Hyperemia is a major criterion for the diagnosis of acute myocarditis on cardiac magnetic resonance imaging but its assessment is challenging and time consuming. We evaluated the usefulness of the contrast-enhanced first-pass perfusion (FPP) on magnetic resonance imaging for detecting subepicardial hyperemia in acute myocarditis. Forty-seven consecutive patients (mean age: 42 ± 15.6 years; 35 men) with a definite diagnosis of acute myocarditis according to the state-of-the-art guidelines were included and compared with 16 control subjects. FPP was evaluated by 2 blinded observers and compared with the reference late gadolinium enhancement. Detection of hyperemia was performed on both qualitative and quantitative methods. Relative increased signal intensity (SI) in the subepicardial hyperemic layer was measured with SI ratio (SI of the subepicardial layer/SI of the immediately adjacent subendocardial layer). Twenty-four patients (51%) with acute myocarditis exhibited subepicardial hyperemia, detected with a good interobserver reproducibility (kappa coefficient: 0.75). The SI in the myocardium of myocarditis patients was increased compared with controls (1.08 ± 0.03 vs 0.945 ± 0.04, p = 0.03) and the SI in myocarditis patients with hyperemia compared with those without hyperemia (1.22 ± 0.04 vs 0.94 ± 0.04, p <0.0001). Sensitivity, specificity, positive predictive, and negative predictive values of FPP for detecting hyperemia were 85%, 94%, 85%, and 93%, respectively. In conclusion, contrast-enhanced first-pass magnetic resonance imaging is a fast and useful method for assessing myocardial hyperemia in patients with acute myocarditis.
Hyperemia is one of the fundamental processes found in the acute phase of viral myocarditis and corresponds to a locally increased blood flow related to inflammation in response to the agent invasion. It is one of the major Lake Louise criteria counting in the diagnosis of acute myocarditis on cardiac magnetic resonance (CMR) imaging, but its assessment is challenging and time consuming. We hypothesized that the relative increase in blood distribution into the subepicardial layer of the inflamed myocardium may be detected as contrast-enhanced first-pass perfusion (FPP) images on CMR examination. The aim of our study was to detect and quantify hyperemia on contrast-enhanced FPP images in a series of patients presenting with acute myocarditis, with using late gadolinium enhancement (LGE) as the reference standard for myocardial injury including hyperemia.
Methods
This was a retrospective, single, tertiary center study. We reviewed all patients who were admitted for acute myocarditis from August 2012 to October 2014 and who met the following criteria: (1) CMR-based diagnosis of acute myocarditis using a modified Lake Louise criteria: presence of ≥2 of the 3 following criteria: edema seen on T2-weighted short tau inversion recovery (T2W-STIR) images, hyperemia seen on early contrast-enhanced steady-state free-precession (SSFP) images, and myocardial injury on LGE ( Figure 1 ); (2) CMR examinations performed within 10 days of presentation; (3) continuous chest pain >10 minutes with confirmation of myocardial injury revealing by an elevated troponin rate; (4) no history of ischemic heart disease; and (5) normal coronary arteries confirmed on coronary computed tomography or conventional coronary angiography. Control subjects were enrolled for comparison. The study was approved by the Ethics Committee, and informed consent was obtained from all the study subjects.
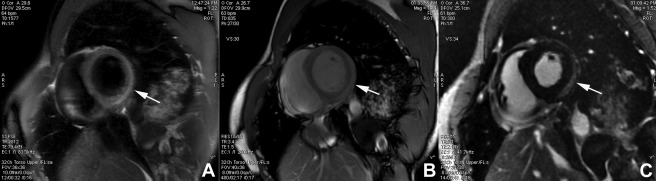
CMR examinations were performed using GE Healthcare systems (Milwaukee, Wisconsin) of 1.5 T (Signa HDxt system) or 3.0 T (Discovery MR 750). All patients underwent the following standard protocol ( Table 1 ): (1) location of the heart, (2) T2W-STIR images for assessing edema acquired in short-axis slices covering the whole heart, (3) cine SSFP images for assessing the left ventricle (LV) function acquired in 2- and 4-chamber slices, (4) FPP images with intravenous injection of 0.2 mmol/kg gadolinium chelate (Gadoteric acid; Dotarem, Guerbet, France) for assessing myocardial perfusion acquired in short-axis slices covering the whole heart, (5) early post-contrast cine SSFP for assessing both early myocardial enhancement and volumes and function of the 2 ventricles in short-axis slices covering the whole heart, and (6) LGE performed 7 to 10 minutes after the gadolinium chelate injection for assessing the global myocardial injury acquired in the earlier mentioned 2 and 4 chambers and short-axis slices covering the whole heart.
Sequence | 1.5 T | 3T |
---|---|---|
SSFP | RT: 3.8ms, ET: 1.3ms, FOV: 36 × 36, Slice thickness: 8mm, matrix: 220 × 220, Nex: 1, Flip angle: 45° or 60°. | RT: 3.3ms, ET: 1.5ms, FOV: 40 × 40, Slice thickness: 8mm, matrix: 224 × 224, Nex: 1, Flip angle: 45°. |
FPP | RT: 2.7ms, ET: 1.2ms, FOV: 40 × 40, Slice thickness: 10mm, matrix: 128 × 128, Nex: 1, Flip angle: 20° | RT: 2.4ms, ET: 1.1ms, FOV: 40 × 40, Slice thickness: 10mm, matrix: 128 × 128, Nex: 1, Flip angle: 20°. |
T2W-STIR | RT: 1800-2000ms, ET: 68ms, FOV: 35 × 26, Slice thickness: 10mm, matrix: 288 × 160, Nex: 1 | RT: 1800-2200ms, ET: 67.9ms, FOV: 38 × 38, Slice thickness: 10mm, matrix: 256 × 192, Nex : 1. |
LGE | RT: 7.7ms, ET: 2.1ms, FOV: 38 × 38, Slice thickness: 8mm, matrix: 256 × 160, Nex: 2, Flip angle: 15°. | RT: 5.1ms, ET: 2.1ms, FOV: 40 × 40, Slice thickness: 8mm, matrix: 256 × 192, Nex: 2, Flip angle: 15° |
CMR images were evaluated by 2 experienced observers as recommended by expert consensus. Endocardial and epicardial borders were traced semi-automatically using a dedicated cardiac software (QMass MR; Medis Medical Imaging System, Leiden, The Netherlands) on short-axis cine SSFP images covering the entire LV and right ventricle (RV) to measure end-diastolic and end-systolic LV and RV volumes indexed to body surface area (mL/m 2 ), ejection fractions of LV and RV (LVEF and RVEF %, respectively), and end-diastolic ventricular mass indexed to body area (g/m 2 ). The T2W-STIR, early contrast-enhanced SSFP, and LGE images were analyzed and localized in each of the 17 segments as recommended by the American Heart Association. Moreover, the global mass of LGE involving the LV was automatically quantified from short-axis stack using a signal intensity (SI) threshold of >5DS above the remote reference for normal myocardium, as previously described. LGE results were expressed as absolute value (g) and percentage of LV myocardial mass (%).
Analysis of the subepicardial hyperemia was made on a dedicated workstation (ADW 4.6; GE Healthcare) by 2 experts in cardiovascular imaging, blinded to clinical status (myocarditis patients or controls), and blinded to both early post-contrast cine SSFP and LGE images. First, qualitative assessment of hyperemia on FPP images was performed separately by each of the 2 observers, consisting on the visual detection of a relative hypersignal in the subepicardial layers on the 16 LV segments (exclusion of the apex). Second, the observers consensually made quantitative measurement of hyperemia. They consensually identified the segment presenting with the most evidence of subepicardial hyperemia and the image (phase) of the FPP sequence, which presented with the highest intensity in the hyperemic subepicardial layer. Then, 2 regions of interest (ROI) were consensually traced in the abnormal segment for measuring the myocardial SI: ROI 1 was traced in the hyperemic subepicardial layer and ROI 2 was traced in the immediately adjacent subendocardial layer ( Figure 2 ). SI ratio = SI of ROI 1 divided by SI of ROI 2 was calculated for evaluating the relative increase of blood flow in the hyperemic myocardium.
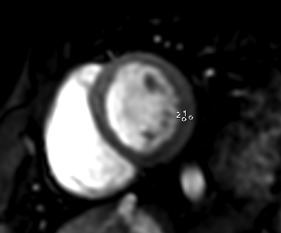
Statistical analyses were performed using JMP statistical software (version 9.0.1 (9.0); SAS Institute Inc., located at SAS Campus Drive, Cary, North Carolina). Continuous data were summarized as mean ± SD, and categorical variables were described with counts and percentage. Student’s t test was used for comparison of the quantitative variables between myocarditis patients versus controls and between myocarditis patients with hyperemia versus those without hyperemia. Before conduction t tests, the normal distribution of the quantitative variables using a Kolmogorov-Smirnov test was verified. Inter-reader agreement for the presence of edema, hyperemia, and LGE was assessed by the Cohen’s weighted kappa analysis with 95% confidence interval. The Kappa κ value were interpreted as 0.00, poor agreement; 0.21 to 0.40, fair agreement; 0.41 to 0.60, moderate agreement; 0.61 to 0.80, good agreement; and 0.80 to 1.00, excellent agreement. The sensitivity, specificity, and positive and negative predictive value of contrast-enhanced FPP images for detecting hyperemia were calculated in comparison with the reference LGE. For all statistical tests, p values <0.05 were considered to be statistically significant.
Results
A total of 50 consecutive patients with acute myocarditis were identified in our CMR database during the reviewing period. Of them, only 47 patients met the study’s inclusion criteria and constituted our final study population (3 patients were excluded because of incomplete CMR protocol). Sixteen healthy subjects were available as the control group. They consisted of diabetic patients, strictly selected for having no history of ischemic or inflammatory heart disease, with an anatomically and functionally normal heart on transthoracic echocardiography (TTE) and on stress myocardial perfusion with single-photon emission computerized tomography.
Clinical characteristics of patients and controls and results of their complementary examinations (electrocardiogram, laboratory, TTE, and CMR) are summarized in Table 2 . Seven patients (15%) with myocarditis were in critical condition, including 1 cardiogenic shock, 1 sustained ventricular tachycardia, 1 complete auriculoventricular block, and 4 severe congestive heart failure. Abnormalities of repolarization on electrocardiogram were frequent (39 patients, 83%) in myocarditis and involved predominantly the inferolateral segments of the LV. Myocarditis patients presented with evidence of increase of biologic markers of inflammation and myocardial injury. Compared with controls, myocarditis patients had a significant decrease of LVEF, increase of LV wall thickness, and experienced hypokinesia involving at least 1 segment. There was no significant difference in LV or RV volumes between myocarditis patients and controls.
Myocarditis (n = 47) | Controls (n = 16) | P Value | |
---|---|---|---|
Variables | |||
Man | 35 (74%) | 9 (56%) | – |
Age (years) | 42 ± 15.6 | 40 ±12.5 | 0.92 |
Body-mass index (kg/m 2 ) | 25 ± 4 | 24 ± 2.8 | 0.63 |
Body mass area (m 2 ) | 1.84 ± 0.2 | 1.8 ± 0.3 | 0.45 |
Diabetes mellitus | 1 (2.1%) | 16 (100%) | – |
Active smoking | 19 (40%) | 5 (31%) | – |
Recent infection | 29 (62%) | 0 | – |
Chest pain | 41 (87%) | 0 | – |
Functional NYHA class >II | 4 (8.5%) | 1 (2.1%) | – |
Systolic blood pressure (mmHg) | 123 ± 15.9 | 127 ± 16.3 | 0.8 |
Diastolic blood pressure (mmHg) | 74 ± 10 | 75 ± 9.7 | 1 |
Electrocardiogram | |||
Sinus rhythm | 46 (98%) | 16 (100%) | – |
Atrial fibrillation | 1 (2.1%) | 0 | – |
QRS complex width | 92 (21%) | 84 (11%) | – |
Bundle branch block (right or left) | 12 (26%) | 0 | – |
ST segment elevation | 19 (40%) | 0 | – |
Negative T wave | 20 (42%) | 1 (6.3%) | – |
Q waves | 1 (2.1%) | 0 | – |
Laboratory | |||
Peak of C Reactive Protein (μg/ll) | 60 ± 32 | – | – |
Peak of Troponin I (ng/ml) | 16 ± 35 | – | – |
Peak of Myocardial Band enzymes of Creatinin Phospokinase-MB (UI/l) | 542 ± 629 | 41 ± 104 | – |
Peak of Brain Natriuretic Protein (ng/ll) | 1476 ± 2270 | 110 ± 329 | – |
Echocardiography | |||
Left ventricle ejection fraction (%) | 55 ± 9.7 | 59.7 ± 5.1 | 0.01 |
Right ventricular systolic pressure (mmHg) | 27.4 ± 5.4 | 34 ± 12.2 | – |
Left ventricle posterior wall thickness (mm) | 9.5 ± 1.8 | 7.7 ± 2 | 0.002 |
Septal wall thickness (mm) | 9.9 ± 2.1 | 7.9 ± 1.9 | 0.001 |
Wall motion abnormality | 11 (23) | 0 | – |
Cardiac magnetic resonance | |||
Pericardial effusion >3mm | 19 (40%) | 0 | – |
Left ventricle end-diastolic volume (ml/m 2 ) | 75 ± 15 | 74 ± 12 | 0.77 |
Left ventricle end-systolic volume (ml/m 2 ) | 36 ± 13.1 | 32 ± 9 | 0.28 |
Left ventricle posterior wall thickness (mm) | 9 ± 1.3 | 7.1 ± 1.1 | 0.001 |
Septal wall thickness (mm) | 10 ± 1.6 | 8.1 ± 1.5 | 0.001 |
Left ventricle mass indexed (g/m 2 ) | 60 ± 13.2 | 54 ± 8.6 | 0.06 |
Left ventricle ejection fraction (%) | 54 ± 9.3 | 60 ± 6.8 | 0.009 |
Right ventricle end-diastolic volume (ml/m 2 ) | 69 ± 15.6 | 73 ± 9 | 0.52 |
Right ventricle end-systolic volume (ml/m 2 ) | 31 ± 10.7 | 31 ± 9 | 0.92 |
Right ventricle ejection fraction (%) | 56 ± 7.8 | 59 ± 8 | 0.13 |
Left ventricle late gadolinium enhancement (g) | 26 ± 21 | 0 | – |
Late gadolinium enhancement/left ventricle mass ratio (%) | 21 ± 16 | 0 | – |
Total of myocardial segments with late gadolinium enhancement | 2.7 ± 1.8 | 0 | – |
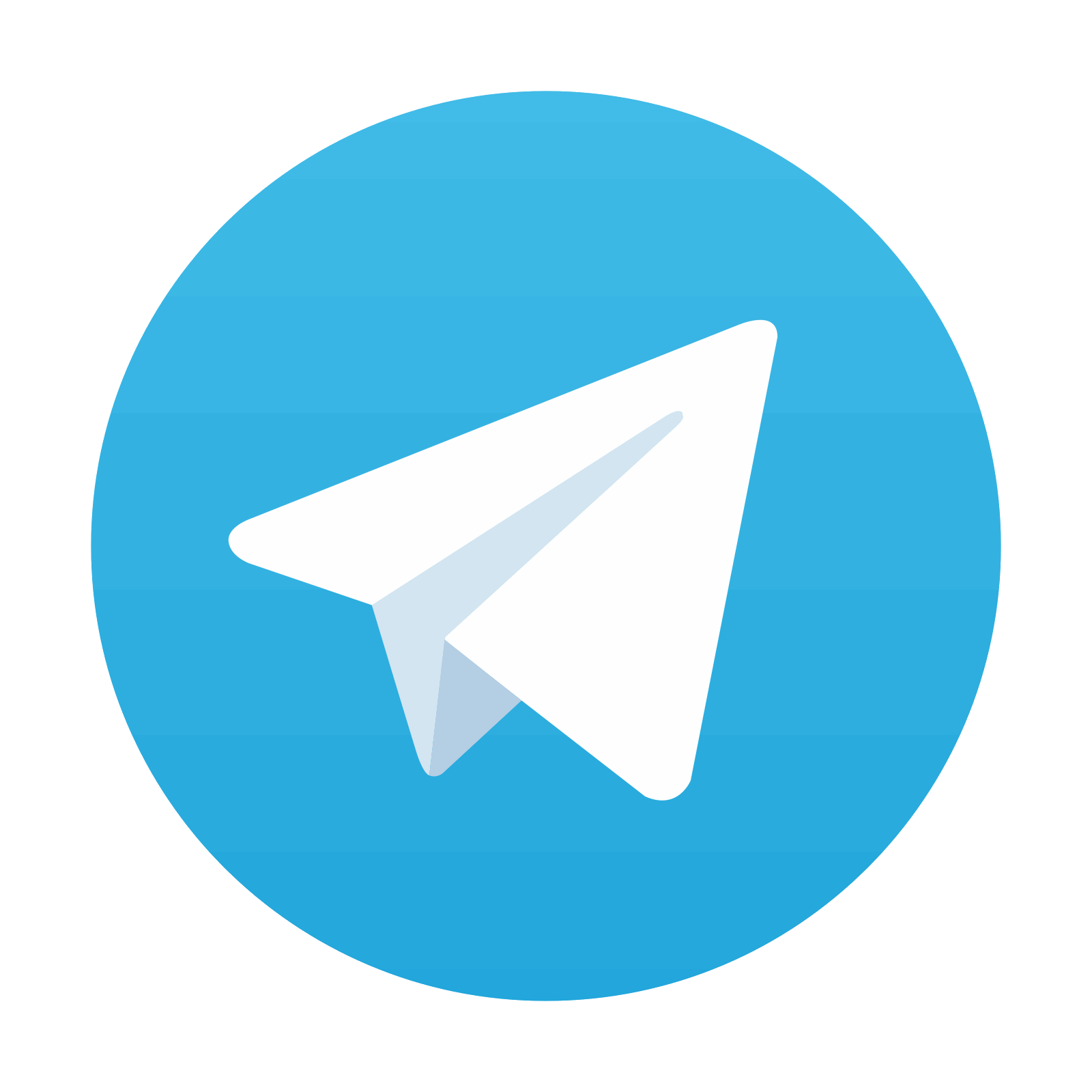
Stay updated, free articles. Join our Telegram channel

Full access? Get Clinical Tree
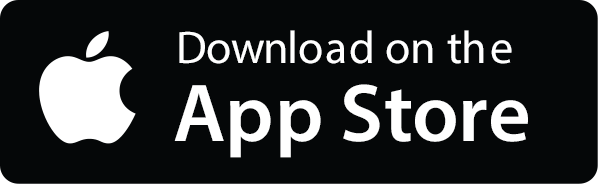
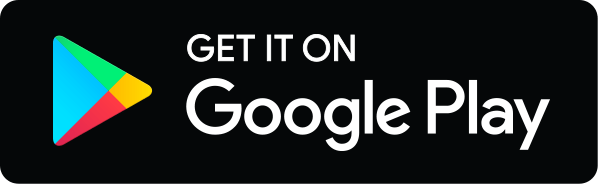
