Introduction and historical perspective
Venous thromboembolic disease includes deep vein thrombosis (DVT) and pulmonary embolism (PE). The first recorded case of thrombosis of the lower limb is believed to be found in the Hindu medicine writings of Susruta, an Indian healer who lived around 800 BC, where he described a patient with a “swollen and painful leg, which was difficult to treat”.1 While only a few thrombosis specialists will be familiar with Susruta’ s contribution to the field, all are familiar with the contributions of Rudolph Virchow. Virchow, a Prussian physician born in 1821, is credited with coining the term “embolism” after discovering the relationship between a blood clot that forms within a blood vessel (thrombus), and a blood clot that breaks loose from the original and travels through the bloodstream to occlude the pulmonary vessels (embolus).1 In addition, Virchow was the first to identify the pathogenic triad for thrombosis: stasis, vessel wall damage, and hypercoagulability. Virchow’ s triad has stood the test of time and today, it is still the foundation upon which prevention and treatment of venous thromboembolism (VTE) is based.
The first randomized trial for the treatment of VTE was performed by Barritt and Jordan in 1960.2 They showed that treatment of PE with heparin and oral anticoagulants reduced the risk of recurrent PE and reduced mortality compared with no anticoagulant therapy (Table 64.1). Since then, there has been considerable progress in the development of new anticoagulants (e.g. low molecular weight heparin (LMWH), fondaparinux) for the prevention and treatment of VTE. Unfortunately, despite these advances, VTE remains a leading cause of morbidity and mortality.
Table 64.1b Landmark trials in the long-term treatment of venous thromboembolism
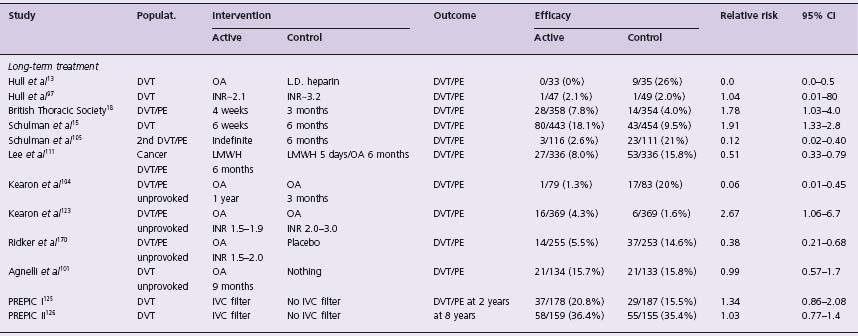
Populat, population studied; 95% Cl, 95% confidence interval of relative risk; OA, oral anticoagulants; LD, low-dose subcutaneous heparin; DVT, deep vein thrombosis; PE, pulmonary embolism; LMWH, low molecular weight heparin; IVC, inferior vena caval filter; * secondary outcome measure; ** DVT detected on mandatory bilateral venography; # additional prophylaxis used: heparin 18%, LMWH 26% and compression stockings 30%;A fixed, weight-adjusted dose without laboratory monitoring.
In this chapter, we will briefly review the natural history and pathogenesis of VTE and provide an overview of evidence-based management of VTE divided into three categories: diagnosis, treatment, and prevention.
Proximal deep vein thrombosis
is defined as a DVT that involves the popliteal and more proximal veins. Distal deep vein thrombosis is defined as a DVT that is confined to the calf veins (including the calf trifurcation).
Incidence, natural history and prognosis
Venous thromboembolism is rare before the age of 16 years, probably because the immature coagulation system is resistant to thrombosis. However, the risk of VTE increases exponentially with advancing age (i.e. 1.9-fold per decade), rising from an annual incidence of approximately 30/100 000 at 40 years, to 90/100 000 at 60 years, and 260/100 000 at 80 years.3–5
DVT usually starts in the calf veins.6 When DVT causes symptoms, over 80% involve the popliteal or more proximal veins.6,7 However, if venography is used to diagnose DVT in asymptomatic high-risk patients (e.g. following orthopedic surgery) only about one-third of venous thrombi are proximal. The majority of isolated calf DVT will undergo spontaneous lysis; however, 20% of these thrombi subsequently extend to involve the proximal veins, usually within a week of presentation.8 Non-extending calf DVT rarely causes PE, whereas proximal DVT often does.9 The majority (70%) of patients with symptomatic proximal DVT have asymptomatic PE and vice versa.10 Only about 25% of patients with symptomatic PE have symptoms or signs of DVT.11
It is estimated that 10% of symptomatic PE cause death within an hour of onset12 and that, left untreated, about one-third of patients with initially non-fatal PE will have a fatal recurrence.2 Untreated, symptomatic proximal DVT progresses to symptomatic PE in about one half of cases and, in general, the risk of recurrent VTE is highest within days or weeks of an acute event.13 The risk of recurrent VTE remains elevated in patients with thrombosis that was unprovoked or associated with persistent risk factors (e.g. malignancy, congenital hypercoagulable states) compared to those in whom VTE was associated with a transient risk factor (e.g. surgery).14–19
Venous thrombi are composed primarily of fibrin and red blood cells. They form when the following elements are present: venous stasis, vessel wall damage, and hyperco-aguability (Virchow’s triad). Classification of risk factors for VTE according to this pathogenic triad remains valuable.
Venous stasis
The importance of venous stasis as a risk factor for VTE is demonstrated by the fact that most DVT associated with stroke affect the paralyzed leg,20 and most DVT associated with pregnancy affect the left leg,21 the iliac veins of which are prone to extrinsic compression by the pregnant uterus and the right common iliac artery.
Vessel wall damage
Venous endothelial damage, usually as a consequence of accidental injury, manipulation during surgery (e.g. hip replacement) or iatrogenic injury, is an important risk factor for VTE. Hence, three-quarters of proximal DVT which complicate hip surgery occur in the operated leg22 and thrombosis is common with indwelling venous catheters.23
Hypercoagulability
A complex balance of naturally occurring coagulation and fibrinolytic factors, and their inhibitors, serves to maintain blood fluidity and hemostasis. Inherited or acquired changes in this balance predispose to thrombosis. The most important inherited biochemical disorders associated with VTE are due to:
- defects in the naturally occurring inhibitors of coagulation including deficiencies of antithrombin, protein C or protein S
- resistance to activated protein C caused by factor V Leiden
- a mutation in the 3’ untranslated region of the prothrombin gene (G20210A) which is associated with a 25% increase in prothrombin levels.
The first three of these disorders are rare in the normal population (combined prevalence of <1%), have a combined prevalence of 5% in patients with a first episode of VTE24 and are associated with a 10 to 40-fold increase in the risk of VTE.25 The factor V Leiden mutation is common, occurring in 5% of Caucasians and 20% of patients with a first episode of VTE (i.e. fourfold increase in VTE risk).25,26 The prothrombin gene mutation occurs in 2% of Caucasians and about 5% of those with a first episode of VTE (i.e. 2.5-fold increase in risk).25–27 Hyperhomocysteinemia, due to hereditary and acquired factors, is also associated with VTE.28
Elevated levels of a number of coagulation factors (I, II, VIII, IX, XI) are associated with thrombosis in a “dose-dependent” manner.29–31 It is probable that such elevations are often inherited, with strong evidence for this with factor VIII.29 Prothrombotic abnormalities of the fibrinolytic system have questionable importance.
Acquired hypercoagulable states include estrogen therapy, antiphospholipid antibodies (anticardiolipin antibodies and/or lupus anticoagulants), systemic lupus erythematosus, malignancy, combination chemotherapy, and surgery.3 Patients who develop immunologically related heparin-induced thrombocytopenia (HIT) also have a very high risk of developing arterial and venous thromboembolism.32 Unlike the congenital abnormalities, acquired risk factors are often transient, which has important implications for the duration of anticoagulant prophylaxis and treatment.
Combinations of risk factors and risks tratification
The risk of developing VTE depends on the prevalence and severity of risk factors (Box 64.1).3
BOX 64.1 Risk factors for venous thromboembolism
Patient factors
Previous venous thromboembolism*
Age over 40
Pregnancy, puerperium
Marked obesity
Inherited hypercoagulable state
Underlying condition and acquired factors
Malignancy*
Estrogen therapy
Cancer chemotherapy
Paralysis*
Prolonged immobility
Major trauma*
Lower limb injuries*
Heparin-induced thrombocytopenia
Antiphospholipid antibodies
Type of surgery
Lower limb orthopedic surgery*
General anesthesia >30min
* Common major risk factors for venous thromboembolism
Objective testing for DVT and PE is important because clinical assessment alone is unreliable. Failure to diagnose VTE exposes patients to the risk of fatal PE. However, inappropriate use of anticoagulants exposes patients to the risk of serious bleeding complications including fatal hemorrhage.
Diagnosis of deep vein thrombosis
Venography is the criterion standard for the diagnosis of DVT.7,33 However, because of its invasive nature, technical demands, costs, and risks associated with contrast media, non-invasive tests have been developed, of which venous ultrasound imaging (VUI) and D-dimer testing are the most important.
Clinical assessment
Although clinical assessment cannot unequivocally confirm or exclude DVT, clinical evaluation using empiric assessment or a structured clinical model (Table 64.2) can stratify patients as having a low (5% prevalence), moderate (25% prevalence) or high (60% prevalence) probability of DVT.34 Such categorization is useful in guiding the performance and interpretation of objective testing.34–38
Table 64.2 Clinical model for determining clinical suspicion of DVT171
Variables | Points |
Active cancer (treatment ongoing or within previous 6 months or palliative) | 1 |
Paralysis, paresis or recent plaster immobilization of the lower extremities | 1 |
Recently bedridden >3 days or major surgery within the previous 12 weeks requiring general or regional anesthesia | 1 |
Localized tenderness along the distribution of the deep venous system | 1 |
Entire leg swollen | 1 |
Calf swelling 3 cm > asymptomatic side (measured 10 cm below tibial tuberosity) | 1 |
Pitting edema confined to the symptomatic leg | 1 |
Collateral superficial veins (non-varicose) | 1 |
Previously documented deep vein thrombosis | 1 |
Alternative diagnosis as likely or greater than that of DVT | -2 |
Total points (pretest probability for DVT): | |
Score >2: High Score 1–2: Moderate Score 0: Low |
NOTE: In patients with symptoms in both legs, the more symptomatic leg is used.
Venous ultrasound imaging (VUI)
With this modality, pressure is applied to the proximal veins with an ultrasound probe to determine compressibility.7,39 Incompressibility of a vein is considered diagnostic for DVT. VUI has a sensitivity for proximal DVT of 97% and a specificity of 94% in symptomatic patients which, on average, translates into a positive predictive value of 97% and a negative predictive value of 98% for proximal DVT.7,40
The utility of VUI for diagnosing calf vein thrombosis is more controversial. VUI is technically more difficult to perform in the calf and some studies have reported the accuracy of this approach to be poor (e.g. sensitivity of 70%).7,40 Recently, investigators have proposed that a single complete compression ultrasound that includes examination of the calf veins should be used to exclude DVT. Studies using this method have reported an incidence of VTE of 0.5% during 3 months follow-up after a negative examination, establishing that negative VUI that includes the calf veins excludes VTE.41–43 However, this method has the potential to diagnose calf DVT that would have spontaneously lysed without treatment and to yield false-positive results, thereby exposing patients to the risk of bleeding due to anticoagulant therapy without clear benefit.
At most centers, if DVT cannot be excluded by a normal proximal VUI in combination with other results (e.g. low clinical probability or normal D-dimer (Table 64.3)) treatment is withheld and a follow-up VUI is performed after 1 week to detect extending calf vein thrombosis (2% of patients).7 If the second VUI examination is normal, the risk of symptomatic VTE during the next 6 months is less than 2%.7
Table 64.3 Diagnostic tests which confirm or exclude DVT
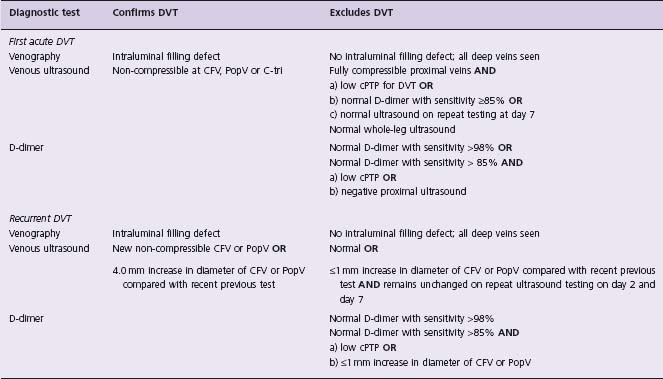
CFV, common femoral vein; PopV, popliteal vein; C-tri, calf vein trifurcation; cPTP, clinical pretest probability.
The accuracy of VUI in asymptomatic postoperative patients who have a high risk for DVT is poor with a sensitivity for proximal DVT of only 62%,7 and such screening is not recommended in patients who have received prophylaxis.44
Computed tomographic (CT) venography and magnetic resonance (MR) venography
The limitations of VUI in diagnosing DVT in certain clinical settings, e.g. isolated pelvic DVT and asymptomatic patients, has stimulated interest in alternative imaging modalities such as CT venography and MR venography. In one review of studies comparing CT venography with VUI, the sensitivity and specificity were reported as 89–100% and 94–100%, respectively, for all DVT.45 However, the majority of the studies included in the review were case series and given the cost, exposure to radiation, and limited availability of CT venography, it is unlikely that this modality will play an important role in the diagnosis of DVT.
Magnetic resonance venography can be performed with injection of gadolinium or without any contrast (MR direct thrombus imaging). A recent meta-analysis of studies comparing MR venography with conventional venography reported a pooled sensitivity of 92% and specificity of 95% of MR venography for proximal DVT.46 However, there was significant heterogeneity between the studies and, therefore, these pooled estimates should be interpreted with caution. MR direct thrombus imaging looks promising, but has only been evaluated in a single accuracy study to date.47 As with CT venography, cost and availability will inhibit the widespread use of MR for diagnosis of DVT.
D-dimer blood testing
D-dimer is formed when cross-linked fibrin is broken down by plasmin and levels are usually elevated with DVT and/or PE. Normal levels can help to exclude VTE, but elevated D-dimer levels are non-specific and have low positive predictive value.48,49 D-dimer tests differ markedly as diagnostic procedures for VTE. A normal result with a very sensitive (i.e. 98%) D-dimer assay excludes VTE on its own.48–50 However, very sensitive D-dimer tests have low specificity (40%) which limits their utility because of high false-positive rates.50 In order to exclude DVT and/or PE, a normal result with a less sensitive D-dimer assay (i.e. 85%) needs to be combined with either a low clinical pprobability or another objective test that has negative predictive value but is also non-diagnostic on its own (see Table 64.3).35,38,51–53 As less sensitive D-dimer assays are more specific (70%), they yield fewer false-positive results. Specificity of D-dimer decreases with aging54 and with co-morbid illness such as cancer.55 Consequently, D-dimer testing has limited value in the diagnosis of VTE in hospitalized patients and is unhelpful in the early postoperative period.
Diagnosis of recurrent DVT
Persistent abnormalities of the deep veins are common following DVT.7,56 Therefore, diagnosis of recurrent DVT requires evidence of new clot formation. Tests that can diagnose or exclude recurrent DVT are noted in Table 64.3.7,56,57
Diagnosis of pulmonary embolism
Pulmonary angiography is the criterion standard for the diagnosis of PE, but it has similar limitations to venography.58
Computed tomographic pulmonary angiography (CTPA)
Computed tomographic pulmonary angiography (also known as spiral or helical CT) with peripheral injection of contrast dye is the current standard diagnostic test for PE.59–62 Helical CT technology has rapidly advanced from use of single detector scanners to use of progressively larger numbers of detectors (termed multidetector CT), which enable more detailed examination of the pulmonary arteries.
Current evidence suggests that CTPA is non-diagnostic in 6% of patients, and that among adequate examinations, sensitivity is 83%, specificity is 96%, positive predictive value is 86% and negative predictive value is 95%.59 Accuracy varies according to the size of the largest pulmonary artery involved: positive predictive value was 97% for pulmonary emboli in the main or lobar artery, 68% for segmental arteries, and 25% for subsegmental arteries. Predictive values were also influenced by clinical assessment of pulmonary embolism probability: positive predictive value is 96% with high, 92% with intermediate, and 58% with low clinical probability (8% of patients); negative predictive value is 96% with low, 89% with intermediate, and 60% with high clinical probability (5% of patients).59
The ability of CTPA to exclude pulmonary embolism has also been evaluated in management studies in which anticoagulant therapy was withheld in patients with negative CTPA.61 More recent studies suggest that less than 2% of patients with a negative CTPA for pulmonary embolism will return with symptomatic VTE during follow-up.60–62 Taken together, these observations suggest the following:
- intraluminal filling defects in lobar or larger pulmonary arteries are generally diagnostic for PE
- a good-quality normal CTPA excludes PE if clinical suspicion is low or moderate
- intraluminal defects confined to segmental pulmonary arteries are generally diagnostic for PE if clinical suspicion is moderate or high, but should be considered non-diagnostic if suspicion is low or there are discordant findings (e.g. negative D-dimer)
- intraluminal defects confined to subsegmental pulmonary arteries are non-diagnostic and patients with such findings require further testing.
CTPA delivers a substantial dose of radiation to the chest, which increases the risk of cancer. For this reason, CTPA should be used selectively, particularly in younger women.63
Magnetic resonance angiography (MRA)
Gadolinium-enhanced pulmonary MRA offers advantages over CTPA in that it can be performed on patients with an allergy to iodinated contrast media and it avoids exposure to radiation. However, only a limited number of small studies evaluating MRA for diagnosis of PE have been performed.64 In addition, a warning has been issued by the Federal Drug Administration about an increased risk of nephrogenic systemic fibrosis in patients with severe renal insufficiency (glomerular filtration rate <30 mL/min) who are exposed to gadolinium. Like CTPA, MRA is able to identify alternative pulmonary diagnoses, and can be extended to look for concomitant DVT.
Ventilation-perfusion lung scanning
In the past, the imaging modality typically used to investigate patients with suspected PE was a ventilation-perfusion lung scan. More recently, CTPA has supplanted this imaging modality, although lung scanning is still used, particularly when CTPA is contraindicated because of renal failure or associated radiation exposure to the chest (e.g. in young women). A normal perfusion scan excludes PE65,66 but is found in a minority (10–40%) of patients.11,54,67 Perfusion defects are non-specific; only about one-third of patients with defects have PE.67,68 The probability that a perfusion defect is due to PE increases with size and number of defects, and the presence of a normal ventilation scan (“mismatched” defect).67,68 A lung scan with mismatched segmental or larger perfusion defects is termed “high probability.”68 A single mismatched defect is associated with a prevalence of PE of 80%.69 Three or more mismatched defects are associated with a prevalence of PE of 90%.69 Lung scan findings are highly age dependent with a relatively high proportion of normal scans, and a low proportion of non-diagnostic scans, in younger patients.54
Clinical assessment
As with suspected DVT, clinical assessment is useful at categorizing probability of PE (Table 64.4).37,70
Table 64.4 Model for determining clinical suspicion of PE71,172
Variables | Points |
Clinical signs and symptoms of deep vein thrombosis (minimum leg swelling and pain with palpation of the deep veins) | 3.0 |
An alternative diagnosis is less likely than pulmonary embolism | 3.0 |
Heart rate >100 beats/min | 1.5 |
Immobilization or surgery in the previous 4 weeks | 1.5 |
Previous deep vein thrombosis/pulmonary embolism | 1.5 |
Hemoptysis | 1.0 |
Malignancy (treatment ongoing or within previous 6 months or palliative) | 1.0 |
Total points (pretest probability for PE): | |
Score <4: PE unlikely * | |
Score ≥4: PE likely * * |
*low;
**score of 4 or 5 = moderate, score of 6 or higher = high.
D-dimer testing
As previously discussed when considering diagnosis of DVT, a normal D-dimer result, alone50 or in combination with another negative test,49,62,70,71 can be used to exclude PE (Table 64.5).
Table 64.5 Diagnostic tests which confirm or exclude PE
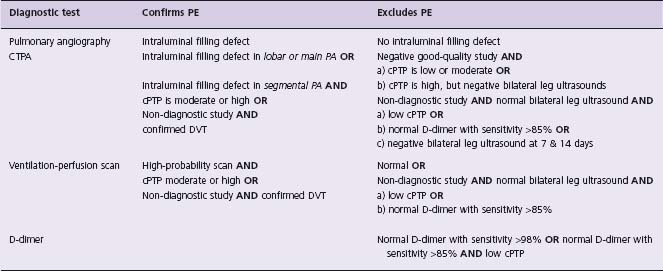
PA, pulmonary artery; CTPA, computed tomographic pulmonary angiography; cPTP, clinical pretest probability.
Management of patients with non-diagnostic combinations of non-invasive tests for PE
Patients with non-diagnostic imaging for PE at presentation have, on average, a prevalence of PE of 20% and therefore further investigations to exclude this diagnosis are required.39,67 The first step is to perform imaging investigations (venous ultrasound, venography) to look for DVT. If DVT is confirmed, it can be concluded that the patient’ s symptoms are due to PE. If imaging studies are negative for DVT, we recommend one of the following management approaches (Table 64.5):
- withhold anticoagulants and perform serial venous ultrasounds to check for evolving proximal DVT (after 1 and 2 weeks)
- perform CTPA or lung scanning if either of these tests has not been performed, or
- repeat CTPA after 24 hours (to reduce the risk of contrast-induced nephrotoxicity).
If serial VUI for DVT (two additional tests a week apart) is negative, the subsequent risk of recurrent VTE during the next 3 months is less than 1%11,70 which is similar to that after a normal pulmonary angiogram.58
Anticoagulants are the mainstay of treatment for VTE. The objectives of anticoagulant therapy are to prevent extension and potentially fatal embolization of the initial thrombus, and to prevent recurrent VTE (early and late).
Acute treatment of VTE
Low molecular weight heparin for a minimum of 5 days, administered by subcutaneous injection on an outpatient basis, has become standard practice for initial treatment of patients with an uncomplicated DVT and normal renal function72 (Class I, Level A). Many trials have established that weight-adjusted LMWH (without laboratory monitoring) is as safe and effective as adjusted-dose unfractionated intravenous heparin (UFH) for the treatment of acute VTE73 (see Table 64.1). Alternatives to LMWH include twice-daily subcutaneous UFH (with or without laboratory monitoring) and fondaparinux administered once daily by subcutaneous injection (without laboratory monitoring)74–76 (see Table 64.1) (Class I, Level A). If unmonitored subcutaneous UFH is used it should be administered in weight-adjusted doses (i.e. 333 units/kg initially followed by 250 units/kg every 12 hours).75 Patients with severe renal impairment or those who are considered at high risk for bleeding should be treated in hospital with intravenous UFH titrated according to activated partial thromboplastin time corresponding to plasma heparin levels from 0.3 to 0.7 IU/mL anti-Xa activity by the amidolytic assay77 (Class II, Level C). Patients with thrombosis associated with HIT or with a past history of HIT should be treated with danaparoid, argatroban or lepirudin78-80 (Class I, Level C).
A similar approach can be used for the initial treatment of PE, including treatment as an outpatient, as long as the patient is hemodynamically stable72,81,82 (Class I, Level A). No trials have specifically randomized acute PE patients to be treated either in hospital or at home. However, in one randomized controlled trial comparing fixed-dose UFH and LMWH, 39% (52/134) of patients who had PE were treated entirely at home75 (see Table 64.1
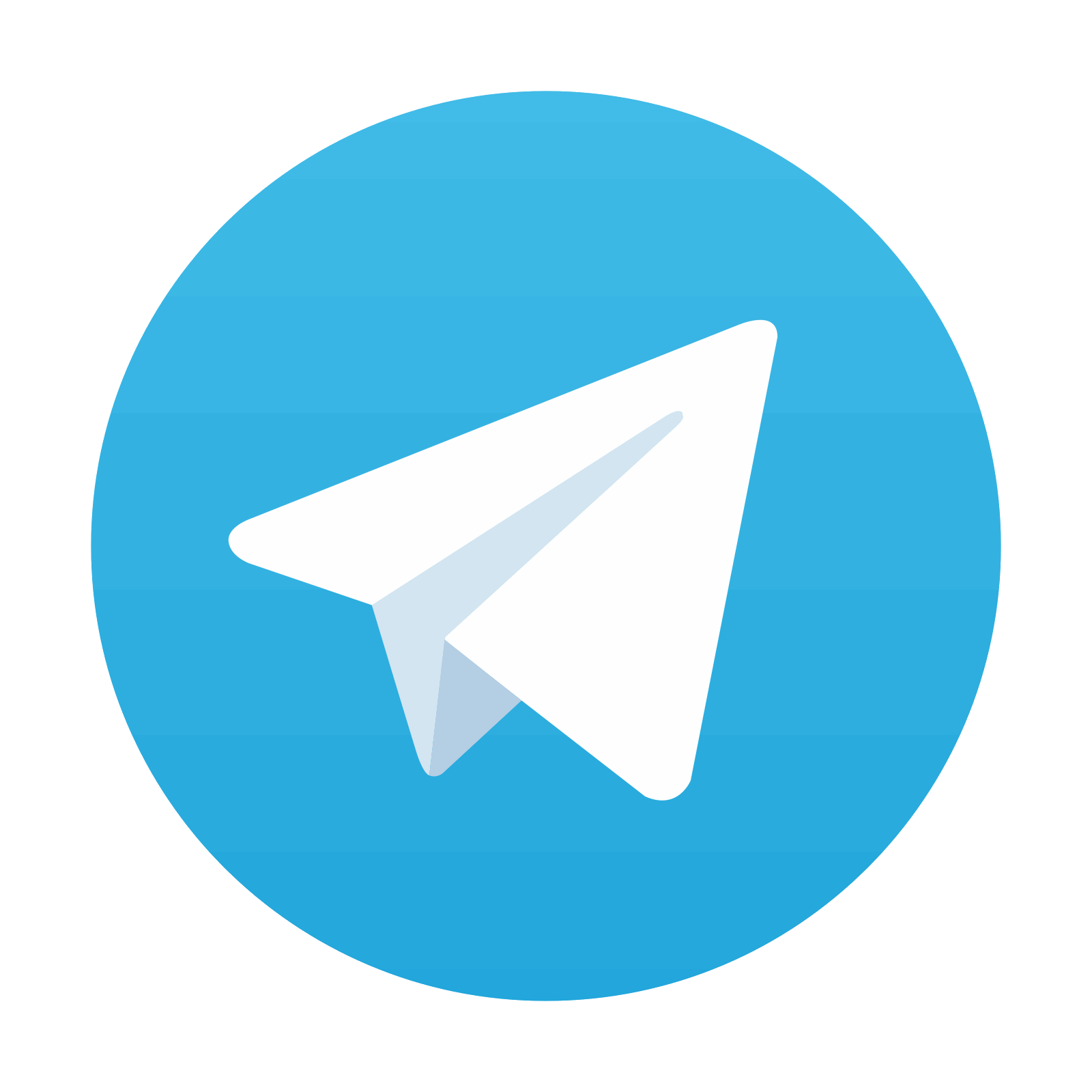
Stay updated, free articles. Join our Telegram channel

Full access? Get Clinical Tree
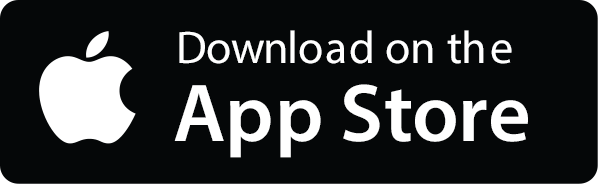
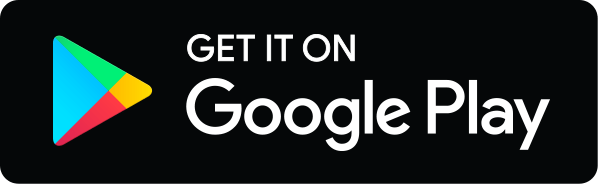