Epicardial fat with its close proximity to coronary arteries has been suggested to be a significant predictor of cardiovascular disease. We studied the relations among acquired obesity, low-grade inflammation, and genetic factors in the accumulation of epicardial fat. A rare sample (n = 15) of healthy monozygotic (MZ) twin pairs discordant for obesity (intrapair difference in body mass index ≥3 kg/m 2 ) and 9 concordant MZ pairs 23 to 33 years old were examined for cardiac structure, function, epicardial fat thickness (echocardiography), abdominal subcutaneous tissue, and visceral adipose tissue (VAT), liver fat (magnetic resonance imaging/spectroscopy), and serum high-sensitivity C-reactive protein. In the entire sample, MZ cotwins were remarkably similar in most echocardiographic measurements including epicardial fat (intraclass correlation 0.63, p = 0.0004). However, in the discordant pairs, the obese cotwins (16.5 kg, 23% heavier) had 26% more epicardial fat (p = 0.0029) than nonobese cotwins. They also had significantly larger atrial and left ventricular dimensions. Epicardial fat correlated with VAT (r = 0.49, p = 0.02) in individual twins and when using intrapair differences of measurements within pairs (r = 0.39, p = 0.06). In multiple regression analyses including abdominal subcutaneous tissue, VAT, and liver fat, high-sensitivity C-reactive protein was the only factor that remained significantly associated with epicardial fat in individual twins and within pairs. In conclusion, subjects who share the same genes seem to have similar cardiac dimensions. However, acquired obesity increases epicardial fat independent of genetic factors. The close relation between epicardial fat and low-grade inflammation is likely to contribute to the development of cardiovascular disease in obesity.
Because body mass index (BMI) and cardiac dimensions have a substantial heritability, it is possible that genetic influences explain associations between adiposity and cardiac structure and function. Indeed, pleiotropic genetic loci contributing to left ventricular (LV) thickness and BMI have already been described. No studies thus far, however, have addressed the question to what extent the accumulation of epicardial fat is genetic. Moreover, there is no evidence of the relations between obesity and cardiac structure independent of potential genetic confounding factors. Study of monozygotic (MZ) twin pairs discordant for obesity offers a unique opportunity to determine whether acquired obesity increases epicardial fat thickness or changes atrial/ventricular structure or function. MZ twins are matched for genes and for age, gender, and ethnicity, and MZ twins often share the same environment, particularly during childhood. In this study we sought to evaluate the relation among epicardial fat thickness, low-grade inflammation, and cardiac dimensions independent of genetic influences by studying young adult MZ twins discordant for obesity.
Methods
The study subjects were selected from 2 population-based longitudinal studies, FinnTwin16 (FT16) and FinnTwin12 (FT12), each consisting of 5 consecutive birth cohorts of Finnish twins. All twins were identified through Finland’s central population registry, permitting exhaustive and unbiased ascertainment of all twins living and resident in the country. In FT16, twins born from 1975 through 1979 were followed up by questionnaires at 16, 17, 18.5, and 22 to 27 years of age (response rates 83% to 97%, n = 5,601 at baseline). In FT12, twins born from 1983 through 1987 were sent questionnaires at 11 to 12, 14, and 17.5 years of age; a subsample was invited to a clinical assessment at 20 to 25 years of age (response rates 74% to 92%, n = 5,184 at baseline).
The metabolic study on twins, TwinFat, started in 2002 with the identification and examination of all (n = 14) obesity-discordant MZ pairs from the last follow-up of FT16 at 25 years where (1) the intrapair difference in BMI was ≥3 kg/m 2 and (2) 1 twin was obese and the cotwin was not. Results from this study have been reported previously and reviewed recently. These twins were recontacted in 2008 for the present study. Of the 14 pairs originally identified and examined in 2002, 9 were re-examined in 2008. Of these, only 5 met the obesity-discordance criteria, and the remaining 4 were no longer discordant. We then searched for new obesity-discordant MZ pairs from the last follow-up of FT12 and found an additional 10 discordant pairs. Five pairs with reported or measured obesity discordance from the FT12 and FT16 databases were examined, although discordance criteria were not currently met. The intensive sample in the present study thus consisted of 15 obesity-discordant (intrapair difference in BMI ≥3 kg/m 2 ) pairs (6 men, 9 women). The 9 currently concordant (intrapair difference in BMI <3 kg/m 2 ) pairs consisted of 7 men and 4 women pairs.
MZ twin pairs were examined for their cardiac structure and function and epicardial fat thickness by echocardiography and for body composition by dual-energy x-ray absorptiometry (percent whole-body fat), magnetic resonance imaging (abdominal subcutaneous fat and intra-abdominal fat), and magnetic resonance spectroscopy (liver fat content). Fasting blood samples were taken to measure low-grade inflammation by high-sensitivity C-reactive protein (hs-CRP).
One obese man cotwin had developed type 2 diabetes mellitus and was on metformin and insulin. His echocardiographic measurements and blood pressure measurements were in the same range as in other obese cotwins, so this pair was kept in the analyses. One obese woman cotwin used mesalazine and azathioprine for currently inactive colitis ulcerosa. All other subjects were clinically healthy and normotensive and did not use any medications except for oral contraceptives. Their weight had been stable for ≥3 months before the study. Zygosity was confirmed by genotyping of 10 informative genetic markers. Subjects provided written informed consent. The protocol was designed and performed according to principles of the Declaration of Helsinki and was approved by the ethical committee of the Helsinki University Central Hospital.
Weight and height were measured after a 12-hour overnight fast while barefoot and in light clothing to calculate BMI. Normal weight was defined as BMI <25 kg/m 2 , overweight as BMI of 25 to 29.9 kg/m 2 , and obese as BMI ≥30 kg/m 2 . Waist circumference was measured midway between spina iliaca superior and the lower rib margin. Body composition was assessed using whole-body dual-energy x-ray absorptiometric scans (version 8.8; Lunar Prodigy, Madison, Wisconsin). A standardized procedure ≥4 hours after a light meal with an empty bladder was used to avoid differences in hydration status.
Magnetic resonance measurements for amounts of subcutaneous adipose tissue, visceral adipose tissue (VAT), and liver fat were performed on a clinical 1.5-T imager (Avanto, Siemens, Erlangen, Germany) with subjects in a supine position head first in the scanner. To allow measurement of abdominal fat distribution, a T1-weighted axial 2-dimensional multislice spoiled gradient echo image stack was centered at the L4/L5 intervertebral disk. Selective fat excitation was used to collect 16 images with 1-cm slice thickness, 0-mm gap, 91-ms repetition time, 5.24-ms echo time, and 80° flip angle.
Point-resolved spectroscopic localization technique with 3,000-ms repetition time, 30-ms echo time, and 16 acquisitions was used to obtain nonsuppressed liver spectra. Orthogonal 3-plane T2-weighted true fast imaging with steady precession images were used for localization of the voxel of interest within the right lobe of the liver. Vascular structures and contaminating signals from subcutaneous fat and the gallbladder were avoided. Voxel size was kept cube shaped but the size varied from 8 to 27 cm 3 because of interindividual differences in the size and shape of liver structures. All magnetic resonance imaging data were collected using a standard body coil. Magnetic resonance spectroscopic data were collected using a flex surface coil in combination with spine coils.
Magnetic resonance images were analyzed using SliceOmatic (TomoVision, Quebec, Canada) 4.3 segmentation software. Areas of subcutaneous adipose tissue and VAT were measured for each slice using a region-growing routine. Results were expressed as total volumes of subcutaneous adipose tissue and VAT.
Liver spectra were analyzed with jMRUI (Claude Bernard University of Lyon, Lyon, France) 3.0 software using the Advanced Magnetic Resonance algorithm. Areas of water signal at 4.7 ppm and methylene signal from intracellular triglycerides at 1.3 ppm were determined using a line-fitting procedure. Spectroscopic intracellular triglyceride content was expressed as methylene/(water + methylene) signal area × 100 and values were further converted to mass fractions as described previously.
Echocardiographic studies were performed using a VIVID 7 instrument (General Electric, GE Healthcare, Milwaukee, Wisconsin) by standard techniques with subjects in the left lateral decubitus position. All echocardiograms were recorded on the hard disk of the instrument. In each 2-dimensional measurement, 10 cycles of parasternal long- and short-axis views and ≥10 cycles of M-mode were recorded with an optimal cursor beam orientation in each view.
Measurement of epicardial fat thickness was conducted using the method presented by Iacobellis et al. A linear epicardial fat thickness was measured on the free wall of the right ventricle from parasternal long- and short axis views, perpendicular to the interventricular septum at the midchordal and tip of the papillary muscle level, as an anatomic landmark. Maximum values in parasternal long- and short axis views were determined, and the average value was considered ( Figure 1 ) . Epicardial fat was not available for 1 obesity-discordant pair for technical reasons.
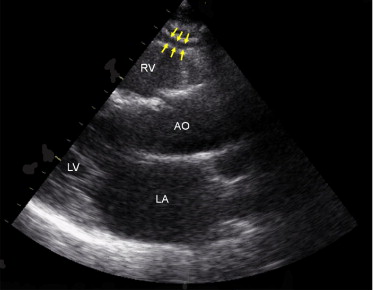
Reproducibility of epicardial fat measurements was determined by the coefficient of variation of triplicate measurements as percent coefficient of variation = (within-subject SD/within-subject mean of 3 epicardial fat measurements) × 100. Coefficients of variation for epicardial fat thickness were 3.6% from the long axis and 3.0% from the short axis. Validity of the epicardial fat measurement was further tested by comparing analyses performed by 2 independent experienced noninvasive cardiologists blinded to each other and to the anthropometric status of the twins. Pearson correlations of 0.999 (long axis) and 0.998 (short axis) and limits of agreement in Bland–Altman comparisons (mean difference between 2 cardiologists 0.024 mm, 95% confidence interval −0.0007 to 0.056, in the long axis; −0.039 mm, 95% confidence interval −0.206 to 0.128, in the short axis) indicated good reproducibility of the epicardial fat measurements.
LV mass was estimated using the anatomically validated formula of Devereux et al. The index that adjusted LV mass was obtained as LV mass/height 2.7 . Measurements of LV end-diastolic diameter and LV systolic ejection fraction were determined according to the American Society of Echocardiography. Relative wall thickness was calculated from the posterior wall thickness and LV end-diastolic diameter as 2 times posterior wall thickness divided by LV end-diastolic diameter. Cardiac output was determined by Doppler echocardiography. LV diastolic function was evaluated using filling and relaxation Doppler echocardiographic parameters: early rapid filling wave, late filling owing to atrial contraction wave, and the early rapid filling wave/late filling owing to atrial contraction wave ratio. In addition, we measured peak early rapid filling wave and late filling owing to atrial contraction wave tissue Doppler velocities by sampling of the mitral annulus.
A standard M-mode left atrial anteroposterior linear dimension was obtained from the parasternal long-axis view in end-systole. Left atrial enlargement was defined as a left atrial diameter >40 mm. Left atrial area was measured in a uniform and standardized manner in all subjects using planimetry from 2-dimensional images in the 4-chamber apical view at end-systole.
Concentrations of serum hs-CRP were measured by a particle-enhanced immunoturbidimetric assay (Cobas CRP HS, Roche Diagnostics, Mannheim, Germany) on a modular automatic analyzer (Hitachi, Ltd., Tokyo, Japan).
Statistical analyses were performed with STATA 11.0 (STATA Corporation, College Station, Texas). Results are expressed as mean ± SE unless stated otherwise. In parametric tests, non-normally distributed data were used after logarithmic transformation. Wald tests for independent samples ( t tests adapted for clustered twin data) and regression models, corrected for clustered data using robust sandwich estimators, were used to compare men and women. Associations among echocardiographic measurements, adiposity, and hs-CRP in individual twins were calculated by Pearson correlations and p values were derived from respective regression analyses adjusted for clustering of twins within pairs.
Comparisons between obese and nonobese cotwins were made by matched-pairs Wilcoxon rank-sum tests. Similarities of cardiac measurements in twin A and twin B were assessed by intraclass correlations with or without adjusting for body height. Within-pair differences of all measurements were calculated by subtracting the leaner cotwin's value from the heavier cotwin's value. Spearman correlations of within-pair differences in all twins were calculated to assess the relations of measurements adjusted for genetic influences. Multiple linear regression models in individual twins (SEs and p values adjusted for twin clustering) and pairs (using within-pair differences) were calculated to determine independent associations among echocardiographic measurements, adiposity, and inflammation (hs-CRP concentration). A p value <0.05 was considered statistically significant.
Results
The mean age of all twins was 28.1 ± 0.7 years (range 22.8 to 33.1). Men and women did not differ in degree of overweight, BMI (28.9 ± 0.9 vs 27.0 ± 1.0 kg/m 2 , p = 0.17), subcutaneous adipose tissue (3,827 ± 490 vs 4,677 ± 366 cm 3 , p = 0.18), or liver fat (2.6 ± 0.6% vs 1.6% ± 0.5%, p = 0.36), but men were expectedly taller and had larger waist circumferences (95.8 ± 2.7 vs 85.9 ± 2.4 cm, p = 0.012), more VAT (1,217 ± 117 vs 686 ± 107 cm 3 , p = 0.0035), and lower percent body fat (29.4 ± 1.8% vs 39.4 ± 2.0%, p = 0.0012). Men also tended to have a thicker epicardial fat pad than women (0.37 ± 0.05 vs 0.28 ± 0.01 cm, p = 0.055). Men had larger LV end-diastolic diameter (52.9 ± 0.9 vs 45.7 ± 1.3 mm, p <0.0001), left atrial diameter (37.4 ± 0.5 vs 33.4 ± 0.9 mm, p = 0.0009), left atrial area (18.7 ± 0.5 vs 16.1 ± 0.7 cm 2 , p = 0.0069), cardiac output (6,844 ± 516 vs 5,565 ± 260 ml/min, p = 0.029), LV mass (216.3 ± 10.0 vs 147.9 ± 9.7 g, p <0.0001), LV mass/height 2.7 (45.0 ± 1.7 vs 37.6 ± 2.1 g/m, p = 0.012), and smaller early rapid filling wave (0.67 ± 0.02 vs 0.85 ± 0.03 m/s, p <0.0001) and early rapid filling wave/late filling owing to atrial contraction wave ratio (1.62 ± 0.07 vs 1.96 ± 0.11, p = 0.017) than women.
Effects of acquired obesity on cardiac measurements independent of genetic influences were examined within MZ pairs. In obesity-discordant MZ pairs, obese cotwins were on average 16.5 kg (23%) heavier than nonobese cotwins and, expectedly, these pairs had significant differences in all adiposity measurements and diastolic blood pressure ( Table 1 ). Obese cotwins had 26% more epicardial fat than their leaner cotwins ( Table 1 , Figure 2 ) . They also had significantly larger left atrial diameter, left atrial area, LV end-diastolic diameter, cardiac output, LV mass, and LV mass/height 2.7 ( Table 1 , Figure 2 ). None of the 15 nonobese cotwins but 4 of the 15 obese cotwins had an enlarged left atrial diameter (>40 mm). LV end-diastolic diameter was >55 mm, the upper limit of normal, in 4 obese cotwins (3 of these also were those who had an enlarged left atrium) and 1 nonobese co-twin. Maximal values of LV end-diastolic diameter were 60.3 mm in obese cotwins and 56.6 mm in nonobese cotwins; these twins belonged to the same twin pair. Although there were some differences in the weights of the concordant twins, these cotwins were similar for all echocardiographic measurements.
Variable | Discordant MZ Twin Pairs | Concordant MZ Twin Pairs | ||||
---|---|---|---|---|---|---|
Nonobese | Obese | p Value ⁎ | Leaner | Heavier | p Value ⁎ | |
Total patients (men/women) | 15 (6/9) | 15 (6/9) | — | 9 (5/4) | 9 (5/4) | — |
Age (years) | 26.8 ± 0.9 | 26.8 ± 0.9 | — | 30.1 ± 0.9 | 30.1 ± 0.9 | — |
Body mass index (kg/m 2 ) | 24.8 ± 1.0 | 30.3 ± 1.0 | <0.001 | 27.6 ± 1.1 | 29.3 ± 1.1 | <0.001 |
Weight (kg) | 75.7 ± 5.0 | 92.2 ± 5.0 | <0.001 | 78.2 ± 4.3 | 83.9 ± 4.2 | <0.001 |
Height (cm) | 173.4 ± 3.0 | 173.6 ± 2.8 | 0.40 | 168.0 ± 2.6 | 168.9 ± 2.3 | 0.43 |
Waist circumference (cm) | 83.4 ± 3.1 | 97.4 ± 3.1 | <0.001 | 88.1 ± 2.9 | 92.8 ± 3.0 | <0.001 |
Subcutaneous adipose tissue (cm 3 ) | 3525 ± 419 | 5733 ± 446 | 0.0007 | 3564 ± 445 | 3870 ± 499 | 0.07 |
Visceral adipose tissue (cm 3 ) | 576 ± 91 | 1165 ± 133 | 0.0010 | 1018 ± 202 | 1033 ± 207 | 0.77 |
Liver fat (%) | 0.8 ± 0.2 | 3.5 ± 0.8 | 0.0038 | 1.3 ± 0.3 | 2.6 ± 1.2 | 0.14 |
High-sensitivity C-reactive protein † (mg/L) | 2.4 ± 0.7 | 4.8 ± 1.9 | 0.0595 | 1.7 ± 0.8 | 1.2 ± 0.3 | 0.51 |
Systolic blood pressure (mm Hg) | 119 ± 3 | 121 ± 3 | 0.43 | 120 ± 3 | 116 ± 2 | 0.14 |
Diastolic blood pressure (mm Hg) | 77 ± 2 | 81 ± 2 | 0.026 | 81 ± 2 | 79 ± 2 | 0.28 |
Heart rate (beats/min) | 72 ± 4 | 76 ± 4 | 0.43 | 70 ± 5.0 | 66 ± 4 | 0.68 |
Epicardial fat † (cm) | 0.29 ± 0.04 | 0.37 ± 0.05 | 0.0029 | 0.31 ± 0.02 | 0.31 ± 0.02 | 0.68 |
Left atrial diameter (mm) | 32.9 ± 1.0 | 36.4 ± 1.1 | 0.0007 | 35.8 ± 1.0 | 36.6 ± 0.7 | 0.24 |
Left atrial area (cm 2 ) | 15.7 ± 0.8 | 18.3 ± 0.8 | 0.0008 | 17.3 ± 0.8 | 18.4 ± 0.7 | 0.14 |
Early rapid filling wave (m/s) | 0.78 ± 0.04 | 0.77 ± 0.04 | 0.86 | 0.14 ± 0.010 | 0.14 ± 0.013 | 0.55 |
Late filling owing to atrial contraction wave (m/s) | 0.44 ± 0.02 | 0.45 ± 0.03 | 0.67 | 0.41 ± 0.03 | 0.47 ± 0.03 | 0.31 |
Early rapid filling wave/late filling owing to atrial contraction wave ratio | 1.85 ± 0.14 | 1.76 ± 0.11 | 0.39 | 1.87 ± 0.13 | 1.71 ± 0.15 | 0.51 |
Peak early rapid filling wave/late filling owing to atrial contraction wave tissue Doppler velocities ratio | 2.41 ± 0.21 | 2.31 ± 0.18 | 0.59 | 2.46 ± 0.31 | 2.43 ± 0.27 | 0.95 |
Left ventricular ejection fraction (%) | 62 ± 2 | 63 ± 2 | 0.69 | 67 ± 3 | 66 ± 2 | 0.95 |
Relative wall thickness | 0.42 ± 0.02 | 0.41 ± 0.02 | 0.46 | 0.42 ± 0.03 | 0.39 ± 0.02 | 0.21 |
Left ventricular end-diastolic diameter (mm) | 48 ± 2 | 50 ± 2 | 0.0015 | 48 ± 2 | 50 ± 1 | 0.37 |
Cardiac output (ml/min) | 5,784 ± 477 | 6,738 ± 458 | 0.0038 | 6,005 ± 492 | 5,931 ± 299 | 0.68 |
Left ventricular mass (g) | 171 ± 14 | 197 ± 17 | 0.0090 | 172 ± 14 | 171 ± 9 | 0.95 |
Left ventricular mass/height 2.7 (g/m) | 38 ± 2 | 43 ± 3 | 0.0157 | 42 ± 3 | 41 ± 2 | 0.81 |
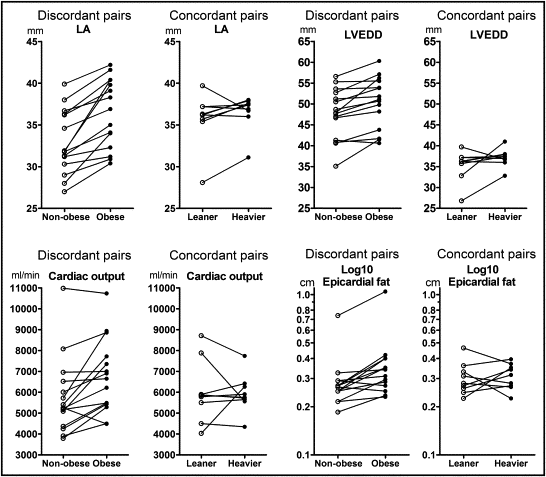
In all twin subjects, epicardial fat was significantly related to waist circumference and VAT but not to measurements of overall fat (BMI), subcutaneous fatness (subcutaneous adipose tissue), or liver fat ( Table 2 , Figure 3 ) . Epicardial fat correlated significantly with left atrial diameter, left atrial area, cardiac output, and LV mass ( Table 2 ). Left atrial diameter, left atrial area, LV end-diastolic diameter, cardiac output, LV mass, and LV mass/height 2.7 correlated very significantly with waist circumference and VAT ( Table 2 , Figure 3 ). Only cardiac output was associated with subcutaneous adipose tissue and liver fat ( Table 2 ).
Variable | Epicardial Fat (cm) ⁎ | BMI (kg/m 2 ) | WC (cm) | AST (cm 3 ) | VAT (cm 3 ) | Liver Fat (%) |
---|---|---|---|---|---|---|
Epicardial fat (cm) | 0.47 | 0.55 † | 0.38 | 0.49 † | 0.36 | |
Left atrial diameter (mm) | 0.42 § | 0.56 § | 0.57 ‡ | 0.21 | 0.43 † | 0.23 |
Left atrial area (cm 2 ) | 0.38 † | 0.53 § | 0.56 § | 0.19 | 0.45 ‡ | 0.22 |
Left ventricular end-diastolic diameter (mm) | 0.36 | 0.44 ‡ | 0.57 ‡ | 0.09 | 0.48 ‡ | 0.23 |
Cardiac output (ml/min) | 0.60 § | 0.57 § | 0.64 § | 0.41 † | 0.50 ‡ | 0.41 † |
Left ventricular mass (g) | 0.31 † | 0.48 ‡ | 0.56 ‡ | 0.10 | 0.44 ‡ | 0.27 |
Left ventricular mass/height 2.7 (g/m) | 0.11 | 0.47 ‡ | 0.47 † | 0.08 | 0.40 ‡ | 0.18 |
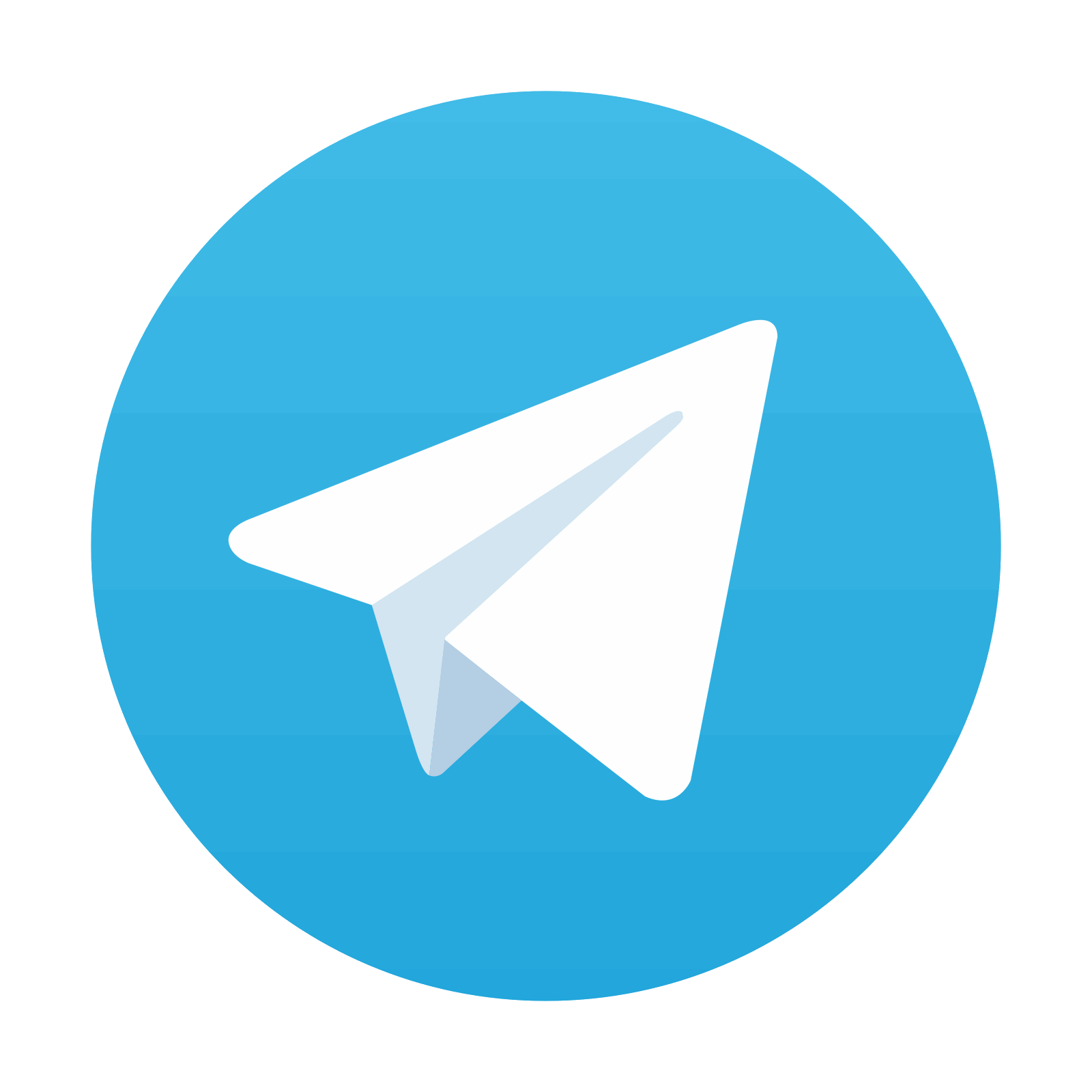
Stay updated, free articles. Join our Telegram channel

Full access? Get Clinical Tree
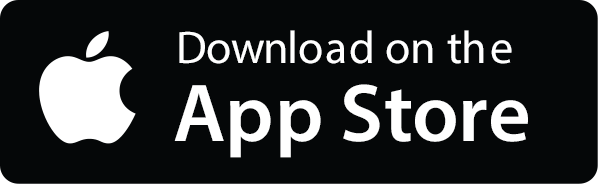
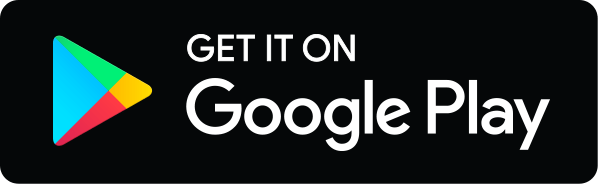
