is inhaled and has an intermediate duration of action (387 minutes) that is faster than lispro and comparable to regular insulin.48
Exubera comes in “blisters” of 1 and 3 mg, with each 1 mg of powder approximately equal to 3 units of injected regular insulin. When the blister is placed into an inhaler, a cloud of aerosolized insulin is inhaled.49
Neutral pH protamine Hagedorn (NPH) is an intermediate-acting insulin that is a suspension of RHI with protamine, thus delaying its absorption. NPH can be used at bedtime to normalize fasting glucose and in combination with rapid-acting insulins during the daytime to provide primarily basal coverage.
Insulin glargine is an insulin analog in which the asparagine residue at position A21 is replaced with glycine and two arginine residues are added to the B chain C terminus. Insulin glargine has a pH of 4 in solution, but after subcutaneous injection, it has a pH of 7.4 microprecipitates, which delays its absorption. It is essentially peakless, with a 24-hour duration of action. Insulin detemir is a soluble long-acting human insulin analog with the elimination of the threonine in position B30 and the addition of a 14-carbon fatty acid chain at position B29. Insulin detemir is absorbed slowly from the injection site and is >98% reversibly bound to albumin in the blood. The basal insulins are used once or twice daily to provide broad coverage.
The most physiologic way of administering insulin therapy is to give a basal insulin once or twice daily, as well as a bolus of insulin prior to each meal based on carbohydrate counting and a correction factor to bring the glucose down to premeal levels by 2 hours after eating. This basal-bolus regimen provides better glycemic control than the control obtained using mixed insulin preparations. There is less hypoglycemia with bedtime administration of insulin glargine or detemir than with NPH, because the glucose-lowering effect is slow and sustained over a period approximating 24 hours.50
Insulin allergies are uncommon with the recombinant preparations of insulins. Unused insulin vials, cartridges, and pens should be kept refrigerated and will stay potent until the expiration date. Once opened, insulin glargine must be changed every 28 days, regardless of whether or not it is refrigerated. Mixed analogs in a pen should be discarded after 10 to 14 days.
Insulin pumps are devices with a subcutaneous catheter that deliver continuous subcutaneous insulin infusion. One or more basal rates are preprogrammed by the user, and boluses are taken as needed whenever carbohydrates are ingested. The catheter is changed every 2 to 3 days, and abdominal infusion sites are most commonly used. In a motivated patient, better glycemic control can be achieved with continuous subcutaneous insulin infusion—compared with multiple subcutaneous insulin injections—because continuous subcutaneous insulin infusion can provide multiple basal rates of insulin. Pumps are generally used in patients with type 1 diabetes but can also be used in patients with type 2 diabetes. A meta-analysis by Weissberg-Benchell et al51 of 52 studies concluded that continuous subcutaneous insulin infusion is associated with improved HbA1c and mean blood glucose levels. Bode et al52 demonstrated that mean HbA1c levels decreased from 8.3% to 7.5% with continuous subcutaneous insulin infusion, with a significant reduction in severe hypoglycemia, compared with multiple insulin injections during the first year of therapy. Patients who counted carbohydrates, checked their glucose levels three or more times a day, and recorded their glucose levels in a logbook had better glycemic control than those who did not.
Continuous glucose monitoring systems that use a glucose sensor to provide up to 3 days of continuous glucose monitoring in the subcutaneous tissue are available. The record shows glucose patterns and trends that can help in the recognition and prevention of hypoglycemia, hyperglycemia, postprandial glucose excursions, and the effects of exercise. However, “normal” interstitial glucose values may be lower than realized, including some values in the “hypoglycemic range.” Also, the continuous glucose monitoring system may not always read the glucose concentrations consistently and accurately. Recently, two systems for real-time continuous glucose monitoring became available: the Dex Com and the Guardian RT. Outcome data with these devices should be available soon.
Multiple other agents are used in the management of diabetes. Table 91–7 summarizes these agents, and they are described in the following sections.
Drug Class | Generic Name | Mechanism of Action | Expected HbA1c Reduction (%) | Daily Dose |
---|---|---|---|---|
Biguanide | Metformin | Decreases hepatic glucose output | 1.0-2.0 | 500 mg qd to 1000 mg bid to 850 mg tid |
Thiazolidinedione | Pioglitazone | PPARα and PPARγ agonist | 1.0-1.5 | 15-45 mg qd |
Rosiglitazone | PPARγ agonist | 1.0-1.5 | 2 mg qd or bid, 8 mg qd | |
Sulfonylurea | Glipizide | ↑ β-cell insulin synthesis and release | 1.0-2.0 | 2.5-20 mg qd or bid |
Glyburide | ↑ β-cell insulin synthesis and release | 1.25-10 mg qd or bid | ||
Glimepiride | ↑ β-cell insulin synthesis and release | 0.5-8 mg qd | ||
Meglitinides | Repaglinide | ↑ β-cell insulin synthesis and release | 1.0-2.0 | 0.5-4 mg tid |
Nateglinide | ↑ β-cell insulin synthesis and release | 0.5-1.0 | 60-120 mg tid | |
α-Glucosidase inhibitors | Acarbose | α-Glucosidase inhibition (decreased carbohydrate absorption) | 0.5-1.0 | 50-100 mg tid |
Miglitol | α-Glucosidase inhibition | 0.5-1.0 | 500-100 mg tid | |
Incretin mimetic | Exenatide | Increasing postprandial insulin, decreasing postprandial glucagon, increasing satiety, and delaying gastric emptying | 0.5-0.9 | 5-10 μg bid |
Metformin has been used in Europe for several decades but has been marketed in the United States since 1995. The mechanism of action of metformin is to decrease hepatic glucose output by inhibiting glucose-6-dehydrogenase activity and stimulating the insulin-induced component of glucose uptake into skeletal muscle and adipocytes.
The starting dose for metformin is 500 mg orally with dinner for 1 week and then 500 mg orally with breakfast and dinner. A sustained-release preparation is available that allows once-daily dosing. Because of its mechanism of action, there is minimal risk for hypoglycemia. This drug should not be used in renal failure or potential hypoxic states, such as congestive heart failure and severe pulmonary disease, because of the risk of lactic acidosis. The risk of lactic acidosis is low and is estimated to be 9 per 100,000 person-years. The most common adverse effects are gastrointestinal—nausea, diarrhea, and abdominal pain—and a metallic taste. It should not be used in patients with impaired renal function (serum creatinine >1.5 mg/dL in men and >1.4 mg/dL in women). Caution should be exercised in prescribing metformin to the elderly. If used in patients older than age 80 years, then a normal glomerular filtration rate should be documented. Metformin should be discontinued on the day patients receive an iodinated contrast material for radiographic studies, which can temporarily impair renal function, as well as prior to any surgical procedure. The metformin dose can be resumed 48 hours later if the serum creatinine is in the normal range. Metformin lowers the HbA1c by 1% to 2%. Metformin can be used to treat the metabolic syndrome because it lowers serum concentrations of triglycerides, plasminogen activator inhibitor-1 activity, and body weight.
Thiazolidinediones (TZDs) induce peroxisome proliferator-activated receptor (PPAR) γ binding to nuclear receptors in muscle and adipocytes, allowing insulin-stimulated glucose transport. Three PPARs have been identified to date: PPARα, PPARδ (also known as PPARβ), and PPARγ. After ligand binding, PPARs change their conformation to permit the recruitment of one or more coactivator proteins. The first mechanism, transactivation, is DNA dependent and consists of binding PPAR components with target genes and heterodimerization with the retinoid X receptor. The second mechanism, transrepression, interferes with other transcription factor pathways that are not DNA dependent. The protein kinase C signaling pathway functions as a molecular switch that dissociates with transactivation and transrepression properties of PPARα. PPARα resides mainly in the liver, heart muscle, and vascular endothelium; when it is activated, it controls genes that regulate lipoprotein levels and confers anti-inflammatory effects. PPARγ is located mainly on adipocytes but is also found in pancreatic β cells, vascular endothelium, and macrophages.
TZDs lower fasting and postprandial glucose levels, as well as free fatty acid levels. A first-generation TZD, troglitazone, is no longer available because of its hepatotoxicity. The second-generation TZDs rosiglitazone and pioglitazone may be used as monotherapy or in combination with insulin, sulfonylureas, or metformin, and have not been found to be hepatotoxic. TZDs are associated with weight gain caused by fluid retention and proliferation of adipose tissue.56-58 However, the TZDs increase fat in the subcutaneous adipose tissue and decrease visceral adipose tissue and fat in the liver. The dose for rosiglitazone is 2 to 8 mg/d; for pioglitazone, it is 15 to 45 mg/d. Two adverse effects to be noted are peripheral tissue edema and, less frequently, congestive heart failure. TZDs are contraindicated in patients with congestive heart failure and should be used cautiously in at-risk patients.
TZDs decrease HbA1c levels by 1% to 1.5%. These agents decrease insulin resistance and possibly preserve β-cell function. The Troglitazone in the Prevention of Diabetes (TRIPOD)59 study was designed to investigate the preservation of pancreatic β-cell function with TZD. Hispanic women with a prior medical history of gestational diabetes were randomized to receive troglitazone 400 mg daily or a placebo. Women who did not develop diabetes were asked to return 8 months after the trial for an oral glucose tolerance test and an intravenous glucose tolerance. The median follow-up was 30 months. The troglitazone group had a 56% reduction in the incidence of type 2 diabetes for as long as 8 months after the medications were stopped. This protection against earlier development of diabetes was associated with a reduction in insulin resistance. Pioglitazone acts like a partial PPARα agonist and has beneficial effects on the lipid profile, increasing HDL cholesterol, decreasing triglycerides, and improving low-density lipoprotein (LDL) cholesterol subtypes. Dual PPARα/γ agonists have not been approved by the US Food and Drug Administration (FDA) to date because of safety concerns.
The TZDs have come under increased scrutiny because of the association with edema and weight gain. What is clear after a number of key trials is that there are likely differences between the two approved PPAR agents pioglitazone and rosiglitazone.60 Pioglitazone and rosiglitazone have significantly different effects on plasma lipids.61,62 Pioglitazone compared with rosiglitazone is associated with significant improvements in LDL particle concentration, LDL particle size, triglycerides, and HDL cholesterol. When prescribing TZDs, it is essential to balance the risk and benefits. Clearly, patients with congestive heart failure are not candidates for TZD therapy.
A number of trials have been published suggesting a cardioprotective effect of pioglitazone.63 The Carotid Intima-Media Thickness in Atherosclerosis Using Pioglitazone (CHICAGO) trial compared the 18-month effects of pioglitazone versus glimepiride on carotid intima-medial thickness and showed that the progression of mean carotid intima-medial thickness was less with pioglitazone, with a difference between groups of 0.013 mm favoring the pioglitazone group.64 Subsequently, the Pioglitazone Effect on Regression of Intravascular Sonographic Coronary Obstruction Prospective Evaluation (PERISCOPE) trial confirmed the CHICAGO findings in an intravascular ultrasonography primary end point. Again, a lower rate of progression of coronary atherosclerosis was found with pioglitazone compared with glimepiride after 18 months of therapy.65
The pivotal outcomes trial of pioglitazone, PROACTIVE, did not provide as clear a picture as the surrogate outcomes trials.44 In this trial, >5000 diabetic patients with established macrovascular complications were randomized to pioglitazone 45 mg/d or placebo. No differences were found in the primary end point, which was a composite of major adverse cardiac event (MACE), leg amputation, peripheral and coronary revascularization, and acute coronary syndrome. However, a key secondary end point of death, MI, and stroke showed a 16% relative risk reduction in favor of pioglitazone (HR = 0.84; 95% CI, 0.72-0.98; P = .027). The potential reduction in cardiovascular events associated with pioglitazone is supported by a meta-analysis of 19 trials demonstrating a lower risk for a composite of MACE (HR = 0.82; 95% CI, 0.72-0.94; P = .005). A comprehensive meta-analysis did confirm a favorable cardiovascular risk profile for pioglitazone.66
The evidence for a cardioprotective effect of rosiglitazone is not apparent. In fact, the meta-analysis by Nissen and Wolski66 created a media firestorm by reporting a 43% excess risk for MI and a 60% excess risk for cardiovascular death in type 2 diabetes patients treated with rosiglitazone compared with an active comparator.67 This meta-analysis was highly criticized for a number of reasons, including pooling trials for which cardiovascular end points were relatively rare and with a great deal of heterogeneity between trials. What was most controversial is that it led to the publishing of an interim analysis of the Rosiglitazone Evaluated for Cardiac Outcome and Regulation of Glycemia in Diabetes (RECORD) trial.68 Eventually, RECORD was published.69 The trial randomized >4000 patients in an open-label design. After a mean follow-up of 5.5 years, the addition of rosiglitazone to a combination of metformin and sulfonylurea was found to be noninferior to a combination of metformin and sulfonylurea for the combined end point of cardiovascular hospitalization and cardiovascular death (HR = 0.99; 95% CI, 0.85-1.16). Heart failure causing admission to hospital or death was increased in the rosiglitazone group (HR = 2.10; 95% CI, 1.35-3.27).
Sulfonylureas are the oldest class of treatment for type 2 diabetes. The mode of action is by stimulating β-cell insulin secretion (Fig. 91–6). The β-cell sulfonylurea receptor (SUR) is functionally linked to an adenosine triphosphate (ATP)-sensitive K+ channel (K+-ATP) on the cell membrane. In the basal state, the K+-ATP channel shifts K+ from the inside of the β cell to the extracellular space and maintains the resting potential of the β-cell membrane. When the sulfonylurea binds to the SUR, K+ efflux diminishes and the membrane depolarizes. This depolarization opens a voltage-dependent calcium channel in the same membrane, which enables extracellular calcium to enter the cell. The resultant increase in intracellular calcium triggers insulin-containing secretory granule exocytosis.
The first-generation sulfonylureas have a long half-life and bind ionically to plasma proteins, making them easily displaced. The major concern with these agents is hypoglycemia. The second-generation sulfonylureas have a shorter half-life and bind to plasma proteins nonionically, making them less easily displaced from proteins and available for binding to receptors. Commercially available second-generation sulfonylureas are glyburide (1.25-20 mg/d), glipizide (2.5-40 mg/d), and glimepiride (1-8 mg/d). Sulfonylureas decrease the HbA1c by 1% to 2%.
Repaglinide is a member of the meglitinide group of insulin secretagogues with a relatively short half-life of 3.7 hours. The binding site on the SUR is distinct from the binding site for sulfonylureas. The drug is taken up to 30 minutes prior to each meal. Repaglinide is particularly useful in the elderly, patients with chronic renal insufficiency, and patients who are erratic eaters. The dose varies between 0.5 and 4 mg before meals. Repaglinide results in a 1% to 2% decrease in HbA1c.
Nateglinide, a derivative of phenylalanine, is structurally distinct from both sulfonylureas and repaglinide. It has a quicker onset and shorter duration of action than repaglinide. Nateglinide is available as 60- and 120-mg tablets, taken with each meal. It is effective for lowering postprandial glucose levels. Nateglinide results in a 0.5% to 1.0% decrease in HbA1c. As with repaglinide, the dose of nateglinide should be omitted if a meal is skipped.
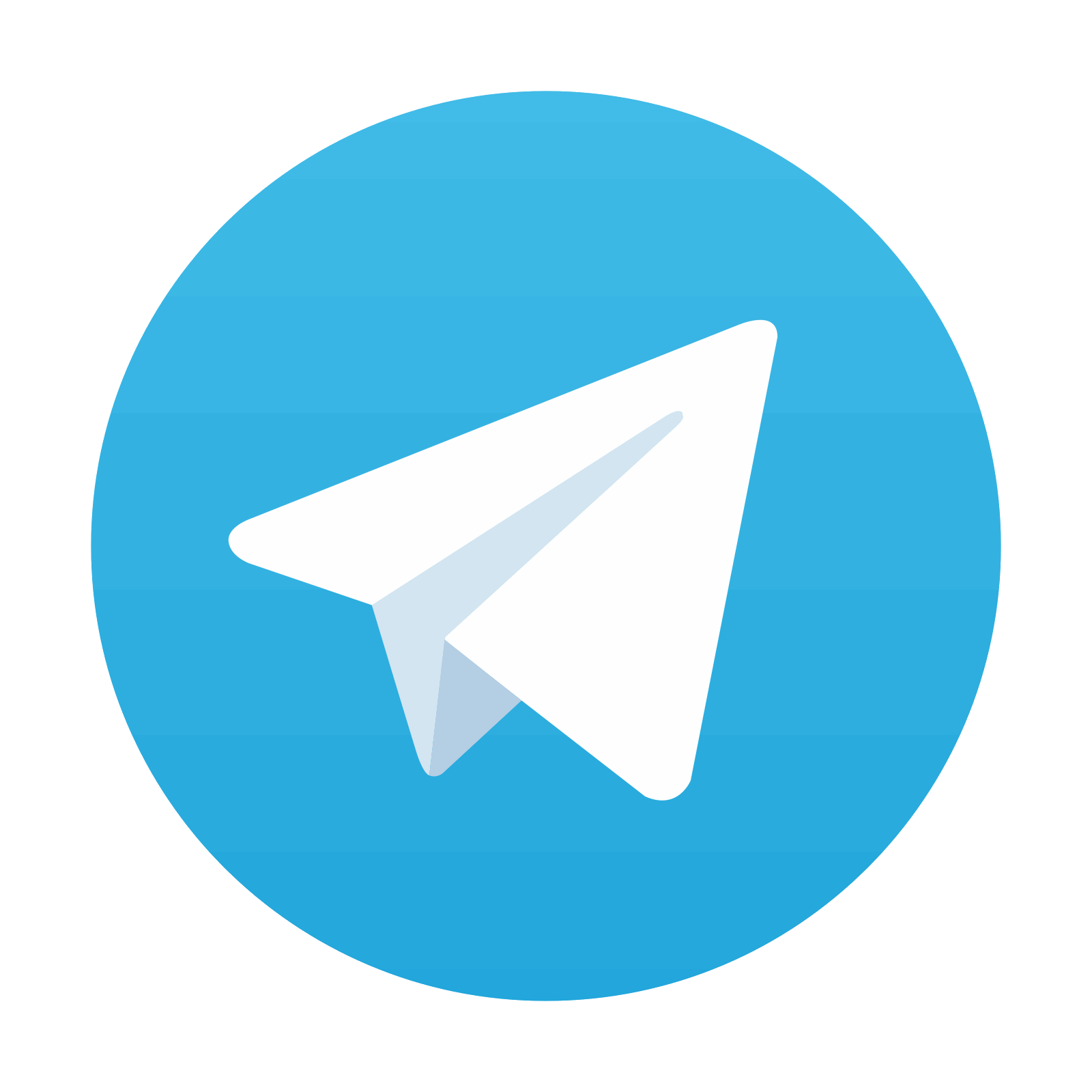
Stay updated, free articles. Join our Telegram channel

Full access? Get Clinical Tree
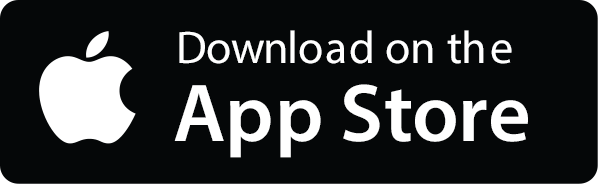
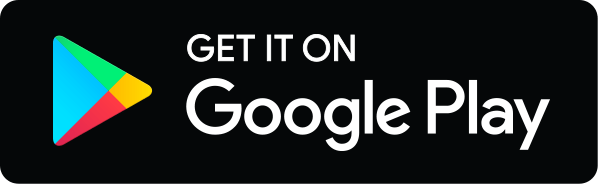