The effect of brain temperature on reported safe duration of deep hypothermic circulatory arrest.
Cardiopulmonary bypass: re-warming
Re-warming should be instituted following a planned rate of rise of core temperature. Excessively rapid re-warming, accompanied by a rise in cerebral arteriovenous O2 difference, is known to worsen neurological outcome. In patients undergoing coronary artery bypass surgery, maintaining a temperature gradient of < 2°C between inflow temperature and brain (nasopharyngeal) temperature has been shown to improve cognitive outcome. Because hyperthermia is known to exacerbate neuronal injury, inflow temperature should not exceed 37°C and CPB terminated when core body temperature reaches 35.5–36.5°C. A significant “after-drop” is inevitable and patients are commonly admitted to the intensive care unit with temperatures as low as 32°C. Using a slow rate of re-warming with adequate time for even distribution of heat between core and peripheral tissues helps to reduce the extent of this after-drop.
During the period of re-warming attention should be given to the correction of metabolic abnormalities, particularly the metabolic acidosis that inevitably accompanies reperfusion following circulatory arrest. Correction of acid–base balance may require the titrated administration of sodium bicarbonate or use of hemofiltration (ultrafiltration).
Hemostasis
Prolonged CPB and hypothermia produce a coagulopathy. Hemostasis is facilitated by meticulous surgery, the use of predonated autologous blood, and administration of donor blood components under the guidance of laboratory tests of coagulation and thromboelastography. Despite safety concerns, antifibrinolytic agents (e.g. tranexamic acid, ε aminocaproic acid) and aprotinin have been shown to be efficacious in aortic arch surgery with DHCA. Recently published and widely publicized studies reporting a high incidence of adverse effects associated with aprotinin use in adult patients undergoing coronary revascularization and high-risk cardiac surgery have, however, prompted withdrawal of the drug.
Neuroprotection during DHCA
Although hypothermia is the principal neuroprotectant during DHCA, additional strategies may be employed to reduce the likelihood of neurological injury (Table 10.3). These include: acid–base management strategy, hemodilution, leukodepletion, and glycemic control. Surgical maneuvers, such as intermittent cerebral perfusion, selective antegrade cerebral perfusion (SACP), and retrograde cerebral perfusion (RCP), may also be used to both protect the brain and extend the operating time available to the surgeon.
Anesthesia | Glycemic control External cranial cooling Neurological monitoring Cerebrospinal fluid drainage Pharmacological neuroprotection |
Perfusion | Acid–base management strategy Hemodilution Leukocyte depletion |
Surgical | Intermittent cerebral perfusion Selective antegrade cerebral perfusion (SACP) Retrograde cerebral perfusion (RCP) |
Hypothermia
Cerebral metabolism decreases by 6–7% for every 1°C fall in temperature below 37°C, with consciousness and autoregulation being lost at 30°C and 25°C respectively. At temperatures < 20°C, ischemic tolerance is around ten times that at normothermia. While some authors maintain that the electroencephalograph (EEG) becomes isoelectric at this temperature, it is evident that a significant number of patients have measurable EEG activity at < 18°C. In addition to its effects on the metabolic rate, hypothermia appears to reduce lipid peroxidation, neuronal calcium entry, membrane depolarization, the production of superoxide anions, and the release of excitotoxic amino acids.
In many centers, placing ice packs or an ice-cold water jacket around the head after induction of anesthesia is used to augment cerebral cooling. The extent to which extracranial cooling influences brain temperature and neurological outcome in humans remains undocumented. Use of the procedure is justified on the basis of an absence of significant adverse effects and limited evidence derived from animal experimentation (see Figure 10.2).
Hemodilution
The combination of vasoconstriction, increased plasma viscosity, and reduced erythrocyte plasticity secondary to hypothermia leads to impairment of the microcirculation and ischemia. Progressive hemodilution during hypothermic CPB, typically to a hematocrit of 0.18–0.20, is thought to partially alleviate this phenomenon. In some centers, a degree of normovolemic hemodilution is undertaken prior to the onset of CPB. The optimal hematocrit for a particular individual at a specific temperature remains unclear. Gross anemia (i.e. hematocrit < 0.10) may, however, result in inadequate oxygen delivery to tissues, particularly during re-warming.
Acid–base management
Hypothermia increases the solubility of gases (e.g. N2, O2, and CO2) and volatile anesthetic agents in blood. While the total content of any particular gas in a blood sample remains constant, hypothermia shifts the equilibrium between dissolved and undissolved gas, leading to an increase in the former, which in turn reduces the partial pressure of the gas. When analyzed at 37°C, a “normal” blood sample taken during hypothermia reveals ‘normal’ results, whereas correction of these results for body temperature reveals reduced PaO2 and PaCO2, and alkalosis. Maintaining PaCO2 within the normal range on the basis of analysis at 37 °C is termed α-stat management, whereas maintaining a normal PaCO2 (and pH) on the basis of “temperature-corrected” analysis is termed pH-stat management. This is discussed further in Chapter 6.
When cerebral perfusion pressure (CPP), PaO2, and PaCO2 are maintained within the physiological range, autoregulation couples cerebral blood flow (CBF) to cerebral metabolic rate (CMRO2). Cerebral autoregulation is obtunded by profound hypothermia and hypercarbia. At PaCO2 > 10 kPa the classical autoregulation “plateau” is abolished and CBF becomes ‘pressure-passive’ – dictated solely by CPP. Alpha-stat management preserves cerebral autoregulation and thus CBF decreases during hypothermia. In contrast pH-stat management results in cerebral hyperperfusion, which in turn increases O2 delivery and ensures more rapid and homogeneous brain cooling, albeit at the expense of increased cerebral microembolic load.
In the piglet model of DHCA, pH-stat management improves neurological outcome. In human neonates undergoing DHCA for repair of congenital heart defects, pH-stat management prior to circulatory arrest appears to be associated with fewer complications than alpha-stat management and better developmental outcome. In adults, however, the superiority of one strategy over another in the setting of DHCA remains unproven. Currently α-stat management is used in most adult centers – presumably on the basis of superior cognitive outcome following hypothermic CPB.
Retrograde cerebral perfusion (RCP)
Reversing the direction of blood flow in the superior vena cava (SVC) has been advocated as a means of improving brain protection during DHCA and extended the period of “safe” DHCA. Following the onset of CPB, the cavae are snared and arterial blood directed into the SVC via an arteriovenous shunt constructed in the CPB circuit. Pump flows of 150–700 ml min−1 are advocated to maintain a mean perfusion pressure of ~ 25 mmHg. The putative advantages of RCP include continuous cerebral cooling, cerebral substrate delivery, and expulsion of air, particulates, and toxic metabolites. The absence of blood flow detectable by transcranial Doppler (TCD) sonography in the middle cerebral arteries of a small, but significant, number of patients subjected to RCP may explain conflicting evidence of efficacy. In addition, significant extracranial shunting via the external jugular veins may occur during RCP. Interestingly, the use of multi-modal neurological monitoring to guide RCP delivery at pressures as high as 40 mmHg, considered by many surgeons to be harmful, has been shown to be safe.
Antegrade cerebral perfusion (ACP)
Selective hypothermic brain perfusion permits surgery to be conducted at lesser degrees of systemic hypothermia (e.g. 22–25°C); reducing the time required for cooling and re-warming, and thus the total duration of CPB. ACP typically requires greater mobilization of the epiaortic vessels and division of the innominate vein. Following the onset of circulatory arrest, the aortic arch is opened and balloon-tipped arterial cannulae advanced into the innominate and left carotid artery ostia (see Figure 10.3). The left subclavian artery is clamped and arterial flow commenced at 10–20 ml/kg to maintain a perfusion pressure – measured in the right radial artery – of 50–70 mmHg. Alternative approaches include cannulation of the right subclavian artery and hemicerebral perfusion via the innominate artery alone. The technique provides more “physiological” cerebral perfusion but has the disadvantage of increasing operative time and carries the risk of atheroembolism and microembolism. In a recently published series of 501 consecutive patients undergoing aortic arch surgery with DHCA (25°C) and ACP (14°C), Khaladj et al. reported an overall mortality of 11.6% and permanent neurological deficit rate of 9.6%.
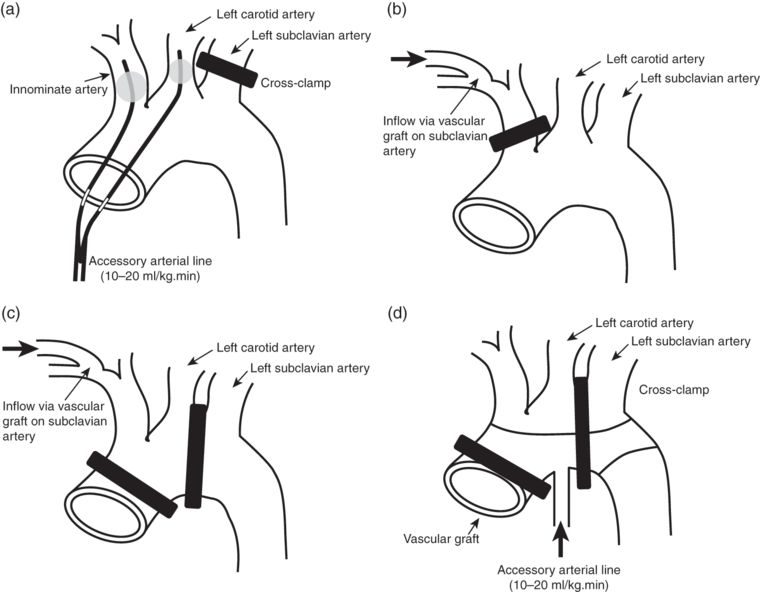
Examples of selective antegrade cerebral perfusion techniques. (a) Direct cannulation of the innominate and left carotid arteries. (b) Hemicranial perfusion via a left subclavian artery graft. (c) Bilateral cranial perfusion via a left subclavian artery graft. (d) Bilateral cranial perfusion via a sidearm on vascular graft.
In some instances intermittent, rather than continuous, ACP is considered more practicable or expeditious. In a piglet model of DHCA, one minute of reperfusion for every 15 minutes of DHCA has been found to be sufficient to provide normal metabolic and microscopic cerebral recovery.
In a retrospective, two-center study of patients undergoing “non-complex” aortic arch reconstruction, Milewski et al. compared the efficacy of RCP and ACP. Patients undergoing DHCA with RCP in Philadelphia, USA (n=682) were compared with patients undergoing moderate hypothermic circulatory arrest (MHCA) with bilateral ACP in Bologna, Italy (n=94). As expected, cerebral ischemic times were significantly longer in the DHCA/RCP group and visceral ischemic times significantly longer in the MHCA/ACP group. There were no significant differences in either the primary (death, neurological deficit) or secondary (new renal failure, acute myocardial infarction, prolonged mechanical ventilation, reoperation for bleeding) outcomes. The authors concluded that both techniques afforded excellent organ protection for aortic reconstruction of < 45 minutes’ duration.
Leukocyte depletion
The use of leukocyte-depleting arterial line filters is reported to moderate the systemic inflammatory response to CPB, reduce reperfusion injury, and reduce postoperative infective complications. Evidence for cerebral protection by leukocyte depletion is lacking in humans, and animal experimentation has yielded conflicting results. Interestingly, perfusate modification in a piglet model of DHCA has been shown to improve neurological outcome. Furthermore, the removal of leukocytes and calcium, and the addition of magnesium, O2, CO2, mannitol, and a Na+/H+ exchange inhibitor to the CPB prime during circulatory arrest were found to improve myocardial function.
Glycemic control
Insulin resistance and hyperglycemia are common consequences of cardiac surgery and hypothermia. In animal models, hyperglycemia worsens outcome after cerebral infarction. While tight glycemic control during cardiac surgery appears to reduce mortality and infective complications, any neuroprotective effect remains unproven and carries the risk of inadvertent hypoglycemia and hypokalemia.
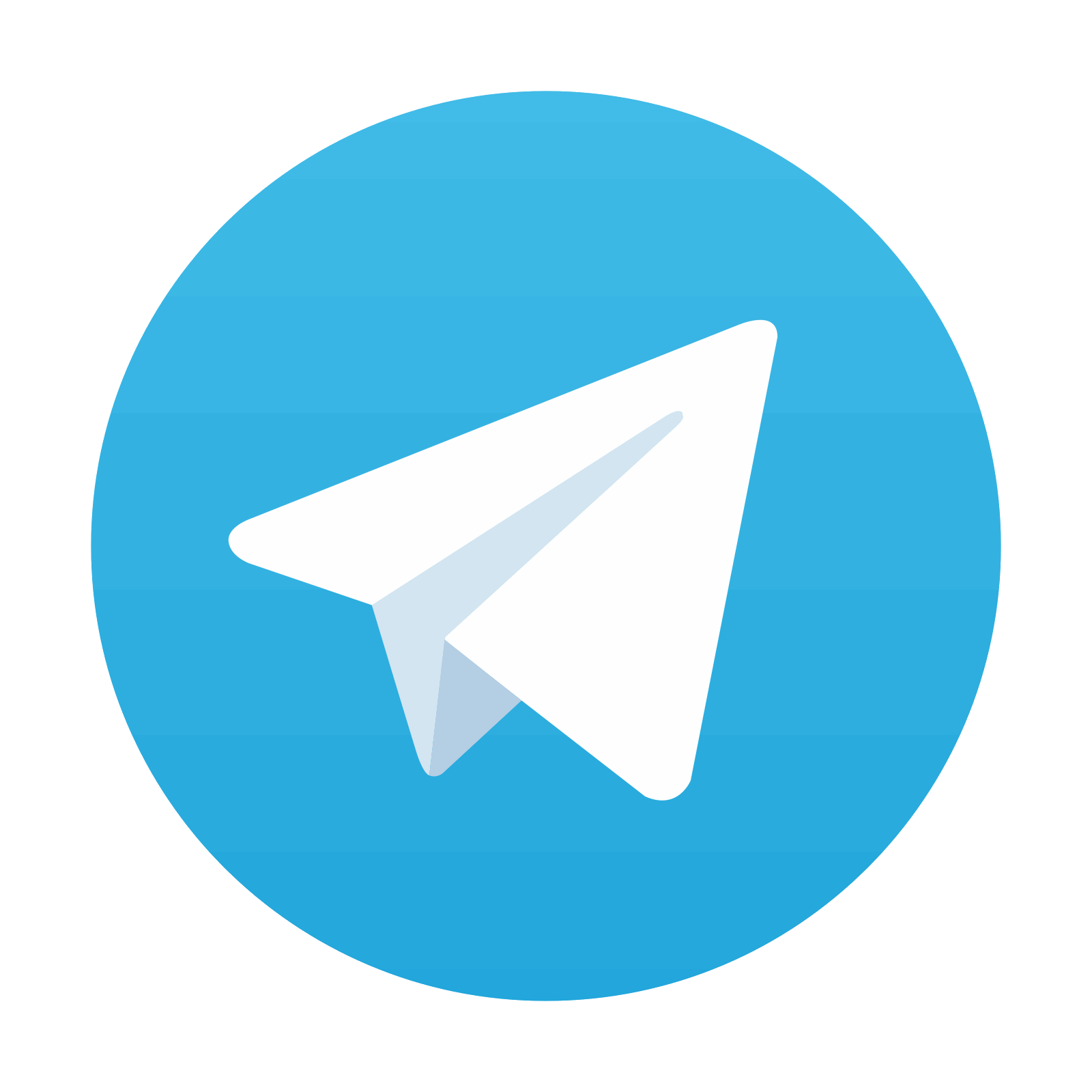
Stay updated, free articles. Join our Telegram channel

Full access? Get Clinical Tree
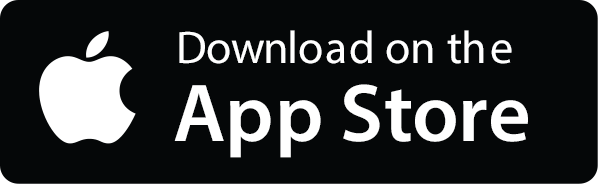
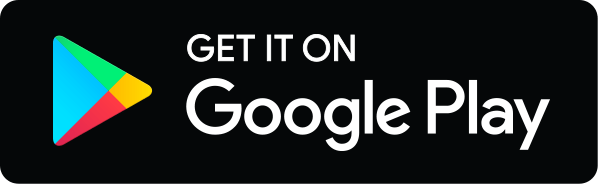