Fig. 6.1
Rib loading during normal respiration was measured by Rehm et al.’s [7] finding that bending is the dominant loading mode
For the biomechanical evaluation of the stability of a rib fixation construct, two tests are desirable: A dynamic test for at least 360,000 loading cycles at or above respiratory loading and a static test to failure to determine the construct strength. The latter test will also reveal the failure mode, which is caused by implant breakage, fixation failure, or rib fracture adjacent to the implant. While simplified biomechanical tests are crucial to detect and eliminate design flaws, results cannot predict clinical performance due to several inherent limitations. For example, biomechanical testing cannot replicate complex in vivo loading, and it cannot account for secondary stabilization due to callus formation, adjacent ribs, and/or intercostal soft tissues. Therefore, prospective clinical studies are an essential complement of biomechanical studies in order to assess implant function in the clinical realm. Despite the obvious need for combined biomechanical and clinical evaluation of implants, this strategy remains rare at best among the many implants that have been employed for rib osteosynthesis.
Ribs Are Highly Elastic
Unlike most long bones that have a cylindrical cross section to maximize bending rigidity, ribs have a pronounced oval, tear-shaped cross section [10] and nonuniform cortex distribution [11] to maximize elastic flexion in bending (Fig. 6.2). The rib cross section is on average approximately half as wide as high [10]. Moreover, the anterior and posterior cortices are considerably thicker than the superior and inferior cortices, which are on average only 0.5 mm thick [10]. Owing to their oval cross section, thin inferior and superior cortices, and curved structure, ribs can elastically bend more than any other skeletal structure. Schultz et al. demonstrated that 0.75 kp loading caused 3–8 cm deflection of ribs 4–10 without inducing failure [12]. In a more drastic description, Fick stated that the sternum in children can be compressed up to the spine without inducing a rib fracture [13].
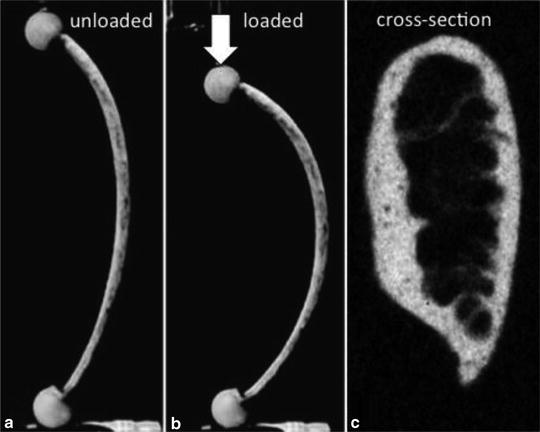
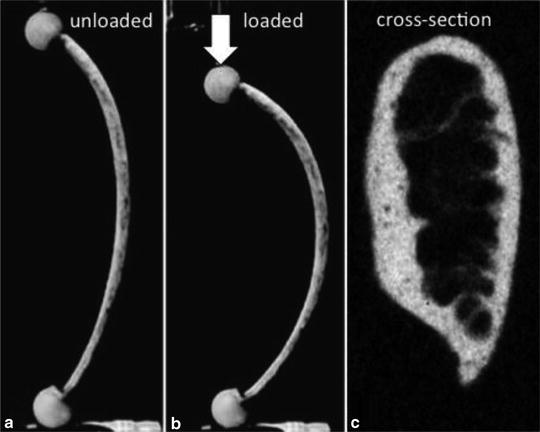
Fig. 6.2
The same rib is shown relaxed (a) and loaded (b). Ribs can elastically flex more than any other long bone due to their pronounced oval cross section and nonuniform cortex distribution. (c) This cross-section of a rib demonstrates it’s oval nature
Accounting for the high bending elasticity of ribs is of crucial importance for implant design. If a rib plate is stiffer than the rib itself, it will resist bending and will induce high stresses at the plate end (Fig. 6.3). This in turn will either lead to pullout of the outermost screws, or to rib fracture through the outermost screw hole [7, 14, 15]. Since the bending strength of ribs decreases by approximately 50 % from age 50 to 80 [16], prevention of stress risers caused by overly stiff implants becomes an even greater concern in the elderly population, particularly in patients with osteopenic bone. From a clinical perspective, implants designed for normal bone may not perform reliably in osteopenic bone. From a biomechanical perspective, it is therefore crucial that rib implants are tested not only in specimens representative of normal bone quality but also in osteopenic rib specimens.
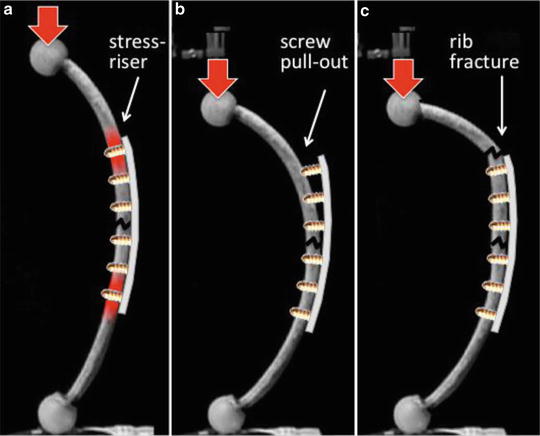
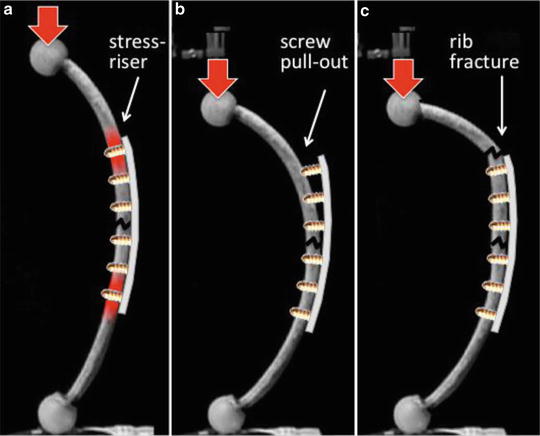
Fig. 6.3
Stiff implants will resist bending and induce stress risers at the plate end (a), leading to screw pullout (b) or rib fracture (c) at the plate end
Ribs Are Thin
The average width of ribs 4–9 reported for 5 human cadavers ranges from 6.0 to 7.6 mm [10]. Approximately 2/3 of this width is comprised of the intramedullary canal that provides little structural support for implant fixation. Among ribs 4–9, the average thickness ranges from 0.9 to 1.4 mm for the inner cortex and from 0.7 to 0.9 mm for the outer cortex. Hence, bicortical screws have on average less than 2 mm of cortex thickness to gain secure and durable purchase. Since the holding power of conventional screws is directly proportional to the length of engagement in cortical bone, it becomes apparent that screw stripping during insertion and screw loosening or pullout after insertion were common modes of failure before the advent of modern locking plates [15, 17, 18]. Intramedullary fixation with Kirschner wires can overcome this limitation by providing a line contact between the implant and the rib canal, thus distributing loads more evenly, rather than concentrating load transfer to discrete screw fixation points. However, Kirschner wires are also prone to migration [19–22] and cutting out through the cortex in the presence of osteoporotic bone, which can have serious negative clinical consequences [17, 23].
Ribs Have a Complex Geometry
The principles of fracture fixation with plates and screws require that a rib plate traces the rib diaphysis and remains in contact with the rib surface over the entire plate length to achieve a durable fixation construct [24]. Fitting a short plate for fixation of a single rib fracture can readily be accomplished by out-of-plane bending to the general curvature of the rib. However, contouring a longer plate for bridging of a comminuted fracture or multiple fractures is complicated for three reasons [25]: First, the outer surface of a rib is not part of a cylinder but rather conical [10]. Therefore, when bending straight plates towards the rib surface, longer plates tend to diverge off the rib (Fig. 6.4a). Second, fixation of a flail segment often requires long plates that can span the fractures on both ends of the unstable rib segment [26]. With increasing length, plates are more likely to deviate from the narrow rib surface. Finally, the rib surface is twisted around the rib longitudinal axis [10], requiring twisting of plates [15]. Despite this complexity, contouring of plates to the rib surface is important since deficient contouring may lead to suboptimal fixation and patient discomfort due to prominent hardware [17]. Consequently, intra-operative contouring of plates to the rib surface can be difficult and time-consuming and may yield suboptimal plate fit and fixation strength [15, 24].
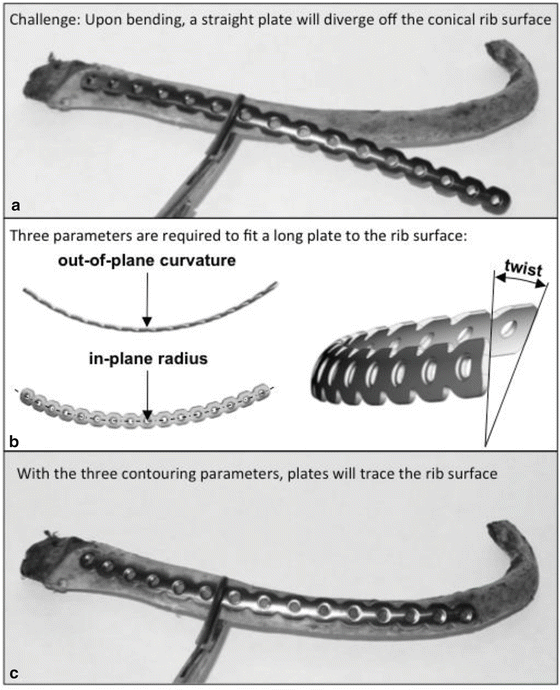
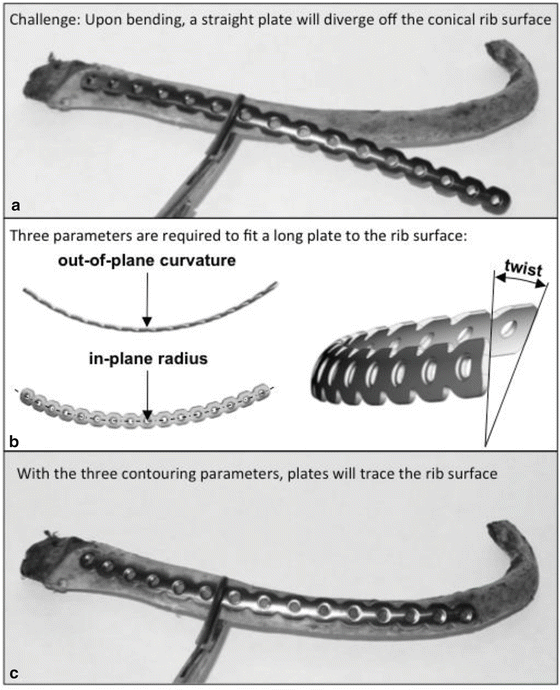
Fig. 6.4
To avoid the typical plate diversion from the conical rib surface (a), a plate must have the appropriate “in-plane” radius and twist in addition to the “out-of-plane” curvature (b). After accounting for these three contouring parameters, a long plate will trace the rib surface (c)
A recent biomechanical analysis of ribs delineated the complex rib surface geometry into three basic parameters pertinent for plate contouring, namely the general “out-of-plane” curvature, the conical contour, and the twist characteristic for ribs three through nine (Fig. 6.4b, c) [10]. The general curvature ranges from 3.8 ± 1.5 m−1 at the lateral aspect of rib 7 to 17.3 ± 1.7 m−1 at the anterior portion of rib 3. The conical contour of the rib surface requires rib plates to be contoured with an in-plane radius of 20–40 cm in order to trace the rib surface upon plate bending to the general rib curvature. Finally, rib surfaces have a characteristic twist of 40° to 60°. Left ribs are twisted clockwise and right ribs are twisted counterclockwise, for which reason plates contoured to a right rib surface may not fit the contralateral left rib. Clinically, in fracture situations where a long plate is required, anatomically pre-contoured rib plates may not only reduce the time and complexity of the operative procedure, but may also support durable, low-profile fixation to minimize implant-induced discomfort in the presence of a thin soft-tissue envelope. For fracture patterns where a shorter (6–8 hole) plate is sufficient, simpler plate designs may suffice.
Rib Fractures Can be Complex
Implants for rib osteosynthesis have to accommodate a wide range of fractures. Since the extent of rib fractures is generally underestimated on radiographs, a preoperative CT scan is recommended to better assess the extent and type of fractures [27]. Intramedullary fixation or short plates can stabilize a simple fracture in a less-invasive manner, but may not be able to bridge and stabilize a comminuted, unstable fracture zone. Long bridging plates that can suspend a flail segment by spanning both associated fractures have been considered fundamental for operative stabilization of a flail chest [26, 28]. Long pre-contoured plates can furthermore serve as a reduction template to restore the physiologic geometry of a comminuted fracture zone or flail segment. From this consideration, it appears unlikely that a single fixation device can satisfy the competing demands for less-invasive fixation of simple fractures and spanning fixation of comminuted fracture or flail segments.
In summary, implants for rib fixation should ideally be strong enough to support peak loading, sufficiently elastic to prevent stress risers, able to provide durable fixation in the presence of thin, osteoporotic ribs, anatomically contoured to eliminate the complexity of intra-operative implant bending, and able to stabilize simple as well as comminuted fractures. Given this list of essential implant requirements, it becomes apparent why many prior implants fell short of expectations and hindered the adoption of surgical fixation as an effective clinical tool for management of rib fractures. Furthermore, it becomes evident that dedicated implants for rib fracture fixation have many potential clinical advantages.
Historic Approaches
This review focuses on extramedullary and intramedullary implants for rib fixation. It does not include wiring techniques or external stabilization with fixators and elevators, which have been abandoned due to their lack of practicality and their inability to provide consistent and pain-free stabilization.
Extramedullary Fixation
Historically, three different modes for fixation of plates to ribs have been used, which are wire or suture cerclage, screw fixation, and elastic claws. Early rib plates were applied with sutures or cerclage wires. The 1917 Surgery Manual of Bier et al. describes the application of Hansmann’s bone plate for stabilization of simple, transverse rib fractures using trans-cortical sutures (Fig. 6.5a) [29]. In 1972, Paris et al. introduced rib struts that were up to 40 cm long to span and suspend multiple flail rib segments [30]. These struts were applied along the rib, between ribs, or across ribs using sutures and were routinely removed upon fracture healing (Fig. 6.5b). Low-profile plates for cerclage fixation that did not require routine removal were introduced by Thomas in 1978 [31] and by Vecsei in 1980 [32] (Fig. 6.5c). Both plates had lateral slots to prevent cerclage wire slippage. In addition, the Vecsei plate had small surface spikes to prevent slippage of the flat plates on the rib surface. The flat cross-sectional profile of the plate (1 mm × 14 mm) precluded in-plane contouring; therefore, these plates were only available up to a length of 8 cm and did not allow bridging of a flail segment. Furthermore, tightening of cerclage wires could cause cortical transection and poses a risk to the neurovascular structures at the inferior aspect of ribs [7].
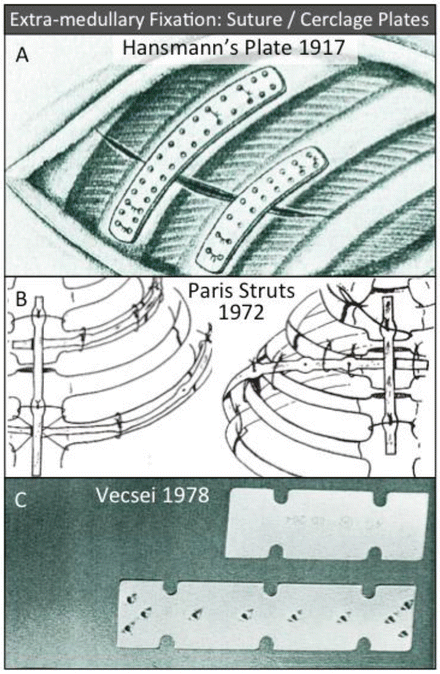
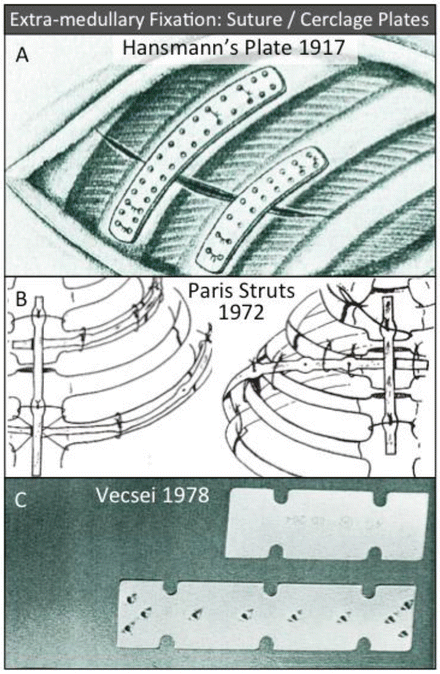
Fig. 6.5
Early plates and struts were attached to ribs with wire or suture cerclage
With the introduction of standardized implants and instruments by the Arbeitsgemeinschaft für Osteosynthesefragen (AO), standard small fragment, 1/3 tubular, and reconstruction plates quickly became the most frequently used plates for rib fracture fixation [33]. These standard plates accommodate in-plane contouring and provide sufficient stability to suspend a flail segment. However, it quickly became apparent that these plates were too stiff and caused stress concentrations that contributed to screw pullout, most prominently of the screws at the plate end, or total loss of fixation [15, 17, 18, 33]. It is important to note that screws at that time were not self-tapping, non-locking, and that tapping of cortical screw holes was not routinely performed. Furthermore, Labizke stated that standard plates required bending and twisting in this situation, making their application technically demanding and time-intensive [15]. For plate contouring of standard plates, Oyarzun recommended that the rib fracture should be reduced first, a template should be contoured to the rib surface, and a plate should be contoured to the template using bending irons, pliers, or a bending press [24]. This complexity frequently required a team approach, whereby the general/thoracic surgeon provided the operative exposure and collaborated with the orthopedic service for plate fixation [3].
The limitations common to generic plates prompted a subsequent generation of rib plates that utilized claws for fixation, with the hope of providing better fixation than screws and simpler fixation compared to cerclage techniques [15, 34]. The Judet plate (1973) had clawed end sections and a flat central section of 5.3 cm length suitable for spanning a single fracture [34, 35]. It was only 0.7 mm thick, which facilitated bending of the claws to the rib using a special forceps (Fig. 6.6a). The Labitzke claw plate was the first rib plate specifically designed to accommodate in-plane bending [15]. Its “self-gripping” claws also allowed supplemental fixation with 2.7 mm screws (Fig. 6.6b). It was sufficiently flexible to closely conform to the conical surface over long rib sections. While the Labitzke plate could span both fractures of a flail rib segment, its high flexibility necessarily limited the ability to suspend a flail segment. Moreover, since claw fixation required soft-tissue stripping to provide an adequate grip for the claws, the long and continuously clawed Labizke plate required extensive soft-tissue denudation along the rib [7]. Stating the fundamental need for rigid spanning of a flail segment, Sanches-Lloret introduced 13–19 cm long rib struts with clawed end sections to bridge fractures on each side of the flail rib segment with a single implant (Fig. 6.6c) [26]. The circular midsection of the struts accommodated contouring but lacked the low profile of plates.
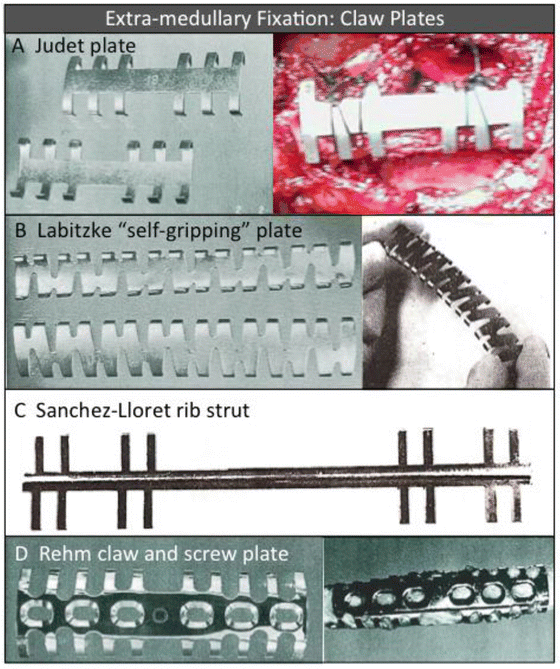
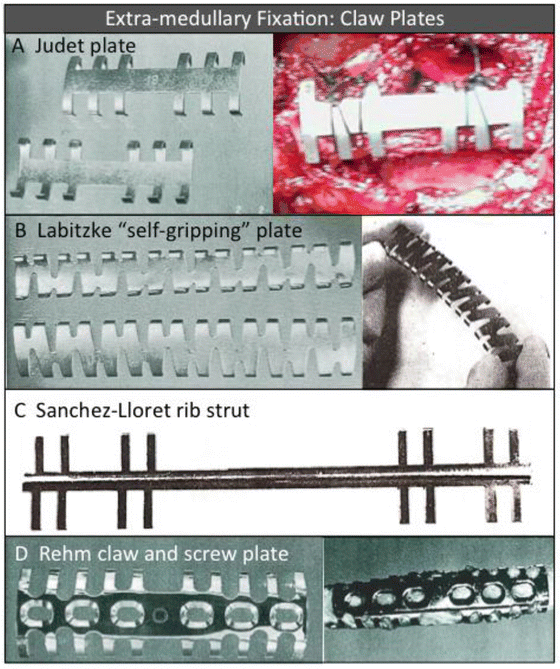
Fig. 6.6
Rib plates with “claw” fixation were introduced to overcome poor fixation of generic plates with (non-locking) screws
In an extensive biomechanical evaluation of rib plates, Boetsch and Rehm documented that rib fixation with Judet, Vecsei, and Labizke plates restored only 21 %, 23 %, and 8 %, respectively, of the strength of the native rib due to their thin plate cross section and poor fixation [36]. Conversely, they found that overly stiff conventional plates induced screw pullout and rib fracture at the plate end. They concluded that a plate should be sufficiently flexible to prevent stress risers at the plate end, yet sufficiently strong to withstand loading. Given an adequate stiffness, they furthermore concluded that longer plates yield stronger fixation constructs. Based on their findings, they developed a novel rib plate that combined claw and screw fixation options (Fig. 6.6d). Their plate was 70 mm long, straight, and tapered toward the ends to reduce stress concentrations.
Most interestingly, in 1986 Rehm also developed and tested the first resorbable rib plate [7]. The 6-hole plate was 5 mm thick, made of Polyglactin, and accommodated 3.5 mm cortical screws. Their initial approach to use resorbable screws was abandoned due to screw breakage during insertion. Static and dynamic testing of resorbable plates applied with stainless steel screws delivered encouraging results, yielding stiffness and strength values in line with the native rib. However, in an animal study, plates had completely dissolved prior to their first 6-week evaluation point. Their experience emphasizes the fact that encouraging biomechanical results cannot be extrapolated into the clinical realm without in vivo data.
Intramedullary Fixation
Intramedullary fixation of rib fractures with Kirschner wires has been successfully performed throughout the past 50 years [37]. Kirschner wires serve as splints to hold a fracture or flail segment in a more anatomic position and prevent paradoxical motion without obtaining rigid fixation (Fig. 6.7a). In this context, it is important to note that rib fractures do neither require perfect reduction nor absolute stabilization to generate “direct” bone healing, which resembles direct but slow remodeling across a perfectly reduced fracture site. Due to abundant blood supply, rib fractures heal spontaneously by normal (“secondary”) fracture healing, which is faster and stronger than primary bone healing, and which is stimulated by small interfragmentary motion [38]. Accordingly, Voggenreiter stated that “the purpose of operative chest wall stabilization is not a totally stable osteosynthesis, and primary fracture healing is not worthwhile” [18]. This fact supports less-invasive, intramedullary rib fixation, whereby fractures neither need to be completely exposed nor perfectly reduced. This approach not only reduces the surgical complexity but also adheres to the principles of modern biological osteosynthesis that aims to minimize soft-tissue injury, particularly the periosteum from which the fracture callus forms.
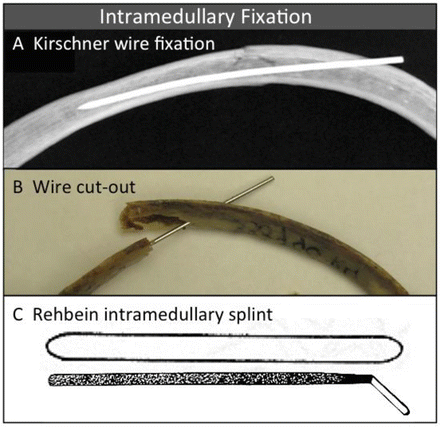
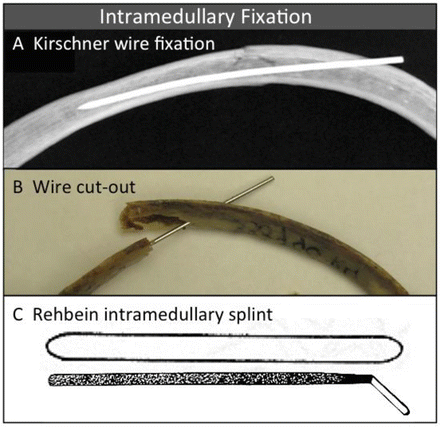
Fig. 6.7
Intramedullary fixation of rib fractures with Kirschner wires (a) is prone to cutout (b). Rehbein splints (c) had improved cutout resistance and rotational stability
Compared to plating, intramedullary fixation of rib fixation with Kirschner wires has several important benefits. Kirschner wires can be inserted through smaller incisions in a less-invasive approach, requiring less resection of intercostal soft tissue than plating [39]. Kirschner wires follow the canal shape upon insertion, while plates require intra-operative contouring to match the rib surface [17, 24]. Intramedullary implants are better tolerated than plates that remain on the surface of the rib and require removal due to persistent discomfort in 5–15 % of cases [40, 41]. Furthermore, intramedullary implants derive fixation strength by confinement in the rib canal, while plate fixation can be prone to screw loosening and plate pull-off, especially in the presence of osteoporotic bone [17, 42]. Most importantly, intramedullary implants allow fixation of posterior rib fractures, where access for plating can be severely restricted by the scapula and latissimus dorsi [43]. The clinical efficacy of rib osteosynthesis with Kirschner wires has been described in a number of case series that reported good to excellent results [19, 37, 39, 44, 45]. In 1972 Dor presented his first 100 cases of flail chest stabilization with Kirschner wires and described a mortality rate of only 16 % in severely injured patients [45]. After two decades of experience in rib fracture fixation with Kirschner wires, Samarrai and associates concluded that this technique had given the most satisfactory results when compared with other fixation techniques [39]. While the clinical utility of intramedullary fixation of rib fractures has been well established, there are two persistent limitations. First, the thin and circular cross section of Kirschner wires provides poor rotational stability and is prone to cutting out through the cortex, especially in the presence of osteoporotic bone (Fig. 6.7b) [17, 23]. Second, Kirschner wires can dislodge and migrate, which may cause discomfort, loss of fixation, or harm [19, 21, 34, 43]. Cases of intraspinal and intracardiac migration of Kirschner wires from ribs were reported as recently as 2012 [20] and 2014 [22], respectively. Albrecht and associates reported abandoning the use of Kirschner wires because of their migration risk and propensity for cutout, despite their relative ease of use and sufficient stabilization [43]. At the present time, with the availability of superior intramedullary devices, the use of Kirschner wires alone for rib fracture fixation is not recommended.
To address these persistent problems, the Rehbein plate, an intramedullary plate with a rectangular cross section designed to provide improved rotational stability, was introduced in 1972 (Fig. 6.7c) [46]. One end of the plate was designed to be left out of the canal and was sutured to the rib to limit migration. Nevertheless, it remains unclear how these rectangular Rehbein plates were reliably inserted and advanced through the fracture site along the intramedullary canal. Rush pins were also proposed in place of Kirschner wires [37]. Interestingly, no biomechanical or clinical studies on the performance of Rehbein plates or Rush pins exist. Even more surprising is the fact that until most recently, Kirschner wire fixation of rib fractures has neither been formally evaluated or improved despite 50 years of clinical experience that revealed both its potential and its obvious complications.
Contemporary Implants for Rib Fracture Fixation
This section provides an overview of currently available implants for rib fracture fixation, describes their features aimed at addressing the five challenges inherent to rib fracture fixation discussed earlier, and summarizes biomechanical and clinical evidence of their performance.
To date, four types of implants for rib fracture fixation exist: the MatrixRIB anatomic plate and splint system, the StraTos clip and bar system, the RibLoc U-plate system, and resorbable implants (including Biobridge). In contrast to historic implants described in the previous section, all contemporary metallic implants are made from titanium alloy rather than stainless steel. Titanium implants are approximately twice as flexible but comparable in strength to stainless steel implants, for which reason titanium has become the material of choice to support elastic fixation of rib fractures.
MatrixRIB System
This system combines anatomic plates and intramedullary splints to provide a comprehensive solution that accommodates the large variety of fracture patterns and anatomic locations encountered in complex flail chest injuries (Fig. 6.8a, b). Specifically, it consists of a set of anatomically contoured rib plates that contains four left and four right plates of 300 mm length, which suffice to accommodate the surface geometry of ribs 3–9, as well as short 6-hole plates for fixation of simple fractures. Long plates allow bridging of comminuted fractures and spanning of multiple fractures to suspend flail segments. Plates may be cut intraoperatively to the desired length. All plates are made of titanium alloy and are 1 mm thick to allow for elastic, low-profile fixation. Screw holes of these locking plates are threaded to engage with correspondingly threaded heads of locking screws. This eliminates screw stripping during insertion and provides improved fixation strength compared to standard screws (Fig. 6.8c). For intramedullary fixation of simple, isolated fractures in a less-invasive manner, the MatrixRIB system contains pre-contoured intramedullary splints that have a rectangular cross section to provide torsional stability and resistance against cutout. Splints are secured to the rib with a single locking screw to prevent migration. The splint tip is tapered and sloped to facilitate splint insertion along the medullary canal.
