Fig. 16.1
Table showing levels of evidence (I–V) for a primary research question
The failure to incorporate evidence, via EBM or otherwise, has led to the adoption of new technologies into clinical practice which later proved problematic. A recent example of new technology being widely accepted without extensive critical appraisal of evidence is the use of modular neck systems in THA. It has been reported that more than 30,000 THAs with modular neck systems have been implanted worldwide [14], and in the United Kingdom more than 6,000 various modular neck stems have been registered with the National Joint Registry [15]. The change from monoblock to modular stem–neck junctions was driven by the ability to make independent adjustments to the vertical and horizontal offsets, the leg length, and the version of the neck, which is especially advantageous in patients with complex anatomy. Evidence had shown that restoration of femoral offset and soft-tissue balancing can reduce abductor muscle imbalance, pain, and rates of wear [16]. Furthermore, the ability to adjust version and offset is useful in the prevention of impingement between the socket and neck, and bony and muscular impingement [17]. In a previous study of monoblock stems, femoral offset and limb length were not restored in 36 % of patients (28 of 79) [18]. At the time this technology was brought to market, in vitro studies suggested that an insignificant amount of corrosion and fretting occurred at the modular neck–stem junction [18, 19] and a similar study of the effect of machine surface finish on fretting and corrosion concluded that the degree of degradation was “within a clinically noncritical range” [20]. With this limited evidence, it appeared as though the benefits of modularity outweighed the potential risks. However, many studies have since reported that stems with increased modularity present an additional site for failure by introducing a second taper junction. These studies report failure mechanisms and increased failure rates of modular hip designs, the most common failure mechanisms being crevice corrosion and fretting corrosion.
Another example of the questionable introduction of new technology includes the use of intramedullary (IM) nailing for humeral shaft fractures. IM nails proved to be an effective method of treating femoral and tibial shaft fractures and became one of the preferred procedures in orthopedics. In general, IM nailing in the lower extremity is associated with high union rates and low complication rates. IM nails present a greater biomechanical advantage for construct stability over a plate and screws as a result of their location at the center of the bone. Compared to plate and screw fixation constructs placed on the cortical surface, biomechanical evidence continues to demonstrate that IM nails provide superior support for axial, bending, and torsional loads while stabilizing the fracture to promote healing. With sound evidence supporting the use of IM nails in lower extremity long bones, it was a logical transition to apply the same technology to the operative treatment of humeral shaft fractures. The use of IM nail fixation in fractures of the femur and tibia has been highly successful and has minimized many of the problems associated with plate fixation. Larger diameter, “locking” humeral nails were introduced in the hopes that they would overcome some of the shortcomings associated with early IM fixation devices or plates. The initial use of humeral nails appeared promising; however, conclusions were largely based on favorable retrospective reviews of the experience of experts with the implant, and these new devices were vigorously marketed for a wide range of indications [21–27]. Unfortunately, reports on the efficacy and success rates associated with the use of locking humeral nails have been inconsistent at best [28–31]. A wide range of complications have been reported, leading some authorities to question the role of these devices in the treatment of humeral shaft fractures. Data from randomized clinical trials directly comparing these implants to standard plate fixation have now emerged and the usage of humeral nails has significantly declined (Fig. 16.2) [32–34].
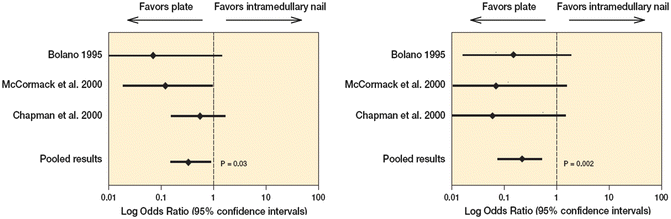
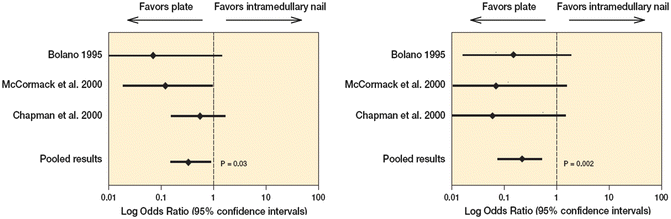
Fig. 16.2
(a) Statistical pooling of three studies (155 patients) revealed that plate fixation results in a significant reduction in reoperation rates (p = 0.03) as compared to intramedulary nail fixation. (b) Statistical pooling of 3 studies (155 patients) revealed that plate fixation results in a significant reduction in reoperation rates (p = 0.002) as compared to intramedulary nail fixation
An example of clinical decision making being transformed by the practice of EBM has been seen in the management of clavicle fractures. Clavicle fractures are common injuries accounting for between 2 and 10 % of all adult fractures [35, 36]. Mid-shaft clavicle fractures account for approximately 80 % of all clavicle fractures, and similarly to rib fractures, they have traditionally been treated non-operatively [37]. The nonoperative treatment strategy was based on early observational studies that suggested that clavicular non-union was rare. A study by Rowe reported the prevalence of four non-unions in 566 patients; a separate study by Neer reported only three non-unions in 2,235 patients [37, 38]. Furthermore, clavicular malunion was described as being of radiographic interest only, with no clinical importance [37–39]. However, more recent studies of displaced mid-shaft clavicular fractures have shown a non-union rate of 15 % (8 of 52 patients) in one series as well as unsatisfactory patient-oriented outcomes in 31 % of patients. Additionally, using an objective strength testing protocol for both maximal effort and endurance strength, deficits ranging from 10 to 35 % were found in patients a mean of 54 months after nonoperative care of a displaced fracture of the clavicular shaft [40].
This led to a number of randomized clinical trials that compared operative to nonoperative treatment of displaced fractures of the clavicle. In particular, a large multicenter RCT was conducted by the Canadian Orthopaedic Trauma Society (COTS) which included 132 patients with a displaced mid-shaft fracture of the clavicle. This study found that the Constant Score and DASH scores were significantly better at all-time points for the operative group of this study (p < 0.01) [41]. The COTS study also found that there were two non-unions in the operative group (3 %), significantly fewer than the seven non-unions in the nonoperative group (14 %) (p = 0.042) [41].
The choice to proceed with operative intervention for a displaced mid-shaft fracture of the clavicle remains a decision to be made between surgeon and patient. However, there is increasing evidence from well-designed level 1 prospective randomized trials that provide clear facts about the outcomes of treating clavicle fractures that can be used when counseling patients regarding treatment options.
The previous examples of modular necks in THA and the use of IM nails for humeral shaft fractures reinforce the principles of EBM that were characterized by Bhandari. The advancement of an existing procedure and the development of new technology were adopted into orthopedic practice prior to adequately and systematically applying EBM. These two examples show that integrating questionable evidence into a clinical approach, or going forward with an innovative technique prior to studying all of the relevant evidence, may cause more harm than benefit to the patient. It is important that orthopedic surgeons as a group do not rush into the treatment of unstable chest wall injuries and avoid following a similar path seen with previously unsuccessful innovation. Therefore, a careful evidence-based approach must be employed regarding the treatment of these injuries. The evidence currently available on management of these injuries is insufficient to determine whether fixation is an appropriate treatment, or whether it is more favorable than nonoperative treatment. The principles of EBM would dictate that such relevant information be attained and evaluated prior to adopting this new technique into clinical practice.
Unstable Chest Wall Injuries
Chest wall trauma is a significant cause of morbidity and mortality for trauma patients. An estimated 25 % of annual trauma deaths occur as a result of chest trauma [42]. Rib fractures are common injuries and occur in up to 39 % of patients with blunt chest trauma [2]. As many as one-third of patients with rib fractures require admission to hospital [42]. These fractures are routinely treated non-operatively, and many heal without major complications. However, a significant fraction (10–15 %) [3] of these injuries can cause chest wall instability, which has high rates of short-term mortality (up to 33 %) [2] and long-term morbidity. Unstable chest wall injuries can occur in cases with flail chest, and rib fractures with severe displacement or chest wall deformity. While surgical treatment of these injuries is technically possible, indications remain controversial and definitive studies have not been performed.
Unstable chest wall injuries can be caused by a flail chest, which is defined as fracture of three or more consecutive ribs in two or more places, creating a flail segment [42]. This definition also applies to (≥3) bilateral consecutive rib fractures, and (≥3) rib fractures associated with a sternal fracture, as both of these also lead to the creation of a flail segment [4]. These injuries severely injure the thorax and can lead to profound sequelae. A study by Velmahos et al. has shown that 73 % of patients with a flail chest who were not initially intubated developed respiratory failure and required intubation with mechanical ventilation [43]. Other causes of an unstable chest wall can be a crush injury that causes a “caved-in chest,” or severe displacement/overriding of the ribs. These can lead to chest wall deformity, loss of thoracic volume, and impaling of lung or intrathoracic structures by the fractured rib fragments [4].
Unstable chest wall injuries have been shown to have a high incidence of complications, including severe pulmonary restriction due to paradoxical movement of the flail segment, loss of lung volume, and inability to control pain despite maximal nonoperative measures (e.g., epidural catheter) [2–4, 9]. The resulting instability, decreased lung volumes, and pain lead to decreased pulmonary function and can leave patients dependent on long-term mechanical ventilation. Such long-term ventilation can lead to high rates of pneumonia, sepsis, tracheostomy, barotrauma, prolonged intensive care unit (ICU) stay, and high healthcare costs [2–4, 9–11]. Following discharge from hospital, patients often report long-term dyspnea and chest pain and have abnormal pulmonary function when assessed with spirometry [42].
The current and almost universal treatment of severe chest wall injuries consists of nonsurgical management via intubation and intermittent positive pressure ventilation (internal pneumatic splint), analgesia, pulmonary toilet, and chest physiotherapy [2, 4, 44]. However, such an approach may not produce optimal results. In addition to the complications listed above, studies have shown that only 43 % of patients return to their previous full-time employment [45], and many complain of chronic pain, subjective dyspnea, chest tightness, and chest wall deformity [45, 46] and have low scores on the SF36, indicating poor functional outcomes [47]. The current suboptimal outcomes following nonsurgical management of these injuries suggest that other approaches may be beneficial and that a large prospective clinical trial would be advantageous.
Unstable chest wall injuries are associated with compromised pulmonary function, substantial morbidity related to prolonged mechanical ventilation, and lengthy stays in the ICU and hospital [2]. At long-term follow-up, flail chest injuries are associated with dyspnea in up to 63 % of patients, chest pain in up to 49 %, and long-term disability in up to 64 %, with abnormal spirometry results in up to 57 % [45, 46]. These injuries are associated with the consumption of a significant amount of healthcare resources when treated non-operatively. Yet, nonoperative care has been the gold standard, in part, because rib fractures do heal without surgery, and in part, related to concerns around surgical fixation including potential complications such as infection, injury to neurovascular structures, lung injury, and the need for subsequent surgery to remove implants [2]. Perhaps one of the largest barriers to surgical treatment is the fact that most surgeons are unfamiliar with the procedure. While nonoperative care of these injuries is almost universal, there have been a limited number of retrospective and long-term studies in the thoracic surgery and trauma literature that have demonstrated the benefit of surgical fixation of severe chest wall injuries when compared to conservative treatment and suggest that this treatment may be cost-effective. This benefit includes decreased days on mechanical ventilation (3.7–10.8 vs. 15–30.7) [10, 11, 48], decreased days spent in ICU (6.8–16.5 vs. 21–28.3) [3, 9–11], decreased chest infections (7.6–24 vs. 50–77 %) [9–11], earlier return to work (95–100 vs. 43 %) [11], decreased chronic pain (5.5–11 vs. 49 %) [3], and decreased long-term respiratory dysfunction [49, 50].
A randomized controlled trial by Tanaka et al. showed that on average, patients with surgical fixation were extubated 2.5 days postoperatively, compared to 18.3 days of intubation in the nonoperative group [11]. In a similar study, Granetzny et al. demonstrated the total days of mechanical ventilation to be 2 days in the surgical group and 12 days in the nonsurgical group [9]. While generating considerable interest in internal fixation, these trials have significant methodological limitations including small sample sizes, lack of modern and standardized nonoperative treatments, as well as the use of variable and outdated methods of rib fracture fixation. Very recently, a randomized controlled trial by Marasco et al. [12] used modern and standardized nonsurgical treatment of these injuries compared to surgical treatment. This study demonstrated reduced ICU stay and use of tracheostomy in the operative group but no differences in duration of mechanical ventilation, spirometry at 3 months, or quality of life at 6 months. This trial also had significant methodological limitations including small sample size, significant delays to randomization and operative treatment, and the use of unconventional implants for rib fixation.
There continues to be marked uncertainty around the management of acute unstable chest wall injuries. We recently completed a retrospective analysis of the injury patterns, management, and clinical outcomes associated with flail chest injuries at 199 North American trauma centers over a 3-year period and found that only 1 % of these injuries received surgical fixation. Yet, a recent survey of 405 general, orthopedic, and thoracic surgeons revealed that 82 % of the general surgeons, 66 % of the orthopedic surgeons, and 71 % of the thoracic surgeons felt that operative repair of rib fractures was indicated in selected patients. Only 22 % of respondents were familiar with a randomized trial in this area [2, 51]. Of those who felt that operative treatment was not indicated, 82–95 % felt that a definitive randomized trial was necessary to change their opinion [51].
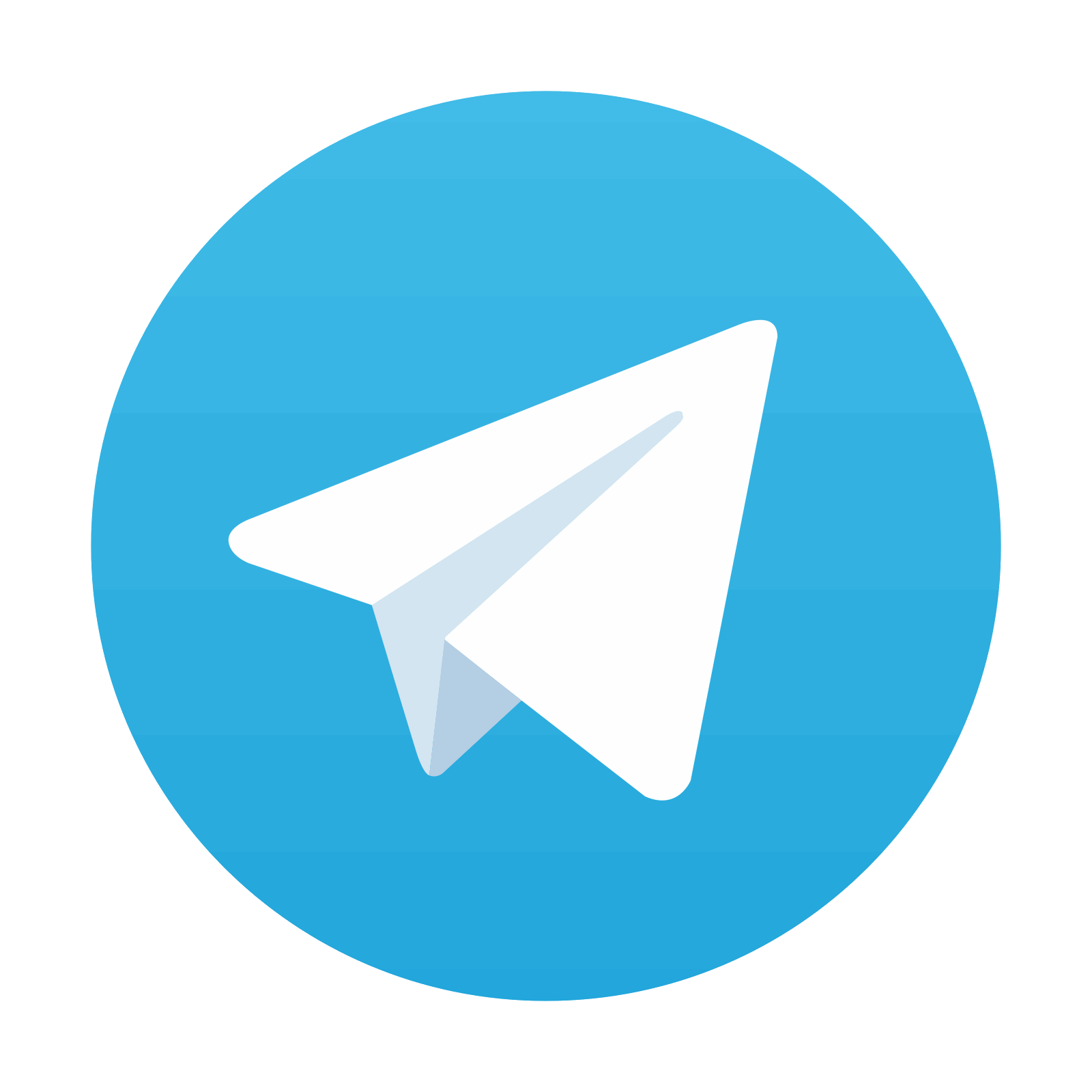
Stay updated, free articles. Join our Telegram channel

Full access? Get Clinical Tree
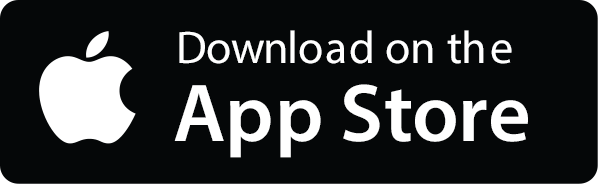
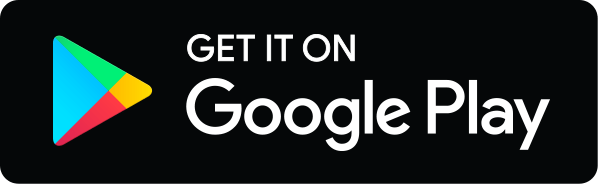