Basic Terms and Concepts of Mechanical Ventilation
Learning Objectives
On completion of this chapter, the reader will be able to do the following:
1 Define ventilation, external respiration, and internal respiration.
5 Write the formulas for calculating compliance and resistance.
7 Describe the airway conditions that can lead to increased resistance.
11 Give the percentage of passive filling (or emptying) for one, two, three, and five time constants.
Key Terms
• Acinus
• Airway opening pressure
• Airway pressure
• Alveolar distending pressure
• Ascites
• Auto-PEEP
• Bronchopleural fistulas
• Compliance
• Critical opening pressure
• Elastance
• Esophageal pressure
• External respiration
• Extrinsic PEEP
• Functional residual capacity
• Heterogeneous
• High-frequency jet ventilation
• High-frequency oscillatory ventilation
• High-frequency positive-pressure ventilation
• Homogeneous
• Intrinsic PEEP
• Internal respiration
• Mask pressure
• Mouth pressure
• Peak airway pressure
• Peak inspiratory pressure
• Peak pressure
• Plateau pressure
• Positive end-expiratory pressure
• Pressure gradient
• Proximal airway pressure
• Resistance
• Respiration
• Static compliance/static effective compliance
• Time constant
• Transairway pressure
• Transpulmonary pressure
• Transrespiratory pressure
• Transthoracic pressure
• Upper airway pressure
• Ventilation
Physiological Terms and Concepts Related to Mechanical Ventilation
The purpose of this chapter is to review some basic concepts of the physiology of breathing and to provide a brief description of the pressure, volume, and flow events that occur during the respiratory cycle. The effects of changes in lung characteristics (e.g., compliance and resistance) on the mechanics of ventilation are also discussed.
Normal Mechanics of Spontaneous Ventilation
Ventilation and Respiration
Spontaneous breathing, or spontaneous ventilation, is simply the movement of air into and out of the lungs. Spontaneous ventilation is accomplished by contraction of the muscles of inspiration, which causes expansion of the thorax, or chest cavity. During a quiet inspiration, the diaphragm descends and enlarges the vertical size of the thoracic cavity while the external intercostal muscles raise the ribs slightly, increasing the circumference of the thorax. Contraction of the diaphragm and external intercostals provides the energy to move air into the lungs and therefore represents the “work” required to inspire, or inhale. During a maximal spontaneous inspiration, the accessory muscles of breathing are used to increase the volume of the thorax.
Normal quiet exhalation is passive and does not require any work. During a normal quiet exhalation, the inspiratory muscles simply relax, the diaphragm moves upward, and the ribs return to their resting position. The volume of the thoracic cavity decreases, and air is forced out of the alveoli. To achieve a maximum expiration (below the end-tidal expiratory level), the accessory muscles of expiration must be used to compress the thorax. Table 1-1 lists the various accessory muscles of breathing.
TABLE 1-1
Terms, Abbreviations, and Pressure Gradients for the Respiratory System
Abbreviation | Term |
C | Compliance |
R | Resistance |
Raw | Airway resistance |
PM | Pressure at the mouth (same as Pawo) |
Paw | Airway pressure (usually upper airway) |
Pawo | Pressure at the airway opening; mouth pressure; mask pressure |
Pbs | Pressure at the body surface |
Palv | Alveolar pressure (also PA) |
Ppl | Intrapleural pressure |
Cst | Static compliance |
Cdyn | Dynamic compliance |
Pressure Gradients | ||
Transairway pressure (Pta) | Airway pressure − alveolar pressure | Pta = Paw − Palv |
Transthoracic pressure (PW) | Alveolar pressure − body surface pressure | PW (or PTT) = Palv − Pbs |
Transpulmonary pressure (PL) | Alveolar pressure − pleural pressure (also defined as the transalveolar pressure) | PL (or PTP) = Palv − Ppl |
Transrespiratory pressure (Ptr) | Airway opening pressure − body surface pressure | Ptr = Pawo − Pbs |
Respiration involves the exchange of oxygen and carbon dioxide between an organism and its environment. Respiration is typically divided into two components: external respiration and internal respiration (Box 1-1). External respiration involves the exchange of oxygen and carbon dioxide between the alveoli and the pulmonary capillaries. Internal respiration occurs at the cellular level and involves the movement of oxygen from the systemic blood into the cells, where it is used in the oxidation of available substrates (e.g., carbohydrates and lipids) to produce energy. Carbon dioxide, which is a major by-product of aerobic metabolism, is then exchanged between the cells of the body and the systemic capillaries.
Gas Flow and Pressure Gradients During Ventilation
An important point in appreciating how ventilation occurs is the concept of airflow. For air to flow through a tube or airway, a pressure gradient must exist (i.e., pressure at one end of the tube must be higher than pressure at the other end of the tube). Air will always flow from the high-pressure point to the low-pressure point.
Consider what happens during a normal quiet breath. Lung volumes change as a result of gas flow into and out of the airways caused by changes in the pressure gradient between the airway opening and the alveoli. During a spontaneous inspiration, the pressure in the alveoli becomes less than the pressure at the airway opening (i.e., the mouth and nose) and gas flows into the lungs. Conversely, gas flows out of the lungs during exhalation because the pressure in the alveoli is higher than the pressure at the airway opening. It is important to recognize that when the pressure at the airway opening and the pressure in the alveoli are the same, as occurs at the end of expiration, no gas flow occurs because the pressures across the conductive airways are equal (i.e., no pressure gradient).
Units of Pressure
Ventilating pressures are commonly measured in centimeters of water pressure (cm H2O). These pressures are referenced to atmospheric pressure, which is given a baseline value of zero. In other words, although atmospheric pressure is 760 mm Hg or 1034 cm H2O (1 mm Hg = 1.36 cm H2O) at sea level, atmospheric pressure is designated as 0 cm H2O. For example, when airway pressure increases by +20 cm H2O during a positive-pressure breath, the pressure actually increases from 1034 to 1054 cm H2O. Other units of measure that are becoming more widely used for gas pressures, such as arterial oxygen pressure (PaO2), are the torr (1 torr = 1 mm Hg) and the kilopascal ([kPa]; 1 kPa = 7.5 mm Hg). The kilopascal is used in the International System of units. (Box 1-2 provides a summary of common units of measurement for pressure).
Definition of Pressures and Gradients in the Lungs
Airway opening pressure (Pawo), is most often called mouth pressure (PM) or airway pressure (Paw) (Fig. 1-1). Other terms that are often used to describe the airway opening pressure include upper-airway pressure, mask pressure, or proximal airway pressure. Unless pressure is applied at the airway opening, Pawo is zero or atmospheric pressure.
A similar measurement is the pressure at the body surface (Pbs). This is equal to zero (atmospheric pressure) unless the person is placed in a pressurized chamber (e.g., hyperbaric chamber) or a negative-pressure ventilator (e.g., iron lung).
Intrapleural pressure (Ppl) is the pressure in the potential space between the parietal and visceral pleurae. Ppl is normally about −5 cm H2O at the end of expiration during spontaneous breathing. It is about −10 cm H2O at the end of inspiration. Because Ppl is difficult to measure in a patient, a related measurement is used, the esophageal pressure (PES), which is obtained by placing a specially designed balloon in the esophagus; changes in the balloon pressure are used to estimate pressure and pressure changes in the pleural space. (See Chapter 10 for more information about esophageal pressure measurements.)
Another commonly measured pressure is alveolar pressure (PA or Palv). This pressure is also called intrapulmonary pressure or lung pressure. Alveolar pressure normally changes as the intrapleural pressure changes. During spontaneous inspiration, PA is about −1 cm H2O, and during exhalation it is about +1 cm H2O.
Four basic pressure gradients are used to describe normal ventilation: transairway pressure, transthoracic pressure, transpulmonary pressure, and transrespiratory pressure (Table 1-1; also see Fig. 1-1).1
Transairway Pressure
Transairway pressure (Pta) is the pressure difference between the airway opening and the alveolus: Pta = Paw − Palv. Pta is therefore the pressure gradient required to produce airflow in the conductive airways. It represents the pressure that must be generated to overcome resistance to gas flow in the airways (i.e., airway resistance).
Transthoracic Pressure
Transthoracic pressure (PW) is the pressure difference between the alveolar space or lung and the body’s surface: Pbs: PW = Palv − Pbs. It represents the pressure required to expand or contract the lungs and the chest wall at the same time. It is sometimes abbreviated PTT or Ptt (TT [and tt], meaning transthoracic).
Transpulmonary Pressure
Transpulmonary pressure (PL or PTP), or transalveolar pressure, is the pressure difference between the alveolar space and the pleural space (Ppl): PL = Palv − Ppl.2–4 PL is the pressure required to maintain alveolar inflation and is therefore sometimes called the alveolar distending pressure. All modes of ventilation increase PL during inspiration, either by decreasing Ppl (negative-pressure ventilators) or increasing Palv by increasing pressure at the upper airway (positive-pressure ventilators). The term transmural pressure is often used to describe pleural pressure minus body surface pressure. (NOTE: An airway pressure measurement called the plateau pressure [Pplateau] is sometimes substituted for Palv. Pplateau is measured during a breath-hold maneuver during mechanical ventilation, and the value is read from the ventilator manometer. Pplateau is discussed in more detail later in this chapter.)
During negative-pressure ventilation, the pressure at the body surface (Pbs) becomes negative, and this pressure is transmitted to the pleural space, resulting in an increase in transpulmonary pressure (PL). During positive-pressure ventilation, the Pbs remains atmospheric, but the pressures at the upper airways (Pawo) and in the conductive airways (airway pressure, or Paw) become positive. Alveolar pressure (PA) then becomes positive, and transpulmonary pressure (PL) increases.
The definition of transpulmonary pressure varies in research articles and textbooks. Some authors define it as the difference between airway pressure and pleural pressure. This definition implies that airway pressure is the pressure applied to the lungs during a breath-hold maneuver, that is, under static (no flow) conditions.
Transrespiratory Pressure
Transrespiratory pressure (Ptr) is the pressure difference between the airway opening and the body surface: Ptr = Pawo − Pbs. Transrespiratory pressure is used to describe the pressure required to inflate the lungs and airways during positive-pressure ventilation. In this situation, the body surface pressure (Pbs) is atmospheric and usually is given the value zero; thus Pawo becomes the pressure reading on a ventilator gauge (Paw).
Transrespiratory pressure has two components: transthoracic pressure (the pressure required to overcome elastic recoil of the lungs and chest wall) and transairway pressure (the pressure required to overcome airway resistance). Transrespiratory pressure can therefore be described by the equations Ptr = Ptt + Pta or (Pawo − Pbs) = (Palv − Pbs) + (Paw − Palv).
Consider what happens during a normal, spontaneous inspiration (Fig. 1-2). As the volume of the thoracic space increases, the pressure in the pleural space (intrapleural pressure) becomes more negative in relation to atmospheric pressures. (This is an expected result according to Boyle’s law. For a constant temperature, as the volume increases, the pressure decreases.) The intrapleural pressure drops from about −5 cm H2O at end expiration to about −10 cm H2O at end inspiration. The negative intrapleural pressure is transmitted to the alveolar space, and the intrapulmonary, or intraalveolar (Palv), pressure becomes more negative relative to atmospheric pressure. The transpulmonary pressure (PL), or the pressure gradient across the lung, widens (Table 1-2). As a result, the alveoli have a negative pressure during spontaneous inspiration.
TABLE 1-2
Changes in Transpulmonary Pressure* Under Varying Conditions
PASSIVE SPONTANEOUS VENTILATION | ||
Pressure | End expiration | End inspiration |
Intraalveolar (intrapulmonary) | 0 cm H2O | 0 cm H2O |
Intrapleural | −5 cm H2O | −10 cm H2O |
Transpulmonary | PL = 0 − ( −5) = +5 cm H2O | PL = 0 −(−10) = 10 cm H2O |
NEGATIVE-PRESSURE VENTILATION | ||
Intraalveolar (intrapulmonary) | 0 cm H2O | 0 cm H2O |
Intrapleural | −5 cm H2O | −10 cm H2O |
Transpulmonary | PL = 0 − (−5) = +5 cm H2O | PL = 0 −(−10) = 10 cm H2O |
POSITIVE-PRESSURE VENTILATION | ||
Intraalveolar (intrapulmonary) | 0 cm H2O | 9-12 cm H2O† |
Intrapleural | −5 cm H2O | 2-5 cm H2O† |
Transpulmonary | PL = 0 − (−5) = +5 cm H2O | PL = 10 − (2) = +8 cm H2O† |
The pressure at the mouth or body surface is still atmospheric, creating a pressure gradient between the mouth (zero) and the alveolus of about −3 to −5 cm H2O. The transairway pressure gradient (Pta) is approximately (0 − [−5]), or 5 cm H2O. Air flows from the mouth into the alveoli, and the alveoli expand. When the volume of gas builds up in the alveoli and the pressure returns to zero, airflow stops. This marks the end of inspiration; no more gas moves into the lungs because the pressure at the mouth and in the alveoli equal zero (i.e., atmospheric pressure) (see Fig. 1-2).
During exhalation the muscles relax and the elastic recoil of the lung tissue results in a decrease in lung volume. The thoracic volume decreases to resting, and the intrapleural pressure returns to about −5 cm H2O. Notice that the pressure inside the alveolus during exhalation increases and becomes slightly positive (+5 cm H2O). As a result, pressure is now lower at the mouth than inside the alveoli and the transairway pressure gradient causes air to move out of the lungs. When the pressure in the alveoli and that in the mouth are equal, exhalation ends.
Lung Characteristics
Normally, two types of forces oppose inflation of the lungs: elastic forces and frictional forces. Elastic forces arise from the elastic properties of the lungs and chest wall. Frictional forces are the result of two factors: the resistance of the tissues and organs as they become displaced during breathing and the resistance to gas flow through the airways.
Two parameters are often used to describe the mechanical properties of the respiratory system and the elastic and frictional forces opposing lung inflation: compliance and resistance.
Compliance
The compliance of any structure can be described as the relative ease with which the structure distends. It can be defined as the opposite, or inverse, of elastance (e), where elastance is the tendency of a structure to return to its original form after being stretched or acted on by an outside force. Thus, C = 1/e or e = 1/C. The following examples illustrate this principle. A balloon that is easy to inflate is said to be very compliant (it demonstrates reduced elasticity), whereas a balloon that is difficult to inflate is considered not very compliant (it has increased elasticity). In a similar way, consider the comparison of a golf ball and a tennis ball. The golf ball is more elastic than the tennis ball because it tends to retain its original form; a considerable amount of force must be applied to the golf ball to compress it. A tennis ball, on the other hand can be compressed more easily than the golf ball, so it can be described as less elastic and more compliant.
In the clinical setting, compliance measurements are used to describe the elastic forces that oppose lung inflation. More specifically, the compliance of the respiratory system is determined by measuring the change (Δ) of volume (V) that occurs when pressure (P) is applied to the system: C = ΔV/ ΔP. Volume typically is measured in liters or milliliters and pressure in centimeters of water pressure. It is important to understand that the compliance of the respiratory system is the sum of the compliances of both the lung parenchyma and the surrounding thoracic structures. In a spontaneously breathing individual, the total respiratory system compliance is about 0.1 L/cm H2O (100 mL/cm H2O); however, it can vary considerably, depending on a person’s posture, position and whether he or she is actively inhaling or exhaling during the measurement. It can range from 0.05 to 0.17 L/cm H2O (50-170 mL/cm H2O). For intubated and mechanically ventilated patients with normal lungs and a normal chest wall, compliance varies from 40 to 50 mL/cm H2O in men and 35 to 45 mL/cm H2O in women to as high as 100 mL/cm H2O in either gender (Key Point 1-1).
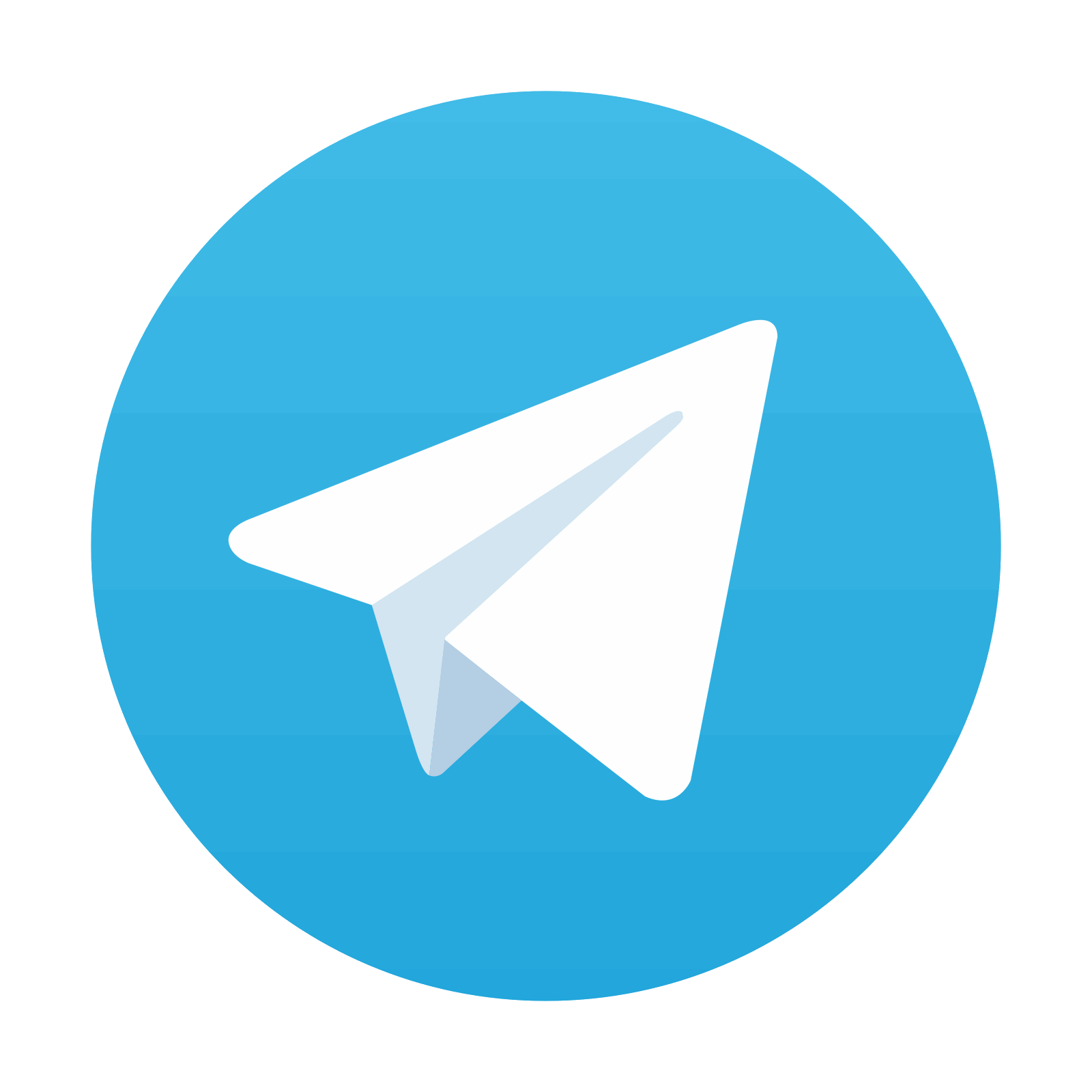
Stay updated, free articles. Join our Telegram channel

Full access? Get Clinical Tree
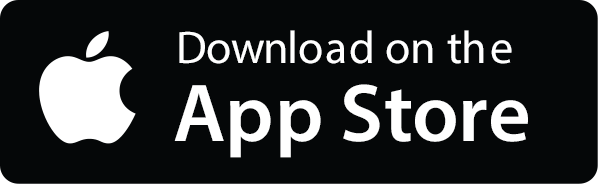
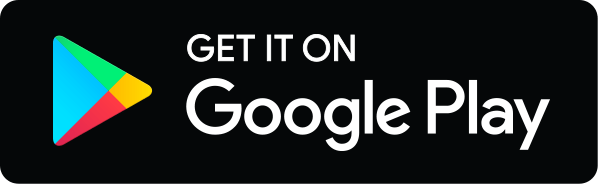