Definition
Perhaps the most widely accepted general definition of the term cor pulmonale remains the one articulated in 1963 by an expert committee appointed by the director general of the World Health Organization (WHO): Chronic cor pulmonale is “hypertrophy of the right ventricle resulting from diseases affecting the function and/or structure of the lung, except when these pulmonary alterations are the result of diseases that primarily affect the left side of the heart or congenital heart disease.”1 In the updated clinical classification of pulmonary hypertension, cor pulmonale can occur due to conditions included in the third (pulmonary hypertension due to lung diseases and/or hypoxia) and fourth categories (pulmonary hypertension due to chronic thrombotic and/or embolic disease).2 Of note, acute dilatation of the right ventricle of the heart, that is, acute cor pulmonale, is a disorder in which the right ventricle is dilated and the muscular wall is stretched thin. This is most often the result of massive pulmonary embolism. The chronic form of the disorder, the principal subject of this chapter, is characterized by right ventricular (RV) hypertrophy with eventual dilatation and right-sided heart failure.
The right ventricle is ill suited to excessive mechanical demands, being adapted to pump blood through the normally low-resistance, high-capacitance lung circulatory bed. The final common pathophysiologic event that causes chronic cor pulmonale is chronically increased resistance to blood flow through the pulmonary circulation leading to pulmonary arterial hypertension (PAH). Unlike systemic hypertension, PAH is difficult to diagnose clinically, so pulmonary vascular pressures may be elevated for prolonged periods before the disorder is recognized. In fact, dysfunction of the right ventricle (ie, cor pulmonale) is often recognized as the initial clinical diagnosis in patients with either primary or secondary PAH. Newer noninvasive techniques can provide more information about pulmonary circulatory function than in the past. However, suspecting PAH early in its course still depends largely on the skill of the experienced clinician.
Incidence
Estimates of the incidence of chronic cor pulmonale, as well as estimates of morbidity and even mortality directly attributable to right-heart dysfunction secondary to lung disease, are difficult to obtain. It is difficult to separate epidemiologic data relevant to cor pulmonale from the lung disease that is its primary cause. In addition, a definitive diagnosis most often requires invasive diagnostic procedures, often at a time when treating the lung disease is the most paramount clinical issue. However, the magnitude of the clinical problem can be appreciated from data on chronic obstructive pulmonary disease (COPD), undoubtedly the most common cause of cor pulmonale.
An estimated 24 million US adults have COPD, and it is the third leading cause of death.3,4 In the United States, >125,000 deaths are attributable to COPD every year. The health care costs and resources associated with COPD are tremendous. Every year, there are >700,00 hospitalizations for COPD exacerbations and related illnesses.3 Worldwide, the problem is even more dramatic, with hundreds of millions of people suffering from COPD.5
Due to varying definitions, diagnostic tests, and patient populations, the prevalence of pulmonary hypertension in patients with COPD is unclear (Table 73–1).6 Although the incidence of severe pulmonary hypertension purely attributable to COPD seems to be the exception (<5%) rather than the rule, the presence of mild pulmonary hypertension is relatively common.7 Furthermore, the fraction of patients with right-sided heart dysfunction resulting from secondary PAH is not established, although in one study, RV dysfunction was found in more than two thirds of patients with end-stage lung disease referred for lung transplantation evaluation.8
Reference | Cohort Characteristics | Diagnostic Modality | No. of Patients | FEV1 | PaO2 | Proportion With Pulmonary Hypertension |
---|---|---|---|---|---|---|
Himelman et al, Am J Med., 1988 | Nonhypoxemia cohort | Echocardiography | 33 | 1.0 L | 70.9 mm Hg | 75% with cor pulmonale |
Oswald-Mammosser et al, Chest., 1995 | Emphysema cohort | Right heart catheterization | 84 | 0.85 L | 52 mm Hg | 65 (77%) of 84 patients with mPAP >20 mm Hg; 31 (37%) of 84 patients with mPAP >30 mm Hg |
Bach et al, Chest., 1998 | LVRS evaluation | Echocardiography | 206/207a | 27.3% pred | 67.0 mm Hg | 40.1%b |
Right heart catheterization | 92 | NA | NA | Resting mPAP >35 mm Hg in 5.4% | ||
Chaouat et al, Am J Respir Crit Care Med., 2005 | Chronic respiratory failure | Right heart catheterization | 998 | 50% pred | 46 mm Hg | Resting mPAP >40 mm Hg in 2.7%; 11 patients had COPD as primary cause (1.1%) |
Christensen et al, Eur Respir J., 2004 | General cohort | Right heart catheterization with exercise | 17 | 35% pred | 10.4 kPa | mPAP with exercise >30 mm Hg in 65% |
Scharf et al, Am J Respir Crit Care., 2002 | Emphysema cohort evaluated for LVRS | Right heart catheterization | 120 | 27.0% pred | 65.9 mm Hg | Resting mPAP >20 mm Hg in 90.8%; resting mPAP >35 mm Hg in 5.0% |
Doi et al, Respir Med., 2003 | Emphysema cohort | Right heart catheterization | 53 | 39.8% pred | 70.9 mm Hg | mPAP ≥2.7 mPa in 43% |
Kessler et al, Am J Respir Crit Care Med., 2001 | Mild to moderate hypoxemia cohort | Right heart catheterization | 131 | 44.6% pred | 67.0 mm Hg | Resting mPAP >20 mm Hg in none; exercise mPAP >30 mm Hg in 58%; mean of 6.8 y later, resting mPAP >20 mm Hg in 25% |
Thabut et al, Chest., 2005 | LVRS or LT evaluation | Right heart catheterization | 215 | 18.5% pred LT; 27.0% pred LVRS | 55.4 mm Hg in LT; 66.2 mm Hg in LVRS | Resting mPAP >25 mm Hg in 50.2%; resting mPAP 35-45 mm Hg in 9.8%; resting mPAP >45 mm Hg in 3.7% |
Etiologies
Diseases that affect lung structure and function can increase pulmonary vascular resistance either directly or indirectly through effects on the lungs’ gas exchange function. Any cause of persistent PAH of sufficient magnitude and duration can cause cor pulmonale. Although classifications can be somewhat arbitrary and there can be enormous overlap, pathophysiologic mechanisms and disease entities that increase pulmonary vascular resistance and contribute to the development of cor pulmonale are listed in Table 73–2.
Principal Pathophysiologic Mechanism | Disease Entity |
---|---|
Persistent vasoconstriction | High-altitude dwellers |
Hypoventilation syndromes | |
Chest deformities | |
? Idiopathic pulmonary hypertension | |
Loss of cross-sectional area of the vascular bed | Thromboembolic disease Emphysema |
Lung resection | |
Fibrotic lung diseases | |
Cystic fibrosis | |
Obstruction of large vessels | Extrinsic compression of pulmonary veins |
Fibrosing mediastinitis | |
Adenopathy/tumors | |
Pulmonary veno-occlusive disease | |
Chronically increased blood flow | Eisenmenger syndrome |
Vascular remodeling | Primary pulmonary hypertension |
Secondary pulmonary hypertension | |
Collagen vascular diseases | |
Cystic fibrosis |
Pathophysiology of Increased Pulmonary Vascular Resistance
The lung has the daunting task of removing carbon dioxide from and reoxygenating all of the blood destined for the systemic circulation. For the lung to perform its principal function of gas exchange efficiently, it must not only maintain the integrity of the vast gas exchange surface that brings blood into intimate contact with air, but must also match the amount of blood perfusing with the amount of air ventilating each gas exchange unit. Hypoxic pulmonary vasoconstriction is an adaptive vasomotor response to alveolar hypoxia, which redistributes blood from poorly oxygenated areas to better ventilated lung segments, thereby optimizing ventilation-perfusion matching, reducing shunt fraction, and optimizing systemic oxygen delivery by an active process of vasoconstriction.9 This matching of ventilation and perfusion is accomplished primarily by the unique property of pulmonary vessels to constrict when exposed to a hypoxic environment (systemic vessels dilate in response to hypoxia). The mechanism responsible for transducing hypoxic pulmonary vasoconstriction remains unclear but may involve local generation of reactive oxygen species that affect smooth muscle ion channels. Figure 73–1 is a schematic representation of proposed pathways involved in hypoxic pulmonary vasoconstriction.9 Because laminar flow of blood through tubes is governed by the Poiseuille law (ie, resistance to flow is inversely proportional to vessel radius to the fourth power), small changes in resistance vessel diameter can have major effects on perfusion.
Figure 73–1.
Proposed schematic representation of pathways involved in hypoxic pulmonary vasoconstriction. The redox theory proposes that the proximal mitochondrial electron transport chain (ETC) senses hypoxia and decreases reactive oxygen species (ROS) production. A decrease in superoxide radical or H2O2 in turn inhibits effector O2-sensitive Kv channels. Kv channel inhibition depolarizes the membrane and activates Ca2+ entry via L-type Ca2+ channels. Other theories propose nicotinamide adenine dinucleotide phosphate (NADPH) oxidase as a sensor, cyclic adenosine diphosphate ribose (cADPR) as a redox-activated mediator that causes intracellular Ca 2+ release by activating ryanodine receptors, and Rho-kinase, which sensitizes the contractile apparatus to Ca2+. EM, membrane potential; MnSOD, manganese superoxide dismutase; SR, sarcoplasmic reticulum. Reproduced with permission from Moudgil R, Michelakis /ED, Archer SL. Hypoxic pulmonary vasoconstriction. J Appl Physiol. 2005;98:390-403.
Viewed teleologically, hypoxic pulmonary vasoconstriction must be a local phenomenon. To optimize gas exchange, blood flow needs to be selectively reduced to areas of lung that are poorly ventilated (and thus hypoxic). Because the normal pulmonary vascular bed has a very large capacitance and a reserve of capacity to exchange carbon dioxide and oxygen, blood shunted away from poorly ventilated areas will be shunted to well-ventilated areas and thus contribute arterialized blood to the systemic blood supply with essentially no increase in overall pulmonary vascular resistance in the normal lung. The diagram in Fig. 73–2 illustrates this phenomenon.10
Figure 73–2.
Adaptation of blood flow to ventilation in the pulmonary circulation. Blood flow (Q) in the pulmonary circulation must, ideally, be directed to well-ventilated areas (symbolized by the V [ventilation] and O2 [oxygenation] in the largest alveolus [blue circle at bottom of figure]) to ensure optimized gas exchange (“matching”), whereas only a small amount of blood should flow through areas of minor or no ventilation (midsize and small alveoli, respectively) (left panel). Reproduced with permission from Ghofrani HA, Osterloh IH, Grimminger F. Sildenafil: from angina to erectile dysfunction to pulmonary hypertension and beyond. Nat Rev Drug Discov. 2006;5:689-702.
But what if all (or a very large fraction) of the lung is hypoxic? In that case, there will be global constriction of the resistance vessels in the lung circulation with an obligatory increase in pulmonary vascular resistance and PAH. The purest clinical example of this is the persistent PAH (and cor pulmonale) that develops in people who are living at a high enough altitude so that ambient air is deficient in oxygen because of the low barometric pressure.11 Alveolar hypoventilation, resulting either from decreased central ventilatory drive or from physical distortions of the lung relative to the ventilatory apparatus because of deformities of the chest, can also result in hypoxia. If hypoxia is sufficiently extensive, the normal hypoxic vasoconstrictive response will result in PAH. That chronic hypoventilation can result in cor pulmonale is graphically illustrated in drawings of the “fat boy” in Charles Dickens’ The Pickwick Papers, who is generally said to have suffered from obesity-hypoventilation (thus pickwickian) syndrome. The peripheral edema characteristic of right-sided heart failure is evident in both Dickens’ elegant description and in the classic illustration. The current epidemic of obesity in the United States makes recognition of this entity especially important and may well result in an increasing incidence of the syndrome.12
Resistance to blood flow through the pulmonary vasculature depends on the radius of individual resistance vessels and on the total cross-sectional area of the vascular bed. If the area of the vascular bed is sufficiently decreased, then PAH will develop.
Although it is clear that resistance to flow should increase if the total amount of vascular bed is decreased, the relationship for the lungs is not linear, at least in situations where the remaining lung vessels are intrinsically normal. The large capacitance of the normal lung vascular bed allows large reductions in the amount of the bed that is perfused without an increase in resistance. The clearest example of the direct effects of reducing the size of the vascular bed is with lung resection.13 The total lung mass can be decreased by half before there is any increase in pulmonary artery pressure. That observation is consistent with clinical experience with lung resection in humans. If the unresected lung is essentially normal, a total pneumonectomy does not result in PAH at rest. Once the capacitance of the pulmonary vascular bed is exceeded, there is a steep relation between pulmonary artery pressure and the amount of vascular bed. Following pneumonectomy in humans, PAH on exercise may occur because of increased pulmonary blood flow in a vascular bed with little remaining capacitance.14
In the most common clinical settings in which there is loss of lung vessels, the remaining perfused vessels are either functionally or structurally abnormal. For example, destruction of lung parenchyma with COPD reduces the area of the vascular bed, but there may be vasoconstriction in the perfused bed as a result of an abnormal ventilatory pattern. Pulmonary emboli directly obstruct lung vessels, but even patent vessels may be constricted because of release of humoral mediators. In addition, chronic pulmonary embolic disease can result in structural remodeling of the pulmonary vascular bed with irreversible PAH and cor pulmonale.15
Pulmonary artery pressure in patients with COPD increases with exercise even though resting pulmonary artery pressure may be normal. Pressure during exercise is a steep function of resting pressure, although exercise increased pressure even in subjects with normal resting pressures, indicating loss of pulmonary vascular capacitance. In the compromised vascular bed, hemodynamic reserve is lost so that physiologic changes such as local hypoxic vasoconstriction or increased pulmonary blood flow, which are easily accommodated in the normal lung circulation, result in increased afterload to the right ventricle, the proximate cause of cor pulmonale.
The most common cause of acute cor pulmonale is occlusion of the large proximal pulmonary artery by a massive embolus. However, large pulmonary vessels may also be compromised more insidiously by disorders of the mediastinum.
Direct compression of either pulmonary arteries or veins can result from enlarging mediastinal lymph nodes or mediastinal tumors. In addition, the rare but devastating disorder, fibrosing mediastinitis, can enmesh large pulmonary arteries or veins (or bronchi) in an inexorably advancing mass of connective tissue with progressive occlusion.16 Occlusion of the main pulmonary artery imposes a direct resistance against which the right ventricle must pump, analogous physiologically to stenosis of the pulmonary valve or hypertrophic stenosis of the pulmonary outflow tract of the right ventricle. If progression of the obstruction is sufficiently slow, chronic cor pulmonale can be a principal clinical picture in the presence of normal lungs. Obstruction of pulmonary veins will also elevate overall pulmonary vascular resistance, but in this case, the lung microvasculature will bear the brunt of the pressure increase. The consequences for the pulmonary circulation and the right side of the heart are analogous to those of either left-sided heart failure or mitral valve stenosis. Depending on the chronicity and pace of the obstruction, cor pulmonale may result.
Although the definition of cor pulmonale given earlier excludes congenital heart disease, chronically increased flow through the pulmonary circulation results in functional and structural alterations in the pulmonary vascular bed, and these changes in the lung come to dominate the pathophysiology. This clinical situation develops most often as a result of an abnormal shunting of blood from the left side of the circulation back to the right side, bypassing the systemic circulation (eg, septal defects). To maintain blood supply to systemic organs, the right side of the heart must pump a larger volume of blood per unit time (the shunted blood in addition to the systemic venous return).
Increased flow through pulmonary vessels causes remodeling of the lung circulation and progressive PAH. The remodeling process involves apparently direct effects of increased flow on expression of several factors that alter the structure of the vessels. Studies in animal models with chronically increased pulmonary blood flow demonstrate increased expression of vascular endothelial growth factor and its receptors, transforming growth factor β, and the cell growth promoters tenascin-C and matrix metalloproteinase in muscular vessels.17,18 Increased linear shear stress imposed on cultured pulmonary vascular endothelial cells induces cytoskeleton remodeling and other endothelial cell alterations.19 Endothelial-dependent pulmonary vascular relaxation is also compromised in experimentally induced increased pulmonary blood flow,20 so that loss of normal homeostatic mechanisms for maintaining low pulmonary vascular resistance may contribute to the development of persistent PAH in this circumstance.
The ultimate result of persistent increased pulmonary blood flow and the worsening PAH is reversal of flow through the shunt. As pulmonary vascular resistance increases, right-sided heart pressures increase, finally reaching levels approaching systemic pressures, reversing the pressure gradient and forcing blood to flow through the shunt from the right side of the circulation to the left. Clinically, this condition is termed Eisenmenger complex.21 Figure 73–3 is the chest x-ray of a patient with RV enlargement and severe pulmonary hypertension due to an unrepaired secundum atrial septal defect. Although patients with this syndrome appear to tolerate high right-sided pressures better than patients with PAH from other causes, eventually right-sided heart failure, cor pulmonale, occurs.
Figure 73–3.
Posteroanterior and lateral chest x-ray of a 40-year-old man with Eisenmenger syndrome due to an unrepaired secundum atrial septal defect. The salient features are the right ventricular hypertrophy (loss of retrosternal space) and prominent pulmonary arteries (severe pulmonary hypertension).
A low baseline resistance to blood flow, a large vascular capacitance, and the ability to create flow heterogeneities in order to match perfusion to ventilation are all essential to normal function of the lung circulation. Structural remodeling of the pulmonary arterial circulation that occurs in several lung diseases resulting in PAH compromises each of these essential characteristics.
The clearest example of vascular remodeling in the lungs is the unusual disorder idiopathic PAH (IPAH; formerly primary PAH), which occurs as both a familial, inherited disorder and a sporadic disorder. The etiology of this progressive fatal disease remains unknown, although an inherited abnormality in the gene encoding bone morphogenic protein receptor-2 (a member of the transforming growth factor β receptor superfamily) is apparently responsible for the disease in at least some families.22 Exactly how this genetic predisposition fits into the pathogenesis of PAH is not yet clear. Remodeling also occurs in secondary PAH with functional consequences such as those in the primary form of the disease. For example, pulmonary complications of collagen vascular diseases include remodeling of the lung vasculature23; vascular remodeling is a feature of COPD as well.24
Much has been learned about how remodeling of the pulmonary arterial circulation occurs from studies in animals in which pulmonary vascular resistance is elevated chronically by various experimental interventions. Remodeling is a complex process that, anatomically, includes hypertrophy of smooth muscle in arterial resistance vessels, extension of vascular smooth muscle peripherally to previously nonmuscular arteries, and loss of microvascular bed resulting from intimal proliferation.25 These structural changes act in concert to increase resistance to blood flow through the lung circulation. In addition, in some forms of persistent PAH, there is a tonic vasoconstrictive component that compounds the problem.26
Response of the Right Cardiac Ventricle to Increased Pulmonary Vascular Resistance
Mechanical demands on the right ventricle under normal conditions are minimal compared with demands on the left ventricle because the work required to pump blood through the low-resistance, low-impedance lung circulation is a fraction of that required to perfuse the high-pressure systemic circulation. The structure of the right ventricle compared to the left ventricle reflects the difference in their physiologic requirements.27 The left ventricle is symmetrical with a thick muscular wall. In contrast, the RV wall is much thinner, and the cavity is crescent shaped because the convexity of the septum bulges toward the right (Fig. 73–4). Both the geometry of the two ventricles and the mass of their muscular walls reflect their different functions. The symmetrical, thick-walled left ventricle is suited to generating high-outflow pressures, whereas the thin-walled, asymmetric right ventricle is not.
Figure 73–4.
A cross-section of a gross specimen of a normal heart illustrating a thick-walled, spherical left ventricle contrasted with a thin-walled, crescent-shaped right ventricle. Reproduced with permission from Rappaport E. Cor pulmonale. In: Murray J, Nadel J, eds. Textbook of Respiratory Medicine. 3rd ed. Philadelphia, PA: W.B. Saunders; 2000:1635.
Figure 73–5 compares the responses of the normal right and left ventricles to increased afterload in an experimental preparation.28
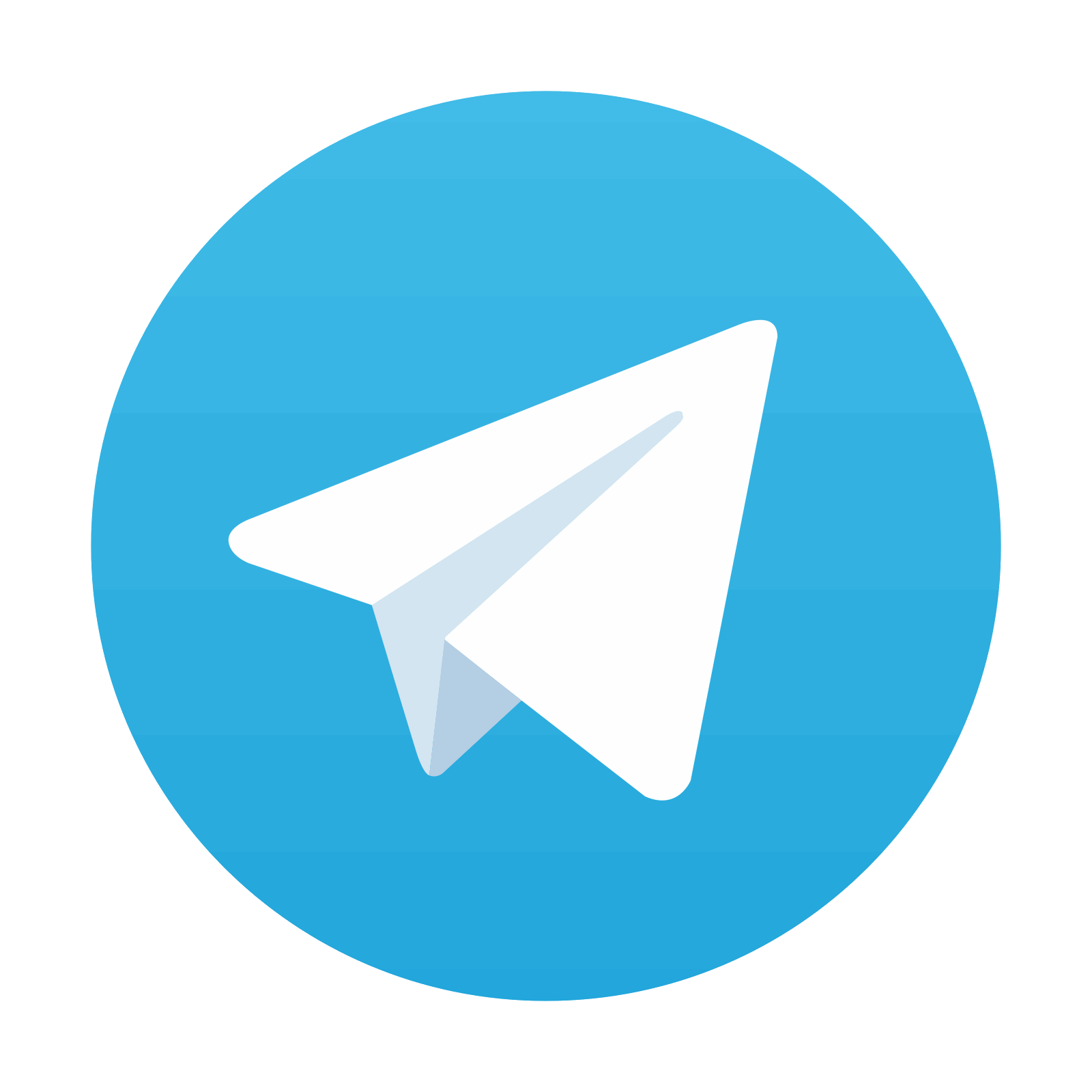
Stay updated, free articles. Join our Telegram channel

Full access? Get Clinical Tree
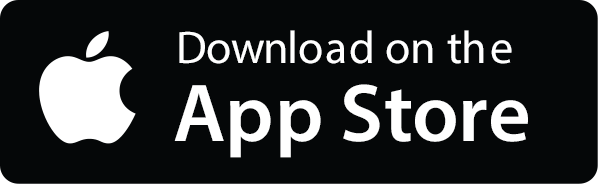
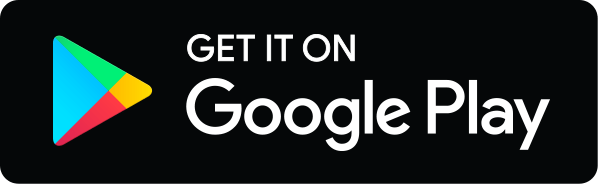
