Bradyarrhythmias
Bradyarrhythmias are most commonly caused by failure of impulse formation (sinus node dysfunction) or by failure of impulse conduction over the atrioventricular (AV) node/His-Purkinje system. Bradyarrhythmias may be caused by disease processes that directly alter the structural and functional integrity of the sinus node, atria, AV node, and His-Purkinje system or by extrinsic factors (autonomic disturbances, drugs, etc.) without causing structural abnormalities (Table 43–1).
Sinus node dysfunction
|
AV conduction abnormalities
|
Bundle branch block
|
Normal electrical activation of the heart arises from the principal pacemaker cells that spontaneously depolarize, located laterally in the epicardial groove of the sulcus terminalis,1 near the junction of the right atrium and the superior vena cava (Fig. 43–1). The sinus node in adults measures approximately 1 to 2 cm long and 0.5 mm wide. The central zone of the sinus node containing the principal pacemaker cells is small and located within a fibrous tissue matrix. In the periphery of the node along the crista terminalis, transitional cells with pacemaker function are also present. Experimental and clinical evidence now suggest that the sinus node region is less defined than previously appreciated. The principal pacemaker site within this region may migrate, resulting in subtle alterations in P-wave morphology. Once the impulse exits the sinus node and the perinodal tissues, it traverses the atrium to the AV node. The conduction of impulses from right to left atrium has been postulated to occur preferentially via Bachmann bundle, and secondarily across the musculature of the coronary sinus.
Once the sinus node impulse activates the atrium, electrical activation continues through the AV node, with a conduction delay ensuring complete atrial contraction before initiation of ventricular conduction. The AV nodal complex is considered to have three related regions: (1) the transitional cell zone, (2) the compact AV node, and (3) the penetrating AV bundle.2 The transitional zone consists of the main atrial approaches to the compact AV node. The compact AV node is shaped like a half oval and is located beneath the right atrial endocardium at the apex of the triangle of Koch. The triangle of Koch is formed by the base of the septal leaflet of the tricuspid valve and the tendon of Todaro (formed by the extension of the eustachian valve into the central fibrous body). The coronary sinus ostium is located at the base of this triangle. The distal end of the compact AV node enters the central fibrous body to become the penetrating bundle and continues in the membranous septum as the bundle of His. The speed of conduction through the AV nodal complex is at 0.03 m/s, whereas the His-Purkinje fibers conduct at 2.4 m/s.
The penetrating part of the AV bundle continues through the annulus fibrosis into the membranous septum, along the crest of the left side of the interventricular septum for 1 to 2 cm, and then divides into the right and left bundle branches. The right bundle branch continues intramyocardially along the right side of the interventricular septum and emerges subendocardially beneath the anterior papillary muscle of the right ventricle. The left bundle begins as a sheet of fascicles and runs along the left side of the inter-ventricular septum and soon separates into anterior and posterior sheets (most anatomists agree it is a trifascicular system with a substantial septal branch) corresponding to the papillary muscles. In many hearts, the left bundle may appear more as a network rather than a well-defined bifascicular system. The terminal Purkinje fibers arising from the bundle branches form interweaving networks on the endocardial surface of both the right and left ventricles. The rapid conduction of electrical impulses across this network results in near simultaneous activation of both right and left ventricles.
The sinus node receives its blood supply from the sinoatrial (SA) nodal artery arising from the right coronary artery in 59% of patients, from the left circumflex artery in 38%, and from both arteries with a dual blood supply in 3%. The AV node is supplied by the AV nodal artery arising from the right coronary artery in 90% of patients, whereas the left circumflex artery provides it in the remaining 10% of patients. The bundle of His is supplied by both the AV nodal artery and branches of the left anterior descending artery. The left bundle has a rich blood supply from the AV nodal artery, posterior descending artery, and branches of the left anterior descending artery.3
The conduction system of the heart is significantly influenced by both the parasympathetic and sympathetic nervous system. The sinus node is richly innervated with postganglionic adrenergic and cholinergic nerve terminals. Vagal stimulation slows the sinus node discharge rate and increases the intranodal conduction time, occasionally to the point of sinus node exit block. Adrenergic stimulation increases the sinus node discharge rate. Parasympathetic tone predominates at rest in healthy individuals, and parasympathetic withdrawal occurs with increased sympathetic discharge during exercise and emotion. The effects of sympathetic and parasympathetic stimulation on the AV node are more pronounced than they are on the His-Purkinje system. Although sympathetic stimulation shortens AV-nodal conduction time and refractoriness, vagal stimulation prolongs AV-nodal conduction time and refractoriness. Both sympathetic and vagal stimulation have minimal effect on normal conduction in the His bundle.
Sinus node dysfunction is a common clinical syndrome, comprising a wide range of electrophysiologic abnormalities from failure of impulse generation, failure of impulse transmission to the atria, inadequate subsidiary pacemaker activity, and increased susceptibility to atrial tachyarrhythmias.4 This disorder has also been variably termed the sick sinus syndrome,tachycardia-bradycardia syndrome,SA disease, and SA dysfunction.
Disorders of sinus node dysfunction may be caused by intrinsic (processes that directly affect the anatomy and physiology of the sinus node and/or the surrounding atrial tissue) or extrinsic factors (processes that affect sinus node function in the absence of structural abnormalities) (Table 43–2). However, in some patients a combination of intrinsic and extrinsic factors may be responsible for sinus node dysfunction. In patients with sinus node dysfunction, histopathologic evaluation has revealed the following patterns5: significant loss of nodal cells with replacement fibrosis, amyloid deposition in the nodal region, hypoplastic or atrophic sinus node, and no detectable morphologic abnormality.
Intrinsic | Extrinsic |
---|---|
Idiopathic degenerative disorder Ischemic heart disease
Hypertensive heart disease Cardiomyopathy Trauma Surgery for congenital heart disease
Inflammation
Infection
Neuromuscular disorder
Familial disorder | Drugs
Cardiac glycosides Antihypertensive agents
Antipsychotic agents
Autonomically mediated
Hypothyroidism Intracranial hypertension Hypothermia Hyperkalemia Hypoxia |
Although an idiopathic degenerative disorder of the sinus node is the most common cause for intrinsic sinus node dysfunction, ischemic heart disease is responsible in a significant number of patients. Chronic ischemia from sinus node artery disease or acute myocardial infarction (MI), especially inferior wall MI, may result in sinus bradycardia, sinus arrest, and atrial tachyarrhythmias. Other potential causes include long-standing hypertension, cardiomyopathy (especially infiltrative disorders such as amyloidosis and sarcoidosis), inflammation, collagen vascular disease, and inherited neuromuscular disorders. In some cases, the condition appears to be familial in origin.6 Mutations in the cardiac sodium channel gene, SCN5A, have been reported in families to result in primary cardiac conduction disease. The mutations that are associated with conduction system disease result in reduced Na+ current. The spectrum of these conduction diseases varies widely, but includes sinus node dysfunction, atrial standstill, AV block, and infra-Hisian block. Rare cases of sinus node dysfunction requiring pacemaker therapy have been reported with Lyme disease (Borrelia burgdorferi infection).7 In children and young adults, damage to the sinus node during atrial surgery (closure of atrial septal defects of the sinus-venosus type, mustard procedure for transposition of great arteries) has been commonly associated with sinus node dysfunction. Sinus node dysfunction is also not uncommon in the donor hearts of patients with orthotopic cardiac transplantation.
The most important causes of sinus node dysfunction in patients without structural abnormalities are drugs and autonomic nervous system influences (see Table 43–2). Drugs may alter sinus node function by direct pharmacologic effects on nodal tissue or indirectly by neurally mediated effects.8 Antiarrhythmic drugs used to maintain sinus rhythm in patients with atrial fibrillation may cause significant sinus node dysfunction, especially in patients with underlying sinus node dysfunction. Other causes for sinus node dysfunction include electrolyte abnormalities, such as hyperkalemia, hypothermia, intracranial hypertension, hypoxia, hypercapnia, and hypothyroidism.
A variety of electrocardiographic (ECG) abnormalities have been described in patients with sinus node dysfunction. Many of the ECG abnormalities that define sinus node dysfunction may be asymptomatic, and these abnormalities by themselves do not warrant therapy.
Sinus bradycardia is defined as a sinus rate <60 beats/min. In most instances sinus bradycardia is a benign arrhythmia. In healthy young adults and trained athletes, resting sinus bradycardia may be a normal phenomenon caused by increased vagal tone. Sinus bradycardia during sleep in elderly individuals is common. Inability to increase sinus rates adequately during exercise is considered abnormal (see below). Sinus bradycardia <40 beats/min (not associated with sleep or physical conditioning) is generally considered abnormal. Correlation of symptoms with sinus bradycardia is critical in the evaluation of these patients.
Sinus pause or arrest means failure of sinus node discharge with lack of atrial activation of sinus origin. This results in absence of P waves and periods of ventricular asystole if lower pacemakers (junctional or ventricular) do not initiate escape beats (Fig. 43–2). The resulting pause in sinus activity should not be in multiples of preceding sinus cycle length (P-P interval). Asymptomatic sinus pauses of up to 3 seconds in duration are not uncommon in trained athletes.8 Pauses longer than 3 seconds need careful clinical correlation with symptoms and warrant further evaluation.
In SA exit block, as the name implies, the impulse is formed in the sinus node but fails to conduct to the atria, unlike sinus arrest. This particular arrhythmia is recognized on ECG by pauses resulting from the absence of normal P waves and the duration of the pause, measuring an exact multiple of the preceding P-P interval (Fig. 43–3). SA block can also be described in the same way as AV block. In first-degree SA block, there is significant prolongation of the time for the sinus impulse to exit into the atria (SA conduction time). This cannot be identified clinically or electrocardiographically. Similar to AV block, second-degree SA block can be type I (Wenckebach) or type II. In type I there is progressive prolongation of SA conduction, manifested on surface ECG as progressive shortening of P-P interval, before the pause created by loss of a P wave. In type II SA exit block, the P-P intervals remain constant before the pause. Third-degree or complete SA block will manifest as absence of P waves, with long pauses resulting in lower pacemaker escape rhythm; it is impossible to diagnose with certainty without invasive sinus node recordings.
Sinus bradycardia interspersed with periods of atrial tachyarrhythmias is a common manifestation of sinus node dysfunction. The atrial tachyarrhythmias usually range from paroxysmal atrial tachycardia to atrial flutter and atrial fibrillation. Apart from underlying sinus bradycardia of varying severity, these patients often experience prolonged sinus arrest and asystole upon termination of the atrial tachyarrhythmias, resulting from suppression of sinus node and secondary pacemakers (Fig. 43–4). Long sinus pauses that occur after electrical cardioversion of atrial fibrillation are another manifestation of sinus node dysfunction. Therapeutic strategies to control tachyarrhythmias often result in the need for pacemaker therapy. These patients are at increased risk for thromboembolism,9 and the issue of long-term anticoagulation should be addressed to prevent strokes.
Chronic atrial fibrillation with a slow ventricular response in the absence of AV nodal-blocking drugs may also be a manifestation of sinus node dysfunction. These patients may demonstrate very slow ventricular rates at rest or during sleep and occasionally have long pauses. They may also conduct rapidly and develop symptoms caused by tachycardia during exercise. Occasionally they may develop complete AV block with junctional or ventricular escape rhythms.
Atrial standstill is a rare clinical syndrome in which there is no spontaneous atrial activity and the atria cannot be electrically stimulated.10 The surface ECG usually reveals junctional bradycardia without atrial activity. This must be differentiated from fine atrial fibrillation with complete heart block. Intracardiac electrograms fail to show any atrial activity. Atria are generally fibrotic and without any functional myocardium. Myocarditis, amyloidosis, and familial etiology have been recognized as causes in some cases. Lack of mechanical atrial contraction poses a high risk for thromboembolism in these patients.
Chronotropic incompetence is the inability of the sinus node to achieve at least 80% of the age-predicted heart rate. It is present in approximately 20% to 60% of patients with sinus node dysfunction.11 Although the resting heart rates may be normal, these patients may have either the inability to increase their heart rate during exercise or have unpredictable fluctuations in heart rate during activity. Some patients may initially experience a normal increase in heart rate with exercise, which then plateaus or decreases inappropriately. Chronotropic incompetence may be secondary to intrinsic sinus node dysfunction or secondary to drugs with negative chronotropic effects. Quantitative evaluation of the heart rate response to exercise may be utilized to characterize the severity of this abnormality using a number of specific exercise protocols, including the Naughton, Balke, Modified Astrand, Ellestead, and chronotropic assessment exercise protocol (CAEP).
Even though sinus node dysfunction may occur in any age group, more than half of the patients affected are older than 50 years of age at the time of diagnosis. The incidence of sinus node dysfunction is approximately equal in both men and women. Patients with sinus node dysfunction commonly present with symptoms of syncope, near syncope, or dizzy spells, predominantly related to prolonged sinus pauses. Patients with sinus bradycardia or chronotropic incompetence, however, may present with relatively nonspecifc symptoms, such as decreased exercise capacity, recurrent falls, or fatigue. Patients with atrial fibrillation may also present with palpitations or congestive heart failure. Elderly individuals may have unexplained confusion or memory loss. Occasionally, stroke may be the first manifestation of sinus node dysfunction in patients presenting with paroxysmal atrial fibrillation and thromboembolism.
The diagnosis of sinus node dysfunction in patients who present with typical symptoms and ECG findings is straightforward. However, because of the intermittent nature of the symptoms and rhythm manifestations, the diagnosis can be time-consuming and frustrating. A number of noninvasive and invasive tests are available to assist in the evaluation.
Documentation of the various arrhythmias associated with sinus node dysfunction can be obtained with routine telemetric monitoring during hospitalization or with outpatient ambulatory 24- to 48-hour Holter recordings. If the symptoms are infrequent in nature, event recorders capable of intermittent or continuous monitoring can be used. Newer monitoring systems use remote real-time monitoring of patients’ rhythm using wireless cellular technology as well as physician-defined triggers. If other noninvasive and invasive tests are inconclusive, patients may benefit from an implantable loop recorder, which has the ability to record both patient-triggered and automatic device-triggered events over a period of 18 to 24 months. Exercise testing to assess chronotropic incompetence can be valuable in select patients. Many patients with sinus node dysfunction may achieve peak heart rates similar to matched controls during specific exercise protocols; however, the time course of heart rate acceleration during activity and deceleration after activity may be markedly abnormal.
Abnormalities of autonomic control of sinus node alone or in association with intrinsic sinus node disease can result in clinical symptoms and electrocardiographic findings of sinus node dysfunction. This can be assessed by observing the response of heart rate and rhythm with carotid sinus massage, head-up tilt testing, and Valsalva maneuver. Pharmacologic evaluation of the sinus node can be performed with atropine, isoproterenol, and propranolol. After injection of atropine 0.04 mg/kg intravenously, the heart rate increases by 15% and to more than 90 beats/min. Isoproterenol infusion at 1 to 3 μg/min increases heart rate by 25%. Not all normal patients will respond as described above. Patients with sinus node dysfunction show blunted heart rate responses to the preceding infusions. Another method to assess sinus node function is to measure the intrinsic heart rate (IHR) of the sinus node when the autonomic influence is negated by both atropine (0.04 mg/kg) and propranolol (0.2 mg/kg), using the following equation12:
IHR = 117.2 − (0.53 × age) beats/min
Patients with sinus node dysfunction often demonstrate a decreased intrinsic heart rate.
Invasive electrophysiology study, in addition to assessing sinus node function, offers insight into other potential etiologies for symptoms of syncope and palpitations (AV block, supraventricular tachycardia, ventricular tachycardia [VT]). The sinus node recovery time is a measure of sinus node automaticity and is measured as the longest pause after atrial overdrive pacing. SA conduction time is a measure of the interval from sinus node depolarization to activation of atrial muscle. With improved modalities for monitoring, it is less common to require electrophysiology studies to make this diagnosis.
Theophylline and β-adrenergic agonists have been used to treat symptomatic bradycardia. Although they have been shown to increase the heart rate and reduce the duration of sinus pauses, they do not prevent recurrent syncope.13 In patients with extrinsic causes for sinus node dysfunction, treatment should be directed toward the underlying etiology. Drugs known to depress sinus node function can be switched to other agents that lack effects on the cardiac conduction system. This is not possible, however, in many patients. Patients with paroxysmal atrial tachyarrhythmias either require drugs to maintain sinus rhythm or agents to control ventricular rate, both of which may have significant sinus node depressant effects. In many of these patients, pacemaker therapy becomes essential. At the time of diagnosis of sinus node dysfunction, the incidence of atrial fibrillation is reported to be approximately 8%, and the likelihood of developing new atrial fibrillation in this group of patients is approximately 5% per year.23 The prevalence of thromboembolism was reported to be 15.2% in this group. This underscores the importance of monitoring for atrial fibrillation and the need for oral anticoagulation in intermediate- to high-risk patients.
The current indications for pacing in the setting of sinus node dysfunction are shown in Table 43–3. In this and subsequent tables, the format used for American Heart Association (AHA)/American College of Cardiology (ACC) guidelines is used, with class I to III indications denoting the degree of agreement for a given procedure or treatment that is useful and effective.
Class I
|
Class IIa
|
Class IIb
|
Class III
|
Sinus node dysfunction is the most common indication for permanent pacemaker implantation in North America, accounting for more than 42% to 60% of new pacemaker implants. The optimal pacemaker choice is influenced by a number of factors. At the time of diagnosis of sinus node dysfunction, 17% of patients have been reported to have AV conduction abnormalities in the form of a PR interval >240 ms, bundle-branch block, H-V interval prolongation, AV Wenckebach rates <120 beats/min, and second- or third-degree AV block. The incidence of new AV block developing over time was reported to be <2.7% per year.15 Generally, if the patient has persistent atrial fibrillation, a single-chamber ventricular pacemaker (VVIR) is recommended. In all other situations, a rate-responsive dual-chamber pacemaker (DDDR) is used, whereas a single-chamber atrial pacemaker (AAIR) can be implanted if no evidence of AV conduction disease is present.
Many nonprospective, nonrandomized studies have suggested significant survival benefits with physiologic pacing compared with ventricular demand pacing (VVI mode) in patients with sinus node dysfunction. This led to randomized, large-scale prospective trials, the results of which are summarized in Table 43–4. The first randomized trial comparing atrial with ventricular pacing in 225 patients with sick sinus syndrome demonstrated significant reduction in the incidence of atrial fibrillation, thromboembolism, and cardiovascular mortality in the atrial pacing group.16 Subsequent large-scale trials have not confirmed these mortality or stroke prevention benefits. The Canadian Trial of Physiologic Pacing (CTOPP)17 randomized patients with symptomatic bradycardia without chronic atrial fibrillation to receive either a physiologic (atrial or dual-chamber) pacemaker or a ventricular pacemaker and showed no difference in stroke or cardiovascular mortality, but a decreased annual rate of atrial fibrillation in the physiologic pacing group (5.3%) compared with the ventricular pacing group (6.6%). Another large randomized trial of dual-chamber pacing versus ventricular pacing was MOST (Mode Selection Trial in sinus node dysfunction),18 which also confirmed the benefit of dual-chamber pacing to reduce the risk for atrial fibrillation. The United Kingdom Pacing and Cardiovascular Events (UKPACE) trial compared dual chamber pacing with single-chamber ventricular pacing in patients older than 70 years of age with high-grade atrioventricular block.19 Over a mean follow-up period of 4.6 years, there was no difference in annual mortality, atrial fibrillation, or heart failure between the groups. In a recent meta-analysis of randomized pacing modes, atrial-based pacing was associated with a significant reduction in atrial fibrillation of approximately 20% (95% confidence interval [CI], 0.77-0.89; P = .00003) and a borderline significant reduction in the risk of stroke of 19% (95% CI., 0.67-0.99; P = .035). The use of atrial-based pacing did not reduce mortality or the incidence of congestive heart failure.20
Trial | Indication | Mode comparison | No. of patients | Follow-up | Endpoints | Results |
---|---|---|---|---|---|---|
Danish16 | SSS | AAI vs VVI | 225 | 8 y | Total mortality, AF, thromboembolism | Atrial pacing significantly reduced mortality (RR 0.66), AF (RR 0.54), and stroke (RR 0.47) |
PASE25 | SSS and AVB | DDDR vs VVIR | 427 | 30 mo | Primary: QOL Secondary: Total mortality, stroke, AF, HF | No change in QOL, mortality, stroke or AF, improved QOL in SSS patients with physiologic pacing |
CTOPP17 | SSS and AVB | DDDR/AAIR vs VVIR | 2568 | 3 y | Primary: Stroke, CV mortality Secondary: Total mortality, AF, hospitalization for HF | No reduction in mortality or stroke; relative risk reduction of 18% for AF with physiologic pacing |
MOST18 | SSS | DDDR vs VVIR | 2010 | 33 mo | Primary: Total mortality, stroke Secondary: Composite of death, stroke, or hospital for HF, AF, QOL, HF score | No difference in primary or secondary end point except for AF; lower incidence of AF with DDD pacing (hazard ratio of 0.79) |
UKPACE19 | AVB | DDDR vs VVIR | 2021 | 4.6 y | Primary: Total mortality Secondary: HF, AF, composite of stroke, TIA, or thromboembolic events | No difference in primary (mortality 7.4% vs 7.2%) or secondary end points |
SAVE PACe23 | SSS | DDD vs DDD with minimal ventricular pacing | 1065 | 1.8 y | Primary: Time to persistent AF Secondary: HF, mortality | 42% relative risk reduction; 4.8% absolute risk reduction in AF No difference in mortality or HF |
The failure to easily demonstrate clinical benefits of dual-chamber pacing has forced a rethinking of physiologic pacing. It is now well accepted that long-term right ventricular (RV) pacing causes a deterioration of left ventricular (LV) function through complex effects on regional ventricular wall strain and loading conditions. This deterioration is thought to result from intraventricular dyssynchrony between different regions of the left ventricle induced by RV apical pacing. Sweeney and coworkers demonstrated, by careful review of data from MOST, that an increase in the frequency of ventricular pacing in patients with sick sinus syndrome who had a narrow native QRS complex was associated with an increased incidence of atrial fibrillation and congestive heart failure.21 These observations were confirmed by Wilkoff and colleagues in the Dual Chamber and VVI Implantable Defibrillator (DAVID) study, in which backup ventricular pacing and dual-chamber pacing were prospectively compared in patients with dual-chamber defibrillators.22 The primary end point was a composite of congestive heart failure, hospitalization, and death, which was increased by a factor of 1.6 in patients with an increased frequency of ventricular pacing. Thus RV pacing is a double-edged sword, conferring atrioventricular synchrony but at the same time possibly negating its benefit by inducing ventricular dysfunction. Sweeney et al23 randomized 1065 patients with sinus node dysfunction and intact ventricular conduction to receive conventional dual-chamber pacing versus or dual-chamber minimal ventricular pacing with the use of new pacemaker features designed to promote atrioventricular conduction and prevent ventricular desynchronization. The hazard ratio for development of persistent atrial fibrillation in patients with dual-chamber minimal ventricular pacing as compared with those with conventional dual-chamber pacing was 0.60 (95% CI, 0.41-0.88; P = .009), indicating a 42% reduction in relative risk. We recommend that patients with sinus node dysfunction have the pacemaker programmed to either AAI(R) or DDD(R) with an algorithm that minimizes unnecessary ventricular pacing.
The pathophysiology, diagnosis, and specific management of these disorders are discussed separately in Chap. 48. A hypersensitive response to carotid sinus stimulation of 5 to 10 seconds is defined as asystole caused by sinus arrest or AV block of more than 3 seconds (cardio-inhibitory), a substantial symptomatic decrease in systolic blood pressure of 50 mm Hg or more, (vasodepressor) or both (mixed). The pathophysiology of carotid sinus syncope is complex and poorly understood and is hypothesized to result from abnormalities of neuromuscular structures surrounding the carotid sinus mechanoreceptors, a central defect of the autonomic nervous system, and association with atherosclerotic disease. Carotid sinus syncope predominantly occurs in elderly males with associated coronary artery disease. Although carotid sinus hypersensitivity is common, carotid sinus syncope is relatively rare, accounting for 11% to 19% of patients with syncope of undetermined cause. For patients with recurrent or severe carotid sinus syncope of the cardioinhibitory type, permanent pacemaker implantation is the accepted and proven modality of treatment as evidenced by many small randomized trials.24 DDI or DDD pacemakers are generally the accepted mode of pacing for patients with carotid sinus syncope, whereas AAI pacing is contraindicated because many patients may demonstrate associated AV block. The current indications for pacing in hypersensitive carotid sinus syndrome and neurocardiogenic syncope are shown in Table 43–5.
Class I
|
Class IIa
|
Class IIb
|
Class III
|
Vasovagal syncope is a common condition caused by inappropriate reflex vasodilation and bradycardia and occasionally even asystole. Dual-chamber pacemakers with rate-drop response or rate hysteresis features are currently a treatment option in a select group of patients with recurrent syncope and refractory to conventional medical therapy. Three randomized but non–placebo-controlled trials have assessed the role of permanent pacemakers in patients with vasovagal syncope and cardioinhibitory response during tilt-table testing (Table 43–6).26-28 The results of these trials are somewhat contradictory, and subsequent randomized, controlled trials29,30 did not show a significant reduction in recurrent syncope. As a result, we recommend pacing therapy as a last resort for patients who are severely symptomatic and refractory to medical therapy.
Trial | Inclusion Criteria | Treatment Groups | No. of Patients | Follow-up | End Points | Results |
---|---|---|---|---|---|---|
VPS26 | Syncope × 6, positive tilt-table test with relative bradycardia | DDD pacer with RDR vs no pacer | 54 | 15 mo | First recurrence of syncope | 85% relative risk reduction for recurrent syncope (22% in pacemaker vs 70% in no pacemaker arm) |
VASIS27 | >3 syncope in last 2 y, positive tilt-table test with HR <42 or asystole <3 s, age >42 y | DDI pacer with rate hysteresis vs no pacer | 42 | 3.7 y (mean) | First recurrence of syncope | Significant reduction in recurrent syncope (5 % in pacemaker arm vs 61% in no pacer arm) |
SYDAT28 | >3 syncope in last 2 y, positive tilt-table test with relative bradycardia, age >35 y | DDD pacer with RDR vs atenolol | 93 | 17 mo (mean) | First recurrence of syncope | Significant reduction in recurrent syncope, 4.3% in pacer group vs 25.5% in atenolol group |
VPS II29 | >5 syncope/lifetime or >2 syncope/2 y, positive tilt-table test with relative bradycardia, age >19 y | DDD pacer with RDR vs pacer in ODO mode | 100 | 6 mo | First recurrence of syncope | Nonsignificant reduction in recurrent syncope (DDD-paced patients 30% vs 42% in ODO) |
SYNPACE30 | >6 syncope/lifetime, positive tit-table test | DDD with RDR vs OOO | 29 | 715 d (median) | First recurrence of syncope | No difference |
AV block occurs when atrial conduction to the ventricle is blocked at a time when the AV junction is not physiologically refractory. This can be caused by conduction block in the atrium, AV node, and/or His-Purkinje system. Using His-bundle electrogram recordings, three anatomic sites of AV block can be identified: AV nodal, intra-Hisian, or infra-Hisian. Block at the AV nodal level implies a favorable prognosis, whereas block at or below the His-bundle level implies an unfavorable prognosis. Surface ECGs often provide adequate information to make a diagnosis regarding the site of the block, whereas occasionally intracardiac recordings are necessary to confirm the level of the block.
Transient or persistent AV block of varying degrees can occur in a variety of clinical situations (Table 43–7). Heightened vagal tone in athletes or during sleep may be associated with first-degree or type I second-degree AV block and occasionally even complete heart block. The AV block in these situations is usually preceded by slowing of the heart rate. Vagally mediated AV block may occur in response to various stimuli such as carotid sinus hypersensitivity, coughing, swallowing, or micturition. Varying degrees of heart block have been described in a large variety of infectious diseases. Heart block associated with endocarditis may be transient or permanent. In patients with endocarditis and ring abscess, heart block may not resolve and pacing may be required. With Lyme disease, cardiac involvement may occur in 8% to 10% of patients. More than 50% of patients with cardiac involvement may develop advanced heart block requiring temporary pacing.31 Even complete AV block generally resolves in 1 to 2 weeks, and permanent pacing is seldom necessary. Chagas cardiomyopathy may be associated with persistent AV block.
Reversible | Permanent |
---|---|
Physiologic
Autonomic mediated
Coronary artery disease
Infectious disease
Myocarditis
Metabolic
Traumatic
Drug induced
| Idiopathic fibrosis
Lenègre disease
Congenital heart disease
Infiltrative disease Amyloidosis
Endocarditis
Systemic lupus erythematosus
Traumatic
Tumors
Neuromuscular disease
|
Idiopathic progressive fibrosis of the conduction system is a common cause of acquired AV block. Also known as idiopathic bilateral bundle-branch fibrosis, Lev disease is characterized by progressive replacement of the proximal bundle branches by fibrosis as a result of the aging process exaggerated by hypertension and arteriosclerosis. Lenègre disease is a variant of idiopathic conduction disorder involving young patients and the peripheral parts of the bundle branches. Some cases of Lenègre disease may be caused by mutations in the sodium channel gene SCN5a, causing a hereditary form of AV conduction disease.32
AV block occurs in 12% to 25% of all patients with acute MI; first-degree AV block occurs in 2% to 12%, second-degree AV block in 3% to 10%; and third-degree AV block in 3% to 7%.33 Ischemic injury can produce conduction block at any level of the AV or intraventricular conduction system. Second-degree type I AV block occurs more commonly in inferior than anterior MIs. Inferior infarctions are often associated early on with increased vagal tone causing sinus bradycardia and AV block. Most patients are asymptomatic, and rarely type I AV block may progress to complete AV block. Second-degree type II AV block occurs in 1% of patients with acute MI and predominantly occurs in patients with anterior infarctions. The risk of progression to complete AV block is high in these patients. Complete AV block can occur in both inferior and anterior infarction. In inferior MI, the block is typically at the level of the AV node and the escape rhythm generally arises from the AV junction, with a narrow QRS and an escape rate of 40 to 60 beats/ min. The prognosis in these patients is generally good as the AV block resolves in almost all patients within a few days and almost always by 1 week. Complete AV block resulting from anterior infarction is usually at the His or infra-Hisian level, and the escape rhythm is from the distal Purkinje fibers or the ventricle, with a wide QRS interval and a rate of 20 to 40 beats/min. Because of the coexisting extensive infarction and pump failure, AV block resulting from anterior infarctions are associated with high mortality. In patients with acute MI, temporary pacing is generally indicated in patients with complete AV block at any level, type II second-degree AV block, and in patients with type I second-degree AV block if associated with symptomatic bradycardia. Although the incidence of complete AV block in acute MIs has decreased after thrombolytic therapy, the mortality still remains high. The indications for permanent pacing in patients with acute MI are listed in Table 43–8. Patients with coronary artery disease and ischemic cardiomyopathy may develop persistent AV block. AV nodal block can occur transiently during episodes of ischemia, especially with Prinzmetal angina.
Class I
|
Class IIb
|
Class III
|
A variety of uncommon autoimmune, oncologic, infectious, and iatrogenic disorders can also lead to heart block and are listed in Table 43–7. Certain neuromuscular disorders (myotonic dystrophy, Kearns-Sayre syndrome, peroneal muscular atrophy, Erb limb-girdle dystrophy, Emory-Dreifuss muscular dystrophy, and X-linked muscular dystrophies) may give rise to progressive and insidiously developing conduction disorders of the His-Purkinje system. Myotonic dystrophy and Kearns-Sayre syndrome are associated with high incidence of unpredictable and rapidly progressive conduction system disease.34 Complete heart block may occur after aortic or mitral valve replacement surgery and rarely after coronary artery bypass surgery. Preoperative right bundle-branch block and multivalve surgery involving the tricuspid valve were shown to be strong independent predictors of postoperative heart block requiring permanent pacemaker implantation. Complete AV block is more common after surgical procedures to correct ventricular septal defects, tetralogy of Fallot, AV canal defects, or myectomy for hypertrophic obstructive cardiomyopathy. A variety of inherited heart disease has been recently reported to be associated with dilated cardiomyopathy and conduction system disease, including disorders of the nuclear envelope proteins lamin A and C.35 Congenital heart diseases such as corrected transposition of great arteries, ostium primum atrial septal defects, and ventricular septal defects may be associated with complete heart block. Congenital complete AV block is a rare anomaly that results from abnormal embryonic development of the AV node and is not associated with structural heart disease in 50% of cases. Congenital complete heart block is also associated with maternal lupus erythematosus. Most children with isolated congenital complete AV block have a stable escape rhythm with a narrow complex. Pacing is generally indicated in children with complete heart block if the heart rate in the awake child is <50 beats/min or if associated with LV systolic dysfunction or ventricular arrhythmias. The indications for pacing in children, adolescents, and patients with congenital heart disease are outlined in Table 43–9. Patients with conduction system disease and evidence of cardiac or sarcoidosis in other organs should undergo implantation of either a dual-chamber pacemaker or defibrillator.
Class I
|
Class IIa
|
Class IIb
|
Class III
|
Prolongation of the PR interval to >200 ms constitutes first-degree AV block. It is most commonly caused by conduction delay within the AV node and occasionally caused by intra-atrial or infra-Hisian conduction delay. If the QRS duration is normal, the site of conduction delay is almost always within the AV node. If first-degree AV block occurs in the presence of bundle-branch block, the conduction delay can be in the AV node (60% of cases), His-Purkinje system, or both. Patients with first-degree AV block have an excellent prognosis even when associated with chronic bifascicular block, as the rate of progression to third-degree AV block is low, and no specific therapy is indicated.
Second-degree AV block is characterized by intermittent failure of conduction from the atria to the ventricles. If the AV block occurs with the atrial rate in the physiologic range, it is considered a primary arrhythmia. AV block in the setting of atrial tachyarrhythmias is generally a normal response. Based on the electrocardiographic patterns, second-degree AV block is classified into Mobitz types I and II.
Second-degree AV block of the Wenckebach type (Mobitz type I) is characterized by the following features: (1) progressive prolongation of the PR interval before a nonconducted P wave, (2) PR interval prolongation at progressively decreasing increments, (3) progressive shortening of R-R intervals, (4) pause encompassing the blocked P wave shorter than the sum of two P-P cycles, and (5) the last conducted PR interval before the blocked P wave longer than the next conducted PR interval (Fig. 43–5). Type I second-degree AV block often occurs with regularity leading to patterns of group beating. When type I second-degree AV block occurs in association with a normal QRS interval, block is almost always in the AV node. In the presence of a prolonged QRS interval, block may be in the AV node, His-Purkinje (rare), or both. Very long PR intervals are usually caused by a block in the AV node. Type I second-degree AV block occurs in a small percentage of normal people and not uncommonly in well-trained athletes. Most patients are asymptomatic, whereas some develop symptomatic bradycardia, near syncope, or occasionally syncope caused by progression to complete AV block.
Figure 43–5
Type I second-degree atrioventricular (AV) block. A 6:5 AV Wenckebach periodicity is shown. Note that the PR interval progressively lengthens with a decreasing increment. This results in shortening of R-R intervals. The last conducted PR interval (0.33 seconds) is significantly longer than the next conducted PR interval (0.24 seconds).
Mobitz type II second-degree AV block is characterized by (1) constant P-P intervals and R-R intervals; (2) constant PR intervals before a nonconducted P wave; and (3) pause encompassing the nonconducted P wave equal to two P-P cycles. Type II AV block usually occurs in the presence of bundle-branch block and is almost always caused by a block in the His-Purkinje system (Fig. 43–6). Type II AV block rarely occurs in patients with normal QRS duration. Type II AV block frequently progresses to a complete AV block and may result in syncopal attacks.
Figure 43–6
Type II second-degree atrioventricular block. Surface electrocardiogram (ECG), leads I, aVF, and V1, and intracardiac electrograms from the right atrial (RA), proximal, and distal His bundle electrogram (HBE) catheters are shown. Surface ECGs show that the PR intervals are constant at 0.2 seconds with a left bundle-branch block morphology of the QRS complex, and the fourth P wave is not followed by a QRS complex. The HBEs reveal that the site of block of the fourth P wave is below the His bundle.
In this form of second-degree AV block, every other P wave is not conducted, making it difficult to diagnose the level of AV block (Fig. 43–7). A 2:1 AV block pattern with normal QRS duration or with a very long PR interval generally suggests block in the AV node. A 2:1 AV block pattern in the presence of bundle-branch block favors block below the AV node, but is not diagnostic. A prolonged electrocardiographic recording may sometimes reveal a transition to varying degrees of AV block (3:2 or 4:3), with type I or type II features that aids in the diagnosis. Rarely, Wenckebach conduction may be noted in the His-Purkinje system. Intracardiac recordings with a His-bundle catheter are sometimes necessary to determine the site of the block. In patients with a 2:1 AV block, vagal maneuvers are helpful in diagnosing the level of AV block. Carotid sinus stimulation may worsen the degree of block if it is in the AV node, whereas slowing of the sinus rate may paradoxically improve the ratio of AV conduction and increase ventricular rate if the block is located in the His-Purkinje system. Similarly, atropine improves AV nodal conduction, but the increased sinus rate may worsen the ratio of AV conduction in patients with His-Purkinje block, resulting in worsened bradycardia. Hence atropine should be used with caution in patients with a 2:1 AV block and bundle-branch block where His-Purkinje disease is strongly suspected.
Figure 43–7
Surface electrocardiogram from leads V1, II, and V5 demonstrating 2:1 atrioventricular (AV) block with narrow QRS complexes. Although a definite diagnosis of type I or type II AV block cannot be made, longer rhythm strip recordings might reveal Wenckebach periodicity. Vagal maneuvers or exercise might assist further in the final diagnosis.
When two or more consecutive atrial impulses do not conduct to the ventricle, it is defined as high-grade AV block. It may be associated with a junctional or ventricular escape rhythm. Occasionally, runs of consecutive atrial impulses may fail to conduct to the ventricles for up to 10 to 20 s with or without an escape rhythm, resulting in ventricular asystole and syncope. The block is usually initiated by a conducted or blocked atrial or ventricular premature beat. The block persists until terminated by an escape beat. Unless a clearly defined reversible etiology is identified, permanent pacing is indicated.
Complete or third-degree AV block is characterized by failure of all P waves to conduct to the ventricle. This results in complete dissociation of P waves and QRS complexes. Complete AV block may occur as a result of block in the AV node or at the His-Purkinje level. In patients with block at the AV node level, the escape rhythm is usually junctional, with a narrow QRS complex (unless associated with preexisting bundle-branch block), at rates of 40 to 60 beats/min (Fig. 43–8). In complete heart block resulting from His-Purkinje disease, the escape rhythm is ventricular in origin, with a wide QRS interval and at rates of 20 to 40 beats/min.
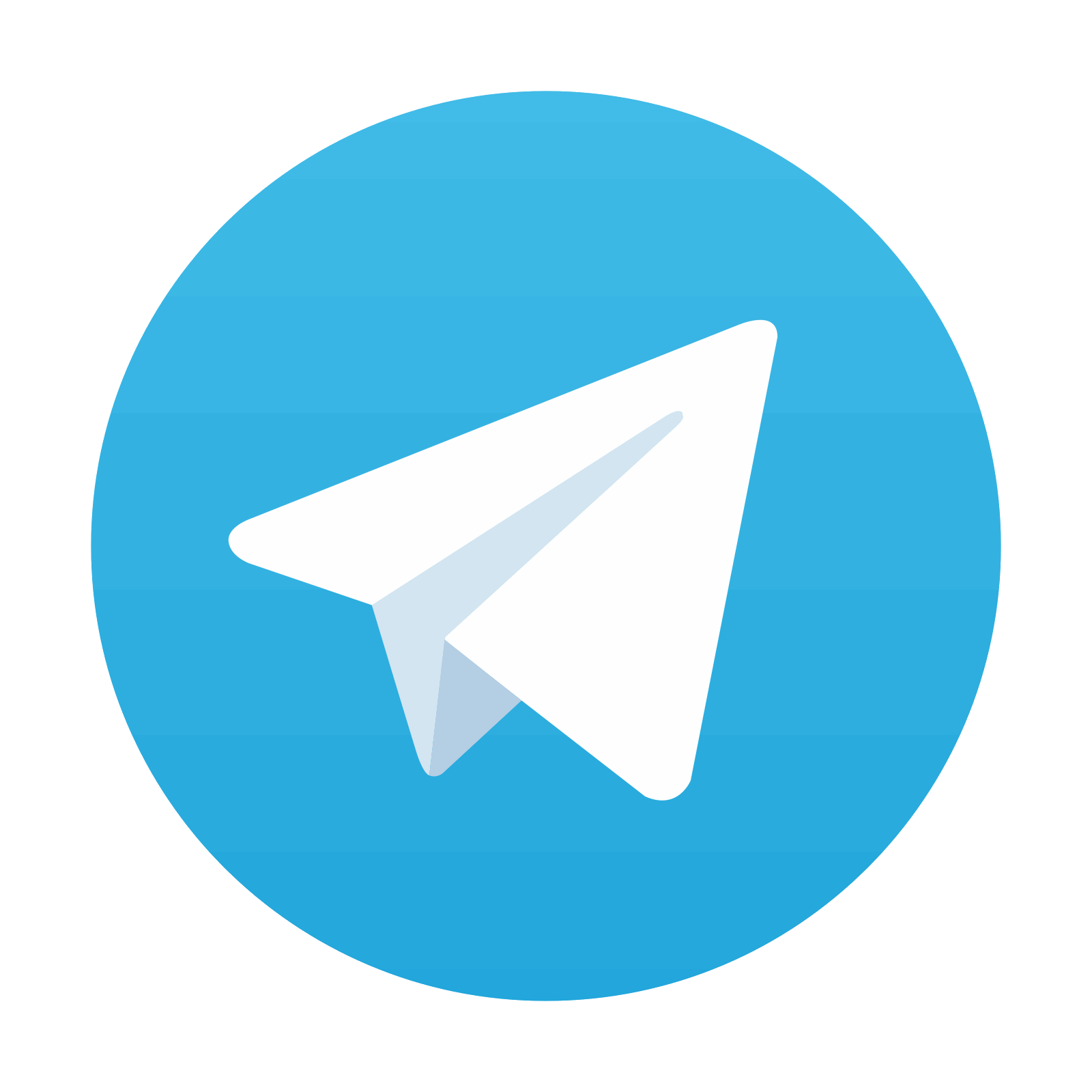
Stay updated, free articles. Join our Telegram channel

Full access? Get Clinical Tree
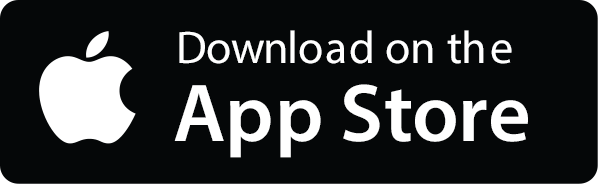
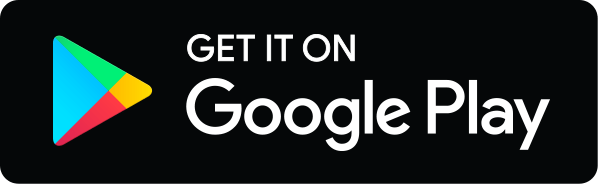