Arrhythmias in Children and in Patients with Congenital Heart Disease: Introduction
The physiology, natural history, and treatment of arrhythmias in fetuses, infants, children, and teenagers are influenced by developmental changes in cardiac dimensions; the hemodynamics, pharmacokinetics, and pharmacodynamics of antiarrhythmic drugs; and specific electrophysiologic features of the maturing heart. Furthermore, a growing understanding of the developmental changes in myocyte ion channels, intercellular connections, and autonomic nervous system influences is helping to unravel our knowledge of the gross changes that occur during maturation. Finally, cardiac arrhythmias in young individuals are relatively more likely to be related to structural congenital heart disease compared with arrhythmias in adults.
Congenital Abnormalities of the Specialized Conduction System
Recently supported by studies using immunohistochemical and molecular markers, the specialized conduction system of the human heart is most likely derived from specialized cardiomyocytes of the primary heart field along the four intersegmental zones of the primitive heart tube,1 the sinoatrial (SA) ring between the sinus venosus and primitive atrium (which gives rise to the sinus node and part of the atrioventricular [AV] node), the AV ring between the primitive atrium and primitive left ventricle (which contributes to the AV node), the primary ring between the primitive left ventricle and the bulbus cordis (which gives rise to the His bundle and bundle branches), and the ventriculoarterial ring between the bulbus cordis and the truncus arteriosus. According to this theory, during cardiac looping, these rings come together at the inner curvature of the heart tube, and portions of these rings lose their specialized character (Fig. 47–1). There are also contributions from neural crest cells to both the venous and arterial poles and from epicardium-derived cells (second heart field), especially for peripheral Purkinje fiber development and annulus fibrosis formation. This theory is supported by our recent appreciation for arrhythmogenic substrates that are localized to the proximal pulmonary veins, proximal great arteries, and AV annuli. In addition, and more germane to this chapter, reports of dissections of the specialized conduction systems from malformed hearts have proved complementary and have resulted in the accurate prediction of the anatomy of the conduction system in many forms of complex congenital heart disease.
Figure 47–1
Current theory of development of the specialized conduction system. Left. After initiation of normal rightward (“dextro”) looping of the early heart tube, rings at transitional zones appear. Middle. During later development and early septation, these rings appear at the putative junctions between chambers—the sinoatrial ring (SAR; teal), atrioventricular ring (AVR; blue), primary ring at the bulboventricular foramen (PR; yellow), and ventriculoarterial ring (VAR; green). Right. Position of rings in the developed heart. AS, aortic sac; PA, primitive atrium; SV, sinus venosus; VIS, ventricular inlet segment; VOS, ventricular outlet segment. Adapted with permission from Jongbloed MR, Mahtab EA, Blom NA, et al. Development of the cardiac conduction system and the possible relation to predilection sites of arrhythmogenesis. Scientific World Journal. 2008;8:239-269.
In levotransposition of the great arteries (l-TGA), also known as congenitally corrected transposition, there are both AV and ventriculoarterial discordance. Therefore, systemic venous return enters the pulmonary arteries, and pulmonary venous return is directed to the aorta, as they should. The majority have associated structural defects, most commonly ventricular septal defect (VSD) or pulmonic stenosis. The specialized AV conduction system is also structurally different, usually giving a QS or qR pattern in V1 and an rS or RS pattern in V6 on a standard electrocardiogram (ECG; Fig. 47–2). In most patients having situs solitus and l-TGA, the AV node is located anteriorly in the right atrium near the mitral–pulmonary junction. The His bundle is relatively elongated as it crosses anterior to the pulmonary valve annulus before bifurcating on the anterior ventricular septum. When a VSD is also present, the His bundle borders the anterosuperior quadrant of the defect. A second, hypoplastic posterior AV node may also be present just anterior to the coronary sinus. Rarely, it also connects with the ventricular mass via a separate posterior penetrating bundle or to the anterior AV node via a sling of conduction tissue (so-called Mönckeberg sling). Congenitally corrected transposition may also exist in the presence of situs inversus. In such cases, the AV node is usually located in a normal location at the apex of the triangle of Koch, especially if there is accompanying pulmonic stenosis or atresia.2 As in most complex congenital heart defects, the location of the specialized AV conduction system appears to be dictated by the alignment of the inlet portion of the ventricular septum with the annulus fibrosus.
Figure 47–2
Examples of electrocardiograms from patients with congenital heart disease who have both structural abnormalities of the specialized conduction system and abnormalities of cardiac chamber size caused by the hemodynamic alterations. A. An 8-year-old child with severe Ebstein anomaly of the tricuspid valve, showing marked right atrial enlargement and incomplete right bundle branch block. B. A 3-year-old child with levotransposition of the great arteries (l-TGA) and pulmonic stenosis, showing a septal q in V1 and RS in V6, as well as right ventricular hypertrophy. C. The cardiac anatomy of l-TGA, showing the specialized conduction system and its relationship to a VSD. D. A 6-month-old child with tricuspid atresia, illustrating left axis deviation, right atrial enlargement, and paucity of right-sided forces (abnormal R:S ratio in V1). E. A 3-year-old child with atrioventricular (AV) septal defect (AVSD), demonstrating left axis deviation and right ventricular hypertrophy (RSR’ in V1). F. Anatomic location of the AV conduction system in hearts with AVSD from the surgeon’s perspective (top) and in isolation (bottom). Part F is reproduced with permission from Ebels T, Anderson RH. Atrioventricular septal defects. In: Anderson RH, Baker EJ, Macartney FJ, Rigby ML, Shinebourne EA, Tynan M, eds. Paediatric Cardiology, 2nd ed. London: Churchill Livingstone; 2002:955.
Pathologic series of heart specimens having l-TGA demonstrate that the elongated penetrating bundle is either infiltrated with fibrous tissue or is completely disrupted. Data analysis shows an approximately 2% per year risk of developing AV block after diagnosis. It is generally recommended that prophylactic pacemaker placement is not necessary in these patients if their escape ventricular rates are greater than 50 beats/min unless they have additional hemodynamic abnormalities. In patients also having a VSD requiring surgical closure, the risk of intraoperative AV block is higher than it is in patients without l-TGA. Special surgical handling of the superior portion of the defect is required to avoid this complication. In such cases, if AV block is avoided, the risk of late AV block is not increased. AV block is congenital in 3.7% to 18.2% of infants with l-TGA3-5 and seems to be more prevalent in those with intact ventricular septum than in those also with a VSD.5
Patients with l-TGA have a higher than expected incidence of paroxysmal supraventricular tachycardia (PSVT), usually AV reciprocating tachycardia (AVRT), involving one or multiple accessory AV connections associated with the left-sided tricuspid valve. As a separate concern, because patients with l-TGA have a high incidence of structural tricuspid valve (systemic AV valve) abnormalities, atrial fibrillation also occurs commonly later in life. Tricuspid regurgitation is associated with increased mortality at 20 years of follow-up. Finally, in older patients with l-TGA, symptomatic ventricular tachycardia (VT) may also occur.
Tricuspid atresia is a congenital abnormality of alignment in which there is no connection between the atrial mass and the morphologically right ventricle. The right atrium ends in a muscular floor, and its only outlet is an atrial septal communication. The atrial and ventricular septa form normally (usually with a VSD). This causes the AV node to be located posterolateral to the blind-ending dimple in the muscular floor of the right atrium, maintaining a normal relationship with the tendon of Todaro and coronary sinus.
The surface ECG reflects hemodynamic and anatomic influences, with right atrial enlargement in almost all, left axis deviation in approximately 75%, and a short PR interval (<0.10 seconds) in up to 12% (see Fig. 47–2). Preexcitation occurs in between 0.29% and 0.51%. However, electrophysiologic evaluation of these patients demonstrates that only approximately 10% have a true accessory pathway, prompting the use of the term pseudopreexcitation in the others. Hence, clinical arrhythmias secondary to structural conduction system abnormalities are uncommon in patients with tricuspid atresia. An interesting form of Wolff-Parkinson-White (WPW) pattern may be first noted after an older form of the Fontan operation, in which the right atrial appendage is anastomosed to the right ventricular infundibulum (Björk-type modification). Acquired conduction across suture lines may give the appearance of a right anteroseptal accessory pathway and may even predispose to AVRT.6 It may also be treated by standard catheter ablation techniques.67
In this disease, the tricuspid valve does not differentiate properly from the underlying right ventricle, resulting in distal displacement of the tricuspid valve leaflets into the right ventricle. There may be significant tricuspid regurgitation into the thin-walled, hypocontractile portion of the right ventricle that lies between the tricuspid valve leaflet coaptation plane and the normally positioned annulus (so-called atrialized portion of the right ventricle). In moderate or severe cases, there may be chronic cyanosis, severe volume overload of both atria, and sometimes right ventricular outflow tract obstruction. The downward displacement of the tricuspid valve septal leaflet results in discontinuity of the central fibrous body with the septal AV ring. This may result in direct muscular connections and possible preexcitation. When present, accessory pathways are multiple in about 50% of patients and are most commonly located in the inferior half of the tricuspid valve annulus. Atriofascicular fibers may also exist. In addition, the AV node may be compressed and displaced toward the coronary sinus ostium. The WPW pattern occurs in 10% to 21% of patients with Ebstein anomaly. Even from early childhood, the ECG from moderately or severely affected patients shows marked right atrial enlargement and—if WPW syndrome is not present—right ventricular conduction delay (see Fig. 47–2). In patients without obvious preexcitation, absence of right ventricular conduction delay may be caused by subtle preexcitation and seems to be a strong predictor for the presence of an accessory AV connection.8 Complete AV block is rare, but first-degree block occurs in approximately 40% of patients with Ebstein anomaly.
This combination of physiologic and anatomic abnormalities results in a patient group with a high incidence of arrhythmias. PSVT contributes to morbidity but not to risk of sudden death, and its incidence increases with age at presentation. In an older large series of 220 cases of Ebstein anomaly, arrhythmia was the most common presenting symptom (42%) in the adolescent and adult age group but not in children. Actuarial survival was 59% at 10 years, but sudden death accounted for only 14% of deaths. In a retrospective analysis of predictors of long-term survival in 48 patients, 14% died by 5 months, none of whom had WPW syndrome. Of surviving patients, 24% had WPW syndrome, and there was 68% long-term survival (11 years mean follow-up). Although atrial fibrillation was associated with nonsurvival (P = .03), WPW syndrome did not appear to play a role in any deaths. Of eight older patients having experienced sudden cardiac death, ventricular fibrillation related to severe cardiomegaly was thought to be the cause.
Most tachycardias in patients having Ebstein anomaly can now be treated nonpharmacologically. The substrates of AV reciprocating tachycardia, AV nodal reentry tachycardia, and primary atrial tachycardias can be successfully ablated using catheter-based techniques, albeit with lower success rates than in patients having normal hearts. In some centers, simultaneous accessory pathway transsection with structural tricuspid valve surgery is still performed.
In all forms of AV septal defect (AVSD), there are predictable abnormalities of the specialized AV conduction system. Embryologically, the AV node always forms at the insertion of the inlet ventricular septum and the atrial septum. Because of the location of the AV septal deficiency in this disease, there is posteroinferior displacement of the AV node toward the ostium of the coronary sinus (Fig. 47–3). There is also a longer than normal penetrating bundle and relative hypoplasia of the anterior portion of the left bundle branch, resulting in the characteristic ECG findings: left axis deviation of the QRS complex with a superior counterclockwise vector loop in the frontal plane (see Fig. 47–2). Accessory AV connections may also exist in patients with AVSD, and they tend to be posteroseptal in location.
Figure 47–3
The heterotaxies. Characteristic gross organ abnormalities (top) and typical ECG findings (bottom) in A. Right atrial isomerism (“asplenia”). B. Left atrial isomerism (“polysplenia”). A depicts epiarterial bronchi (1), trilobed lungs (2), mirror-image right atrial appendages (3), and total anomalous pulmonary venous return (4). The rhythm strip shows evidence of duplicated conduction systems, with QRS axis changes after P wave morphology and PR segment changes. In B is shown hyparterial bronchi (1), bilobed lungs (2), mirror-image left atrial appendages (3), ipsilateral pulmonary venous return and azygos continuation of lower body venous return caused by an interrupted inferior vena cava (4), and multiple small spleens (8). The 12-lead electrocardiogram shows junctional rhythm. Common to both entities are common atrium, atrioventricular septal defect, and outflow tract obstructions (5); abnormalities in systemic venous return (6); and midline liver with gut malrotation (7). The anatomic figures are adapted with permission from Van Mierop LHS, Gessner IH, Schiebler GL. Asplenia and polysplenia syndromes. Birth Defects. 1972;8:36-43.
The heterotaxy syndromes are patterns of malformation involving multiple organs and thought to occur as a result of failure of lateralization of thoracic and abdominal viscera into either a normal pattern (situs solitus) or inverted pattern (situs inversus). They usually involve the cardiovascular system, often with severe congenital malformations. They are categorized as either right isomerism or left isomerism, although there is great variability within each group (see Fig. 47–3).
The cardiac conduction system is often structurally abnormal. Among patients with right isomerism (or “asplenia” in the older literature), structural heart defects tend to be more severe, and they may have duplication of sinus node structures, one in each side of the atrial mass, with normal or duplication of the specialized AV conduction systems. In those with left isomerism (or “polysplenia”), there is often a paucity of sinus node tissue, usually manifesting as slower than normal low atrial rhythms. These patients may develop complete AV block, especially in the presence of coincidental ventricular l-looping. Fetuses having heterotaxy with severe structural heart disease and congenital AV block have an especially high mortality rate (approaching 90%).
An interesting tachycardia almost unique to some heterotaxy patients is an AV reentry tachycardia that uses twin AV nodes. This should be suspected in any symptomatic patient having the combination of ventricular l-looping and an AVSD because it is this combination that predicts the presence of both anterior and posterior AV nodes. Catheter ablation of the AV conduction system responsible for the ventriculoatrial component of the reentry circuit has proven curative in these patients.
Arrhythmias Associated with Common Congenital Heart Defects
Among the most prevalent congenital heart defects are VSD, atrial septal defect (AVSD), pulmonary valve stenosis, and aortic valve stenosis.
Preoperatively, a nonsinus atrial rhythm may coexist in up to 10% of children and young adults with sinus venosus ASDs but much less commonly in those with isolated ostium secundum ASDs.9 Because chronic left-to-right shunting results in dilatation of the right atrium and right ventricle in both lesions, the relatively proximate location of sinus venosus defects with respect to the SA node may play a role in this observation. When considering older adults having unrepaired ASDs, however, the incidence of SA node dysfunction has been reported to be 22% to 65%. Starting in early childhood and probably as a consequence of right ventricular stretch, right ventricular conduction delay is ubiquitous. Similarly, right atrial enlargement may produce intra-atrial conduction delay, resulting in PR interval prolongation.
After childhood repair of ASD, the late-term (mean, 26 years) incidence of symptomatic atrial tachyarrhythmia is only approximately 4%.9 Atrial flutter and fibrillation are increasingly prevalent with increasing age at ASD presentation, increasing pulmonary-to-systemic flow ratio, and increasing pulmonary artery pressure. This prevalence approaches 50% in patients older than 50 years old and 80% in octogenarians. Surgical repair later in life may not protect these patients from atrial tachyarrhythmias. In an old series of 123 patients who had undergone repair of ostium secundum or sinus venosus ASDs at the Mayo Clinic, late follow-up (27-32 years) revealed that the incidence of atrial flutter or fibrillation increased directly with age at repair: 4% if operated on at 11 years old or younger, 17% if operated on at 12 to 24 years old, 41% if operated on at 25 to 41 years old, and 59% if operated on at older than 41 years old. More recent reports show additional risk factors for late postoperative atrial flutter or fibrillation: the presence of preoperative atrial flutter or fibrillation, the occurrence of immediate postoperative atrial flutter or fibrillation or junctional rhythm, and older age at surgery (>40 years).9 Pacemaker requirement for new onset sinus node dysfunction or AV block is low in all groups, ranging from 0% to 3.7%.9
When postoperative atrial flutter does occur, it seems to be potentiated by slow conduction within either the low lateral right atrium (between the atriotomy scar and the orifice of the inferior vena cava) or the subtricuspid isthmus. Catheter ablation within these zones is highly effective in long-term relief of tachycardia.10
Starting in the late 1990s, techniques using catheter-delivered devices to permanently close many secundum ASDs began to supplant surgery. Transient AV block has been reported in up to 6% of these procedures but with permanent AV block in only 0.1% at medium-term follow-up.9-11 The incidence of symptomatic atrial tachyarrhythmias after device closure is negligible in children and only approximately 1% per year in adults unless present before the procedure.12
Patients with VSD develop chronic volume overload in the right ventricle, left atrium, and left ventricle proportionate to the size of the left-to-right shunt. In patients with large defects, pulmonary and right ventricular hypertension may also occur. The long-term effects of having a VSD were investigated as part of the Second Natural History Study, published in 1993. Among 2408 patients entered into this study between 1958 and 1969, 93% were younger than 18 years old at entry. At the time of follow-up, ambulatory ECG (Holter) data from 419 patients having a VSD demonstrated that 15.3% had supraventricular arrhythmias versus 5% of control subjects (P <.0001). Compared with age-appropriate controls, premature ventricular beats were more frequent in 11% to 17% of patients younger than 40 years old (>4 per hour) and in 22% to 39% of those greater than 40 years old (>8 per hour). These incidences related to disease severity, occurring in 12% of those medically managed, 20% of those surgically managed, and 80% of those having Eisenmenger physiology. The presence of serious ventricular arrhythmias (defined as the presence of ventricular couplets, multiform premature ventricular beats, or VT) was determined from some combination of ECG, Holter, and exercise testing in all 1252 patients with a VSD. Table 47–1 shows the incidences of and independent risk factors for having serious ventricular arrhythmias. Although this work demonstrated that arrhythmia risk is related to severity of hemodynamic alterations, even small VSDs may be associated with symptomatic arrhythmias in adulthood, especially VT and atrial fibrillation.
Serious Ventricular Arrhythmiasa | Sudden Unexplained Death | |||
---|---|---|---|---|
Congenital Heart Defect | Incidence (%) | Independent Risk Factors | Odds Ratio | Incidence (%) |
Ventricular septal defect | 31.4 | Main pulmonary artery pressureb | 1.49 | 4.0 |
Agec | 1.51 | |||
NYHA classc | 8.53 | |||
Cardiomegalyc | 2.79 | |||
Pulmonic stenosis | 29.7 | Ageb | 1.05 | 0.5 |
Agec | 1.04 | |||
NYHA classc | 7.93 | |||
Cardiomegalyc | 3.21 | |||
Aortic stenosis | 44.8 | Left ventricular end-diastolic pressureb | 2.02 | 5.4 |
Aortic regurgitationc | 11.70 | |||
Genderc | 4.10 | |||
Prior aortic valve replacementc | 4.80 |
Catheter-delivered devices have been used to close muscular VSDs since about 1998. This technique is not associated with long-term arrhythmias or conduction defects13 except perhaps when performed in young infants.14 Newer devices for perimembranous defects do seem to carry risk of AV block and bundle branch block,15-17 although sufficient experience is not yet available.
Pulmonic stenosis results in right ventricular hypertension and hypertrophy proportionate to the degree of outflow tract obstruction. Treatment with surgical or balloon valvotomy can cause right ventricular volume overload from valve insufficiency. In the Second Natural History Study, among 182 patients having Holter data, 18.9% had supraventricular arrhythmias versus 5% of control subjects (P <.001), although this incidence was not influenced by prior valvotomy. However, only those with prior valvotomy had more premature ventricular beats than control subjects (P <.0001), suggesting a role for volume loading from pulmonary valve insufficiency. Table 47–1 shows the incidence of “serious” ventricular arrhythmia. The incidence did not increase with lesion severity, and the overall incidence of VT (2%) was not greater than that of control patients. However, the severity of hemodynamic abnormality as represented by New York Heart Association (NYHA) class and by the presence of cardiomegaly was related to the likelihood of serious ventricular arrhythmia.
Aortic stenosis results in left ventricular hypertension and hypertrophy proportionate to the severity of obstruction. Aortic valve insufficiency may be associated naturally or occur after surgical or balloon valvotomy. As ventricular compliance worsens, subendocardial ischemia eventually results from a decreasing diastolic transmural coronary perfusion gradient. In the Second Natural History Study, among 134 patients having Holter data, 24.8% had supraventricular arrhythmias versus 5% of control subjects (P <.001) unrelated to prior valvotomy. As in the case of pulmonic stenosis, 13% of patients having undergone prior valvotomy had premature ventricular beats at a rate exceeding normal for age (P <.0001 vs control subjects). The incidences of serious ventricular arrhythmias were high in these patients, as depicted in Table 47–1, with an overall incidence of VT (13%) much greater than in control subjects. This incidence increased with increasing lesion severity, unlike that in patients with pulmonic stenosis. Independent predictors of risk of serious ventricular arrhythmias were identified only in patients having undergone valvotomy or valve surgery (see Table 47–1) and included hemodynamic, demographic, and surgical procedure characteristics.
The issue of sudden death risk in patients with these common congenital heart defects is only now being unraveled. Based on the Second Natural History Study, the relationship of serious ventricular arrhythmia (from noninvasive monitoring) to symptoms or to sudden cardiac death in patients with VSD, pulmonic stenosis, or aortic stenosis is unclear (see Table 47–1). Although the incidence of serious ventricular arrhythmia is similar among the three groups, the risk of unexpected sudden death is significantly higher among patients with VSD (4%) and aortic stenosis (5.4%) compared with control subjects.
Late Arrhythmias after Complex Congenital Heart Surgery
This section considers arrhythmias that are of clinical concern long-term after the Mustard and Senning operations for d-transposition of the great arteries (d-TGA), Fontan-style palliative operations for functionally single ventricle, and surgical repair of tetralogy of Fallot (ToF). These conditions were selected for discussion because of their relatively high incidence in the adult population, the high prevalence of clinically important postoperative arrhythmias, and the important incidence of sudden death.
These patients should be evaluated as outpatients at least annually. SA node dysfunction (SAND) presents similarly to adults having structurally normal hearts. However, the standard ECG or rhythm strips in patients having cardiac symptoms must be interpreted carefully, especially after extensive atrial surgery. Atrial flutter (will be used interchangeably with intra-atrial reentry tachycardia [IART] in this section) commonly is associated with very low-amplitude flutter waves, and the ECG may appear as an accelerated junctional rhythm at 100 to 140 beats/min. Monotony of the QRS rate, application of adenosine, or cautious exercise testing will reveal the diagnosis. Even if there are no symptoms, ECG signs of SAND after atrial surgery requires regular surveillance. Generally, 24-hour ambulatory ECGs are sufficient every 2 to 5 years. In patients having chronic fatigue or dizziness at rest or with exercise, a 24-hour ECG and exercise test should be performed. It should be recognized that the maximum attained heart rate in these patients is usually below predicted, often with an abnormally pronounced reduction in heart rate during the immediate postexercise recovery phase. This relatively sudden decrease in heart rate immediately after exercise may correlate with the frequently observed fatigue and dizziness that many patients experience immediately after exercise.
Electrophysiologic testing should be performed in any patient who has experienced syncope, presyncope, or palpitations suggestive of a tachyarrhythmia or who has documented nonsinus tachycardia. (If atrial flutter is the cause of symptoms, it can be argued that electrophysiologic study can be deferred unless catheter ablation is to be performed.) Even if noninvasive monitoring shows sinus or atrial bradycardia as the source for syncope, cardiac catheterization and electrophysiologic study may be useful before pacemaker implantation for several reasons: (1) evaluation of AV nodal and His-Purkinje system function, (2) identification and possible ablation of coexisting tachyarrhythmias, and (3) demonstration of venous and atrial anatomy pertinent to permanent lead implantation. Syncope as a symptom is especially nonspecific in this patient group because it may be caused by SAND (d-TGA, single ventricle), AV block (ToF, single ventricle), ventricular tachyarrhythmias (ToF, d-TGA, single ventricle), atrial flutter (ToF, d-TGA, single ventricle), or PSVT (ToF, d-TGA, single ventricle). Although diagnostic techniques such as attached ambulatory event recorders (“loop recorders”) or insertable loop recorders are reasonable first approaches, there is a tendency toward invasive electrophysiologic testing very early in the course of these patients.
Device management of SAND and AV block in this patient group is guided by the recommendations of the American Heart Association (AHA)/American College of Cardiology (ACC)/Heart Rhythm Society (HRS) Joint Committee on Device-Based Therapy (Table 47–2).18 These recommendations are only guidelines; we have offered permanent pacing in children having less defined symptoms of fatigue, morning headaches, or daytime somnolence and only moderate bradycardia while awake.
Class I: Permanent cardiac pacing is beneficial, useful, and effective. Benefit >>> risk. “Should” be performed.
|
Class IIa: There is conflicting evidence or a divergence of opinion about the usefulness or efficacy of permanent cardiac pacing. Benefit >> risk. Additional studies with focused objectives are needed. “It is reasonable to perform.”
|
Class IIb: Usefulness or efficacy is less well established by evidence or opinion. Benefit ≥ risk. Additional studies with broad objectives needed. Additional registry data would be helpful. “May be considered.”
|
Class III: Conditions for which there is evidence or general agreement that permanent cardiac pacing is not useful or effective and in some cases may be harmful. Risk ≥ benefit. “Should not be performed because it is not helpful and may be harmful.”
|
Recommendations for Implantable Cardioverter-Defibrillators in Pediatric Patients and Patients with Congenital Heart Disease |
Class I: ICD is beneficial, useful, and effective. Benefit >>> risk. “Should” be performed.
|
Class IIa: There is conflicting evidence or a divergence of opinion about the usefulness or efficacy of ICD. Benefit >> risk. Additional studies with focused objectives needed. “It is reasonable to perform.”
|
Class IIb: Usefulness or efficacy is less well established by evidence or opinion. Benefit ≥ risk. Additional studies with broad objectives needed. Additional registry data would be helpful. “May be considered.”
|
Class III: Conditions for which there is evidence or general agreement that ICD is not useful or effective and in some cases may be harmful. Risk ≥ benefit. “Should not be performed because it is not helpful and may be harmful.”
|
After surgical correction of congenital heart defects, the long-term incidence of sudden cardiac death is 25 to 100 times greater than in age-matched control subjects.19 Silka et al20 performed a population-based study of sudden cardiac death after repair of a broad range of congenital heart defects. Similar to the Natural History Study, the highest incidence of sudden cardiac death was observed among patients with aortic stenosis, with an incidence of 3% at 10 years of follow up, 13% at 20 years, and 20% at 30 years. ToF patients demonstrated a time-dependent intermediate risk with an incidence of 1.2% at 10 years, 2.2% at 20 years, 4% at 25 years, and 6% at 30 years. More recently, Walsh and Cecchin21 also reported that sudden death risk is greatest among patients having had cyanotic heart disease or left ventricular outflow tract obstructive lesions, ranging from 1.5 of 1000 patient-years for ToF to 4.9 and 5.4 of 1000 patient-years for aortic stenosis and atrial switch operation for d-TGA, respectively. Other than resuscitated sudden death, additional indications for implantable cardioverter-defibrillator (ICD) implantation in congenital heart disease include (1) symptomatic sustained VT (consider ablation or surgical repair if applicable; class I indication), (2) recurrent syncope of unknown cause in the presence of ventricular dysfunction or inducible VT (class IIa indication), and (3) recurrent syncope in the presence of ventricular dysfunction and no evidence of arrhythmia.18
Device implantation requires knowledge of the often complex anatomy, and placement of epicardial leads requires the assistance of a cardiothoracic surgeon. This is the rule in the presence of AV block and single-ventricle physiology and among all patients with extracardiac venopulmonary connections and no systemic venous atrium. This issue is discussed in detail in the therapeutics section of this chapter.
These operations were designed to direct the systemic venous return to the mitral valve and the pulmonary venous return to the tricuspid valve. An atrial septectomy is required in both instances. The Mustard operation involves placement of a pantaloon-shaped patch of artificial material, which encircles the caval orifices; bisects the subtricuspid isthmus inferiorly; and often damages the sinus node, perinodal structures, and their blood supply superiorly. The Senning operation is conceptually similar but uses the right atrial free wall and atrial septal flap to accomplish redirection of venous returns.
When evaluated by ambulatory ECG monitoring 5 to 10 years postoperatively, only 20% and 40% of patients having Mustard procedures are still in sinus rhythm, 7% to 35% are in junctional rhythm, up to 40% are in slow ectopic atrial rhythm, and 10% have atrial flutter. In an older series of 249 patients, there was a 2.4% per year loss of sinus rhythm, and the presence of junctional rhythm carried a 2.1-fold increased risk of developing atrial flutter (P <.05). At 23 years median follow-up of 86 adults who had undergone the Mustard operation, up to 48% had a history of SVT (mostly IART), and up to 22% had pacemakers.19,22,23 Risk factors for SVT (including atrial flutter and IART) include pulmonary hypertension, right ventricular dysfunction, and junctional rhythm. SA node dysfunction appears to be more common after the Mustard procedure than after the Senning operation. Both brady- and tachyarrhythmias increase in frequency with increasing time from operation.
The presence of atrial flutter increases the risk of sudden death up to 4.7-fold (P <.01). The mechanism of sudden death has been thought to be 1:1 AV conduction during atrial flutter, either directly causing ventricular fibrillation or followed by marked overdrive suppression of all subsidiary pacemakers with resultant asystole. (However, primary VT in those having depressed right ventricular function may also contribute to this risk.19,22-24) For these reasons, aggressive treatment of atrial flutter is desirable. Occasionally, patients having impaired AV conduction remain asymptomatic with atrial flutter as a consequence of 2-4:1 AV conduction, and may not require aggressive treatment. Patients who present with atrial flutter (with or without symptoms) should undergo transvenous or transesophageal overdrive pacing termination or direct current cardioversion. Chronic treatment with an AV node-blocking agent alone after a first episode is reasonable until a timeline of recurrences is established, especially if that event is not associated with syncope or other evidence of severe cardiovascular decompensation. After recurrences, three options are available: (1) antitachycardia pacing (with an AV node blocking drug), (2) antiarrhythmic medications (class I agent plus an AV node blocking drug and bradycardia pacing, or class III agent with bradycardia pacing), or (3) radiofrequency (RF) catheter ablation. Implantation of an antitachycardia pacemaker may obviate the need for potentially proarrhythmic drugs, but the safety and efficacy of this modality must be demonstrated by electrophysiologic testing in the drug-free state. The risk of tachycardia acceleration to a more dangerous rhythm must be considered, especially if defibrillation is not a component of the device. RF ablation of IART can now be performed with success rates at long-term follow-up of more than 80%. This is now our preferred approach in patients with recurrent IART (Fig. 47–4).
Figure 47–4
Successful and unsuccessful radiofrequency catheter ablation sites from patients having undergone the Mustard or Senning operations for d-transposition of the great arteries d-TGA. Rectangular sites represent linear lesions for intra-atrial reentry tachycardia, and circular sites represent focal lesions for “focal atrial tachycardia.” In the Mustard figure, the gray region represents the systemic venous atrium, and the stippled zone represents the subtricuspid isthmus. In the Senning figure, the lightly shaded area is a part of the systemic venous atrium, whose roof (atrial free wall flap to the viewer’s left) has been removed from its attachment to the atrial septal remnant (vertical dashed line), and a portion of the pulmonary venous atrium adjacent to the tricuspid valve is visible to the viewer’s right of the vertical dashed line. IVC, inferior vena cava; MV, mitral valve; PVA, pulmonary venous atrium; SVA, systemic venous atrium; SVC, superior vena cava; TV, tricuspid valve. Reproduced with permission from Kanter RJ, Papagiannis J, Carboni MP, et al. Radiofrequency catheter ablation of supraventricular tachycardia substrates after Mustard and Senning operations for d-transposition of the great arteries. J Am Coll Cardiol. 2000;35:428-441.
The group of procedures known as Fontan operations has been used as a palliation for single-ventricle physiology for 35 years. These operations result in passive flow from the systemic venous system into the pulmonary arterial system, originally with an interposed right atrium. Because the Fontan-style operations have gone through several iterations over the decades, the incidences and prevalences of resultant atrial tachyarrhythmias must be interpreted cautiously. Initially performed as an atrial-to-pulmonary connection, modifications were later developed to reduce late complications related to significant atrial dilatation and hypertrophy. Among more than 2600 patients having undergone any kind of Fontan operation over 2 decades of publications, atrial tachycardia occurs in 0% to 57% of patients, at follow-up ranging from 3 months to 17 years.19,25 The prevalences vary according to Fontan type: 12% to 57% for atriopulmonary or Bjork-type Fontans, 3% to 26% for lateral tunnel or total cavopulmonary connections (TCPC), and 0% to 9% for extracardiac conduits. Risk factors for the development of atrial tachycardias include the duration of follow-up, right atrial enlargement, AV valve surgery, early postoperative arrhythmias, atriopulmonary connection (vs lateral tunnel), and lateral tunnel (vs extracardiac conduit).19,23,25,26 Moreover, animal studies and clinical reports show a lower incidence of immediate and medium-term (2 to 8 years) postoperative atrial tachycardias in TCPC patients when the crista terminalis is not violated by suture lines. Most centers have evolved to extracardiac conduits whenever possible to prevent hypertension in any portion of the atrial mass.
SA node dysfunction may occur after Fontan operations, especially if more than one procedure is performed. The National Institutes of Health–sponsored Pediatric Heart Network reported 32% of patients younger than 18 years of age were not in sinus rhythm at follow-up, and this prevalence continued to increase over time.27,28 The incidence of pacemaker requirement in patients after the modified Fontan operation ranges from 5% to 16%, related to SAND, congenital AV block, and postoperative AV block. The reported incidence of late arrhythmic death overall is 2% to 3%.25,28 These data will also be influenced by newer surgical techniques.
Pharmacologic suppression of atrial tachycardias is especially difficult after Fontan-type operations. The importance of AV synchrony and adequate diastolic filling time makes arrhythmia control especially crucial. Combinations of antiarrhythmic drugs, often including amiodarone, are often necessary. However, there is an unusually high incidence of amiodarone-induced thyrotoxicosis in Fontan patients. Antitachycardia pacing is occasionally helpful but may also result in dangerous rhythm acceleration.
Because of an unpredictable imbalance in natural anticoagulant and procoagulant factors, Fontan patients with atrial tachyarrhythmias may be at unusually high risk for atrial and pulmonary thrombosis, sometimes with catastrophic results. Transesophageal echocardiography should be performed before conversion of IART in these patients because transthoracic echocardiography has been shown to underestimate the prevalence of thrombus. Warfarin should be used for at least 3 weeks after cardioversion, although its efficacy is somewhat controversial in this patient group.
Compared with catheter ablation within the atria after other heart operations, RF catheter ablation of IART in the Fontan right atrium is more difficult because of the frequent presence of multiple tachycardia circuits and the increased atrial wall thickness. In the large series of patients undergoing catheter ablation for IART after surgery for congenital heart disease by Triedman et al,29 63 patients had undergone the Fontan operation. Multivariate factors relating to acute success included use of an irrigated ablation catheter (odds ratio [OR], 3.8) and, inversely, a history of atrial fibrillation (OR, 0.43). Favorable outcome at medium-term follow-up was related to complete procedural success (OR, 3.9); the use of an electroanatomic mapping technique (OR, 2.4); and, inversely, the number of IART circuits (OR, 0.58). After the atrio-pulmonary connection type of Fontan procedure, corridors of conduction critical to IART circuits and amenable to ablation seem to be clustered in the posterolateral right atrium associated with the atriotomy scar, in the subtricuspid isthmus (when present), and related to the atriopulmonary connection itself (Fig. 47–5). Standard techniques for identifying ideal targets for ablation in macroreentry atrial tachycardias, such as demonstration of diastolic potentials and concealed entrainment mapping, may not be effective in these patients. Successful ablation of more than 80% of these circuits is now being achieved, although the recurrence rate is approximately 50% at 2 to 3 years of follow-up.29,30 Among patients having IART after newer Fontan-type operations, catheter access to the tachycardia circuits may require novel approaches, including transthoracic puncture.31
Figure 47–5
Successful radiofrequency catheter ablation sites in patients having had Fontan operation (predominantly atriopulmonary connection) and intra-atrial reentry tachycardia (n = 40 sites). Open circles are anterior, mostly associated with the right atrial appendage-to-pulmonary artery anastomosis; closed circles within the dottedrectangle are in the region of the right atriotomy; and closed circles within the dottedcircle are in the region of the subtricuspid isthmus (when present). Adapted with permission from Collins KK, Love BA, Walsh EP, et al. Location of acutely successful radiofrequency catheter ablation of intra-atrial reentrant tachycardia in patients with congenital heart disease. Am J Cardiol. 2000;86:969-974.
These marginal procedural outcomes and high recurrence rates often combined with poor hemodynamics has prompted a surgical approach to atrial tachycardia ablation after the atriopulmonary-style Fontan operation. The so-called “Fontan conversion operation” improves hemodynamics by reducing the atrial mass and by changing the atriopulmonary connection to a lateral tunnel or extracardiac connection. Concomitantly, arrhythmia surgery via a modified cryothermal maze or Cox-maze procedure is customized to the patient’s congenital venoatrial and AV anatomy32 (Fig. 47–6). IART recurrence rates have been reported to be less than 20% up to 3 years after the procedure.32,33
Figure 47–6
Typical cryolesions used for the intraoperative maze procedure to treat atrial intra-atrial reentry tachycardia arising in the right atrium in patients who had undergone an older Fontan-style operation for complex heterotaxy and atrioventricular (AV) septal defect, at the time of surgical conversion to an extracardiac conduit from inferior vena cava (IVC) to the pulmonary arteries. A and B course from the AV valve annulus to the amputated right-sided atrial appendage and to the atriotomy, respectively, and C and D course from the orifice of the amputated IVC to the AV valve annulus and to the coronary sinus ostium, respectively. Adapted with permission from Mavroudis C, Backer CL, Deal BJ, et al. Total cavopulmonary conversion and maze procedure for patients with failure of the Fontan operation. J Thorac Cardiovasc Surg. 2001;122:863-71.
Repair of ToF requires closure of a perimembranous VSD and enlargement of the right ventricular outflow tract (“infundibulectomy”). This usually requires a transannular patch, resulting in pulmonary valve insufficiency. The presence of a ventricular septal patch, a right ventriculotomy, preoperative left ventricular dilatation (if an aortopulmonary shunt had been placed before repair), postoperative right ventricular dilatation (caused by pulmonary valve insufficiency), preoperative right ventricular hypertension, and preoperative cyanosis create the substrate for ventricular arrhythmias. Furthermore, if postoperative AV block results from the VSD patch placement, long-term dyssynchronous right ventricular pacing may contribute to left ventricular dysfunction.
After repair of ToF, permanent complete AV block occurs in fewer than 1% of operated patients in the modern surgical era.34 This is substantially less than after AVSD repair (%3 to 4%) or VSD with l-TGA (10%). That said, in Hokanson and Moller’s35 series, even transient complete AV block may be associated with late risk of sudden death. Approximately 80% of patients have complete right bundle branch block (RBBB); an additional 11% have the combination of RBBB with left axis deviation; and 3% have the combination of RBBB, left axis deviation, and first-degree AV block. In the absence of prior AV block, isolated RBBB or RBBB with axis deviation is generally a benign finding. However, it has specific clinical implications: (1) It will never be possible to identify progressive ventricular dilatation or hypertrophy from the ECG. (2) We are now learning that the dyssynchronous ventricular activation, which results from bundle branch block, may be deleterious to overall myocardial performance. Modern echocardiographic techniques, including tissue Doppler strain imaging and the Tei index, can identify patients with decreased regional and global left ventricular dysfunction,36 decreased exercise capability,37 and increased risk of ventricular arrhythmias.37 (3) In the presence of proximal RBBB, the development of left bundle branch block, which occasionally occurs later in life, places these patients at risk for complete AV block.
Occasional patients undergo pacemaker insertion in the postoperative period but later regain AV conduction. In Batra et al’s38 series, among 72 patients having complete postoperative AV block during surgery for congenital heart disease (1.3% of their total patient population), seven patients (9.6%) regained AV conduction at 18 to 113 days postoperation. None redeveloped AV block at 4.4 ± 2.6 years of follow-up. However, in Liberman et al’s39 report of 15 patients who developed late-onset complete AV block, 12 patients had not experienced immediate postoperative complete AV block. Nine patients had undergone VSD repair and four AVSD repair. Seven of the patients required placement of a pacemaker more than 6 years after the operation. We believe that lifelong surveillance of AV conduction, at least by serial ECGs, is prudent in this patient group.
Ventricular tachyarrhythmias are the major cause of paroxysmal symptoms or sudden death in the ToF population. Hence, risk stratification for the purpose of primary prevention is currently an area of intense interest. In the presence of RBBB, an unusually increased QRS duration appears to be sensitive and perhaps specific for such risk, with a cutoff value of ≥180 ms providing the greatest accuracy. The coexistence of increased JT-interval dispersion may enhance the positive and negative predictive value, suggesting that both repolarization and depolarization abnormalities are important. A rapid increase of these measurements is especially ominous. Application of magnetic resonance imaging to quantify pulmonary valve regurgitant and ventricular ejection fractions (in the presence of a transannular patch) and tissue Doppler imaging to identify ventricular dyssynchrony are newer modalities being investigated. In patients with poor hemodynamics, follow-up is stricter than it is in those with excellent hemodynamics. Equivocal or minor symptoms may be evaluated with event recorders, but paroxysmal palpitations or syncope merit invasive electrophysiologic testing.
The demonstration of symptomatic sustained monomorphic VT by noninvasive means is an indication for electrophysiologic testing if catheter ablation is anticipated. In the era of ICDs, electrophysiologic testing as a guide for antiarrhythmic drug selection is becoming antiquated. In the absence of symptoms, ventricular programmed stimulation could also be considered if a patient has impaired hemodynamics or markedly prolonged QRS duration plus high-grade ventricular ectopy.
After ToF repair, the predictive value of programmed ventricular stimulation results has become better defined than for patients with other defects. Not surprisingly, inducibility of ventricular arrhythmias increases with advancing age at repair and time since repair.42 The induction of monomorphic or polymorphic sustained VT at electrophysiology study has been reported as a reliable predictor of future arrhythmic events and had better prognostic value than known noninvasive risk factors.40 However, the positive predictive value is still poor when this modality is used as part of a screening strategy (67% in the presence of “indications” vs 25% as a screening tool).
Partly because of the relatively high incidence of the defect, ToF constitutes the largest subgroup of ICD recipients who also have congenital heart disease and hence is the most useful model for exploring the contribution of historical, postsurgical, anatomic, and physiologic factors to risk of dangerous ventricular arrhythmias. Using data collected on a group of these patients with ICDs placed for primary prevention, Khairy et al41 developed a scoring system that predicts the risk of experiencing an appropriate shock for ventricular tachyarrhythmia (Table 47–3). Although this scoring system was developed in the highest risk patients and not validated in the general ToF population, its use may add an important component to risk assessment of all ToF patients, with the caveat that even “appropriate” ICD discharge may not necessarily be used as a surrogate for sudden cardiac death.
Variable | Pointsa |
---|---|
Prior palliative shunt | 2 |
Inducible sustained VT | 2 |
QRS duration ≥180 ms | 1 |
Ventriculotomy incision | 2 |
Nonsustained VT | 2 |
LVEDP ≥12 mm Hg | 3 |
Symptomatic VT requires treatment. In the current era of device and ablation therapy, antiarrhythmic drug therapy has become less popular. Nevertheless, the efficacy of phenytoin, mexiletine, propranolol, amiodarone, and sotalol has been reported in small series. Class Ia drugs are not very useful for sustained VT in these patients, and class Ic agents may be especially proarrhythmic. Because of potential drug side effects, suboptimal efficacy, and difficulty in proving efficacy, nonpharmacologic therapies are now favored. After complete tachycardia circuit mapping in the electrophysiology laboratory, surgical resection, cryoablation, and microwave ablation have all met with variable success. RF catheter ablation has been used to successfully interrupt macroreentry VT circuits in several patients, even when there are multiple VT circuits and when VT is poorly hemodynamically tolerated. Newer electroanatomic mapping systems are very helpful in these patients. Programmed ventricular stimulation before hospital discharge and again at several months postablation is recommended to ensure successful elimination of VT substrate unless an ICD is also used. The ICD is an excellent primary treatment modality for infrequent sustained monomorphic VT and is mandatory for patients having resuscitated sudden death (class I indication). They provide antitachycardia pacing as well as a safety net of cardioversion or defibrillation.
Less clear are the indications for treating asymptomatic patients with high-grade ventricular ectopy. If their hemodynamics are acceptable, we would only follow these patients closely. In those with poor hemodynamics, options include ventricular programmed stimulation to help identify patients with inducible sustained tachycardia; surgical correction of hemodynamic abnormality when possible; or less desirable, empiric implantation of an ICD. Until there are randomized clinical trials involving treatment and nontreatment of such patients, the best approach remains unclear.
Specific Arrhythmias in the Young
This section discusses arrhythmias specific to or requiring special considerations in this age group.
Fetal arrhythmias are detected by auscultation during routine evaluation by the obstetrician or by ultrasound surveillance performed for routine evaluation of fetal development in about 3% of all pregnancies. Fetal echocardiography may be used to discriminate arrhythmia types by using M-mode analysis of atrial and ventricular wall motion or of valve opening and closure and by using Doppler flow across valves in the aorta or in the pulmonary artery and veins.42 Echocardiographic tissue Doppler velocity imaging may be used to evaluate the onset and pattern of propagation of atrial and ventricular mechanical activation, providing additional information about the mechanism of arrhythmias and conduction abnormalities.43 Construction of a ladder-type diagram from this information has been dubbed the “fetal kinetocardiogram”44 (Fig. 47–7). Magnetocardiography has also been used to elucidate the mechanism of arrhythmias and conduction abnormalities45-47 (Fig. 47–8) and more recently has included multichannel magnetocardiography48 and vector magnetocardiography49 to improve diagnostic capacity. Signal amplitude of the fetal magnetocardiogram allows for indirect assessment of chamber enlargement and may be more sensitive than echocardiography for atrial enlargement.50 Repolarization abnormalities can also be assessed with magnetocardiography, allowing for prenatal diagnosis of prolonged QT syndrome.51,52 Fetal ECG has also been used as a “real-time” method for rhythm evaluation and allows for assessment of repolarization abnormalities53-58 but is associated with technical difficulties in performance, especially in the early third trimester. Two-dimensional conventional ultrasound examination provides information about the physiologic response of the fetus to arrhythmias, including heart failure. Specifically, signs of fetal heart failure (hydrops fetalis) include ascites, pleural and pericardial effusions, and increased thickness of fetal scalp tissue from edema. Doppler evaluation of the fetal venous circulation provides earlier and more sensitive assessment of cardiovascular dynamics and health.59 Using current techniques, fetal arrhythmias may be detected as early as 6 weeks of gestation.
Figure 47–7
Kinetocardiography using tissue Doppler imaging for diagnosis of fetal arrhythmias. (Top left) Four-chamber apical view with regions of interest identified by colored cursors: lateral mitral valve annulus (teal), lateral tricuspid valve annulus (yellow), posterior left atrium (LA; red), and posterior right atrium (RA; green). (Top right) Corresponding and simultaneous tissue velocity imaging curves during sinus rhythm, showing much greater velocities for annular movement than posterior atrial wall, with annular motion away from the transducer representing ventricular filling and annular motion toward the transducer during ventricular systole (S). Asterisks and arrows represent onset and end of atrial contraction. The timing between mechanical events from identical structures can then be measured and displayed as a ladder diagram (bottom). This example represents premature atrial beats from the right atrium. A, atrial “kick”; E, early; LV, left ventricle; RV, right ventricle. Adapted with permission from Rein A, O’Donnell C, Geva T, et al. Use of tissue velocity imaging in the diagnosis of fetal cardiac arrhythmias. Circulation. 2002;106:1827-1833.
Figure 47–8
Magnetocardiograms from a 32-week-old fetus with second-degree isoimmune atrioventricular (AV) block. A. Before treatment with transplacental dexamethasone, the only P waves (arrows) that conduct to the ventricles do so by an accessory AV connection. B. After maternal treatment, there is 2:1 AV conduction (one non-preexcited QRS for every two very large amplitude P waves). Reproduced with permission from Cuneo BF, Strasburger JF, Wakai RT. Magnetocardiography in the evaluation of fetuses at risk for sudden cardiac death before birth. J Electrocardiol. 2008;41:116.e1-e6.
About 70% of fetal arrhythmias are isolated extrasystoles (90% of these, atrial). They are usually benign, usually resolving shortly after birth.60 It should be remembered that nonconducted premature atrial contractions may represent single AV reciprocating beats caused by an accessory pathway and may be a harbinger of impending fetal tachycardia; hence, some increase in the frequency of fetal monitoring may be indicated. Similarly, irregularity of the fetal heart rhythm may occur because of second-degree AV block from clinically unsuspected damage from maternal antibodies.47
Nonsinus fetal tachycardia represents about 15% to 20% of reported fetal arrhythmias. Most of these patients have orthodromic AV reentrant tachycardia.61 Atrial flutter occurs with less frequency, and other SVTs and VTs are rarely seen.62 AV reentrant tachycardia typically has a rate of 250 to 300 beats/min. It is most commonly initiated in fetuses by premature atrial contractions and terminated by AV nodal block, probably influenced by autonomic factors.47 The permanent form of junctional reciprocating tachycardia (PJRT) has a heart rate not exceeding 240 beats/min, signs of heart failure, and resistance to therapy.63 Atrial flutter has an atrial rate of 350 to 500 beats/min, typically with a ventricular rate half that of the atrial rate caused by 2:1 AV conduction, but other conduction patterns are seen.64 It is rarely seen before 27 weeks of gestation, probably because the critical atrial size required to maintain macro-reentry is not achieved until that age. Junctional tachycardia and VT may present with a ventricular rate faster than the atrial rate, but 1:1 retrograde conduction during these tachycardias make their diagnosis difficult using conventional ultrasound techniques. Magnetocardiography,65,66 fetal ECG,57 and “fetal kinetocardiography” may be helpful in prenatal diagnosis of these arrhythmias.43-55
Persistent regular fetal bradycardia constitutes about 10% of fetal arrhythmia and is usually caused by complete AV block. Fetal ultrasonography shows a regular pattern of ventricular contractions, which is slower and dissociated from the rate of atrial contractions. A minority of patients have associated congenital heart disease, usually severe. Patients with associated structural heart disease and those diagnosed in the first trimester have an extraordinarily high rate of fetal demise.67 Nonconducted atrial bigeminal rhythm results in a regular ventricular bradycardia that must be discriminated from complete AV block. Ultrasonography demonstrates an alternating long–short sequence of atrial mechanical events with a ventricular contraction sandwiched within the shorter interval of atrial events. Sinus bradycardia in fetuses may be associated with the congenital prolonged QT syndrome,45,68,69 and findings of persistent sinus bradycardia in the fetus should prompt assessment of ventricular repolarization, either in utero with magnetocardiographic51 or fetal ECG57 recording or an ECG after delivery.
Treatment decisions for fetal tachycardia are based on the duration and rate of tachycardia, the fetal response (signs of fetal hydrops, diminished fetal activity), and gestational age. Maternal administration of digoxin (loading with 500 μg every 8 h for three doses, attempting to achieve maternal level of 2 ng/mL) has traditionally represented first-line therapy but has a poor response rate when the fetus has hydrops.70 Although there is increased risk of inducing premature labor, intramuscular injection of digoxin into the fetal thigh may be effective. Sotalol and flecainide71 have also been shown to be effective in the treatment of fetal atrial flutter and AV reentry with excellent transplacental transmission72 with and without concomitant digoxin.71,73 Recent publications favor amiodarone for second-line therapy, especially when fetal hydrops is present.50,70,74,75
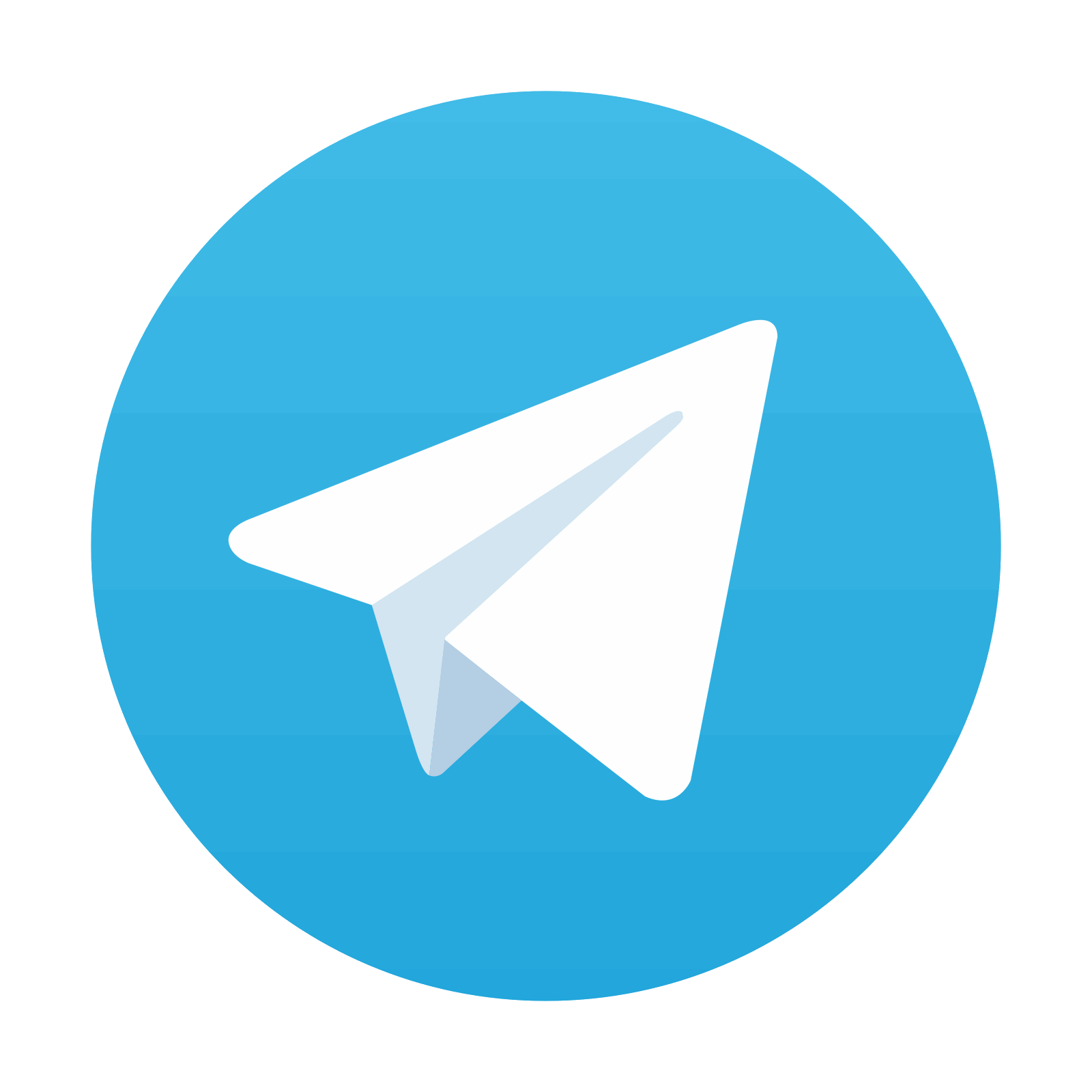
Stay updated, free articles. Join our Telegram channel

Full access? Get Clinical Tree
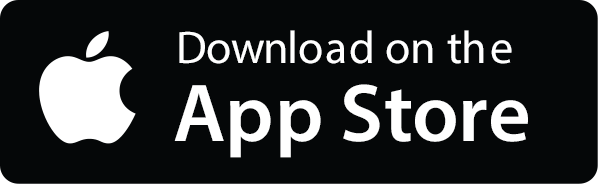
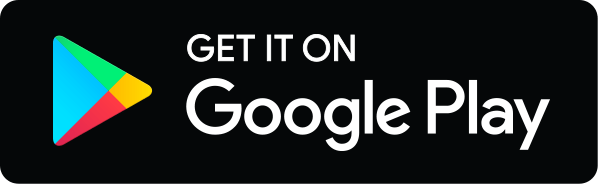
