Tricuspid Atresia/Single Ventricle and the Fontan Operation
Stephanie Fuller
Pradeep Kaushik
INTRODUCTION
Atresia of any heart valve, atrioventricular (AV) or semilunar, results in single-ventricle physiology. A variety of anatomic defects should be considered as a single ventricle from the standpoint of surgical interventions (Table 95.1). Patients born with a single functional ventricle have a dismal long-term prognosis without eventual surgical intervention. But the results of staged surgical palliation, culminating in the Fontan procedure as the final palliative surgical procedure for these patients, are generally quite good.
CLASSIFICATION OF SINGLE-VENTRICLE ANATOMY
The classic defect for which the Fontan operation was first performed is tricuspid atresia. In this defect, there is no identifiable tricuspid valve tissue or valve remnant. Instead, the floor of the right atrium is completely muscular, and the floor is separated from the ventricular mass by the fibrofatty tissue of the AV sulcus. Generally, in association with the atretic right AV valve, there is a total absence of the inlet and varying portions of the trabecular portion of the right ventricle.
There is almost always an associated ventricular septal defect (VSD), which is frequently restrictive. The great vessels may be transposed with the aorta arising from the infundibular chamber or normally related with the aorta arising from left ventricle (LV). The VSD is frequently restrictive, which results in restriction of pulmonary blood flow when the great vessels are normally related but results in subaortic stenosis when there is transposition of the great arteries (TGA). When transposition is present, even if the bulboventricular foramen (BVF) is initially large and unrestrictive, with time it can be expected to become smaller, resulting in subaortic stenosis later in life. If there is a coarctation present, then it is very likely that the BVF will be restrictive in the newborn period.
The natural history in tricuspid atresia is quite variable, but it is predicted by the underlying pathophysiology as in all single-ventricle hearts. Most patients with tricuspid atresia and normally related great arteries have some degree of obstruction to pulmonary blood flow. This generally progresses as the VSD becomes more obstructive or infundibular obstruction increases. As a result, there is increasing cyanosis. Without surgical intervention, over 90% of patients will die by 1 year of age from complications of hypoxia. Early palliation is, therefore, advocated for every neonate with tricuspid atresia. In the neonatal and early infantile period, when pulmonary vascular resistance (PVR) is high and pulmonary blood flow is inadequate, a systemic-to-pulmonary artery (PA, modified Blalock-Taussig) shunt is performed.
In contrast, those patients with tricuspid atresia and ventriculoarterial discordance (associated TGA) generally have a worse prognosis. Unobstructed pulmonary blood flow results in congestive heart failure and death within the first year of life. Subaortic obstruction complicates the scenario, further shortening the survival time. In the extreme case, there is ductal dependency of the systemic circulation in the newborn. In the case of excessive pulmonary blood flow, it is imperative to limit the pulmonary blood flow, usually with a PA, to protect the patient from developing pulmonary vascular disease and ventricular dysfunction caused by chronic volume overload.
Some children with single ventricles who present in infancy or later develop systemic ventricular outflow tract obstruction (subaortic stenosis). The resultant myocardial hypertrophy can adversely affect the suitability for a Fontan procedure. Patients at risk are those in whom the aorta arises above a small outlet chamber, such as in tricuspid atresia with double-inlet LV, rudimentary right ventricle, and TGA, particularly if the VSD is small or if there is coexisting aortic arch obstruction. A modified Damus-Stansel-Kaye procedure should be performed to establish unobstructed systemic arterial outflow.
PLANNING FOR SINGLE-VENTRICLE PALLIATION
Because the ultimate success of the Fontan operation depends on a suitably low PVR and adequate PA architecture, it is critical to commence the preparation for a Fontan procedure in the newborn period by appropriately regulating pulmonary blood flow and allowing for adequate growth of the PAs. The ultimate goal of palliative interventions leading up to Fontan operation are to improve clinical symptoms with simultaneously providing optimal PA architecture with low PVR, preserve systolic and diastolic ventricular function, avoid AV valvar regurgitation, and relieve systemic ventricular outflow obstruction. Thus, surgical management of patient with tricuspid atresia is based on the concept that systemic venous blood can be made to pass through healthy mature lungs without the assistance of a ventricular pump.
Physiologically, after the first few months of life, PVR generally falls, and then the goals of subsequent surgical interventions should be to reduce the volume load on the single ventricle. Once systemic arterial-to-PA shunts are eliminated, pulmonary blood flow is reestablished by creating direct connections between the venous system and the PAs (by means of a bidirectional cavopulmonary artery shunt or hemi-Fontan). Any residual anatomic problems such as PA distortion, obstruction to systemic blood flow, AV valvar regurgitation, or a restrictive atrial septal defect should be dealt with at the same procedure to simplify the “definitive” management (Fontan operation). Staging of the Fontan procedure is performed because of the high incidence of pleural effusions and ventricular failure that occurred when patients were taken
directly from a neonatal single-ventricle palliation to the Fontan.
directly from a neonatal single-ventricle palliation to the Fontan.
Table 95.1 Structural Defects That Are Managed by Staged Palliation | ||||||||||
---|---|---|---|---|---|---|---|---|---|---|
|
STAGE II OPERATION
Indications for Stage II Palliation
Infants who have had neonatal palliation become candidates for a second-stage operation by 3 to 6 months of age. Younger age at the time of second-stage surgery is associated with better exercise performance than when surgery is delayed until a later age. Adequate ventricular function remains a prime determinant for a successful Fontan circulation. Indications for second stage include cyanosis secondary to inadequate pulmonary blood flow after initial palliation, congestive heart failure from ventricular volume overload caused by either severe AV valve regurgitation or an elevated Qp:Qs. Prior to cavopulmonary anastomosis, an echocardiogram should be performed. If there is concern regarding the anatomy, patency, or tortuosity of the PAs, then cardiac catheterization is an indication. Cardiac catheterization is also recommended if there is a clinical concern that can be treated interventionally after initial palliation such as aortic arch obstruction, or cyanosis resulting from either decompressing veins or stent stenosis.
The two options for a stage II palliation are the bidirectional Glenn (BDG) and the hemi-Fontan. While they are similar physiologically, each procedure has some unique advantages and different surgical techniques. Typically, our choice at Children’s Hospital of Philadelphia is to perform a BDG on most patients unless a pulmonary arterioplasty is required in which case a hemi-Fontan is the natural choice. Ultimately, the choice of stage II operation will dictate the type of Fontan performed. The BDG is converted to an extracardiac fenestrated Fontan, whereas the hemi-Fontan is easily converted to a lateral tunnel fenestrated Fontan.
Bidirectional Glenn
Glenn performed the first cavopulmonary connection. He used a unidirectional (classic) superior cavopulmonary anastomosis to the right PA. Eventually, this operation was modified to allow continuation of flow to both PAs. This has now become the standard of care for infants with single-ventricle physiology and route to end-stage completion.
The Glenn is performed on normothermic cardiopulmonary bypass, typically with a beating heart. Bicaval cannulation is performed and, once on cardiopulmonary bypass, the shunt is ligated. The azygos vein is ligated and divided. It is important to then maintain proper orientation of the superior vena cava (SVC) to avoid twisting and obstruction of the vessel. The SVC is divided from the right atrium and the cardiac end is oversewn. The distal end of the SVC is anastomosed to the ipsilateral PA in an end-to-side manner (Fig. 95.1). Typically, no prosthetic material is used when performing a Glenn. In cases of pulmonary distortion or narrowing, however, a separate patch of homograft may be necessary to augment the PA. However, if augmentation is necessary, we typically prefer to perform a hemi-Fontan in the cases of PA narrowing.
Hemi-Fontan
The hemi-Fontan procedure (anastomosis of the SVC with the PAs, augmentation of the PAs to eliminate any areas of distortion or narrowing, and elimination of systemic-to-pulmonary shunts or any other sources of pulmonary blood flow) is generally performed at the same time of the Glenn, ideally by 6 months of age. The main differences
between the hemi-Fontan and the Glenn are (1) mandatory use of homograft material for PA augmentation, (2) use of deep hypothermic circulatory arrest, and (3) no division of the SVC to right atrial junction. Alternatively, a “dam” is created using a homograft patch in the orifice of the SVC to obstruct draining directly into the atrium and force blood into the PAs.
between the hemi-Fontan and the Glenn are (1) mandatory use of homograft material for PA augmentation, (2) use of deep hypothermic circulatory arrest, and (3) no division of the SVC to right atrial junction. Alternatively, a “dam” is created using a homograft patch in the orifice of the SVC to obstruct draining directly into the atrium and force blood into the PAs.
The hemi-Fontan procedure is accomplished using hypothermic cardiopulmonary bypass combined with surface cooling before use of deep hypothermic circulatory arrest allowing for adequate visualization of the complex baffle. The PAs are freed from behind the aorta, and a longitudinal incision is made in the anterior aspect of the PAs extending from the level of the origin of the right upper lobe branch to the level of the origin of the left upper-lobe branch (Fig. 95.2A). An incision is made in the most superior portion of the right atrium and is extended onto the medial aspect of the SVC.
At the most rightward extent of the pulmonary arteriotomy, the right PA is anastomosed to the posterior lip of the opened SVC (Fig. 95.2B). A gusset of cryopreserved PA homograft is used to augment the confluence of the PAs and to create a roof over the patulous anastomosis of the PAs to the SVC. A portion of the same homograft gusset is rotated posteriorly and used as a dam to close the junction of the right atrium with the SVC, obligating superior vena caval return to flow exclusively through the branch PAs (Fig. 95.2C). When bilateral superior vena cavae are present, each is anastomosed to the ipsilateral branch PA using cryopreserved homograft to augment the anastomosis in a manner similar to that described above.
At the most rightward extent of the pulmonary arteriotomy, the right PA is anastomosed to the posterior lip of the opened SVC (Fig. 95.2B). A gusset of cryopreserved PA homograft is used to augment the confluence of the PAs and to create a roof over the patulous anastomosis of the PAs to the SVC. A portion of the same homograft gusset is rotated posteriorly and used as a dam to close the junction of the right atrium with the SVC, obligating superior vena caval return to flow exclusively through the branch PAs (Fig. 95.2C). When bilateral superior vena cavae are present, each is anastomosed to the ipsilateral branch PA using cryopreserved homograft to augment the anastomosis in a manner similar to that described above.
Concurrent Procedures
In some cases, additional surgical revision is required at the time of the second-stage operation. One of the most common lesions requiring revision is recoarctation of the aorta. In many centers, this is diagnosed by echocardiography and the diagnosis is confirmed by cardiac catheterization. We favor balloon dilation of the coarctation once detected as long as the patient is approximately 8 weeks from surgical reconstruction. If distal aortic arch recoarctation is neither suitable nor responsive to balloon dilation, patch augmentation at the time of the cavopulmonary anastomosis may be required.
Another indication for more extensive operation at the second stage is AV regurgitation. Often, unloading the volume stressed ventricle and performing the cavopulmonary anastomosis ameliorate the severity of regurgitation. However, in cases of severe regurgitation, inspection and possible intervention upon the AV valve is recommended. Commissuroplasty, annuloplasty, choral shortening, and leaflet extension are common valvuloplasty techniques to minimize regurgitation.
Postoperative Management
Conversion from an aortopulmonary shunt to a stage II operation decreases the work of the single ventricle by decreasing the volume load on the ventricle. Postoperative management includes judicious administration of fluids guided by cardiac filling pressures and early extubation, which usually can be accomplished in the operating room or within 3 to 5 hours after completion of surgery. Positive pressure ventilations can cause increased airway pressures and adversely affect both PVR and ventricular filling. Spontaneous breathing likely leads to an increase in PCO2 that promotes cerebral blood flow therefore increasing SVC return to the lungs or pulmonary blood flow.
Typically, hemodynamics are robust. In fact, transient hypertension has been observed after the cavopulmonary shunt. One of the goals of postoperative management is to minimize the transpulmonary gradient to allow passive blood flow through the lungs guaranteeing return of oxygenated blood to the common atrium. An elevated transpulmonary gradient may cause cyanosis and could result from elevated PVR, downstream pulmonary venous obstruction, or from impaired lung expansion due to hemo- or pneumothorax. Elevation of PVR due to cardiopulmonary bypass may be decreased by the administration of nitric oxide as a pulmonary vasodilator.
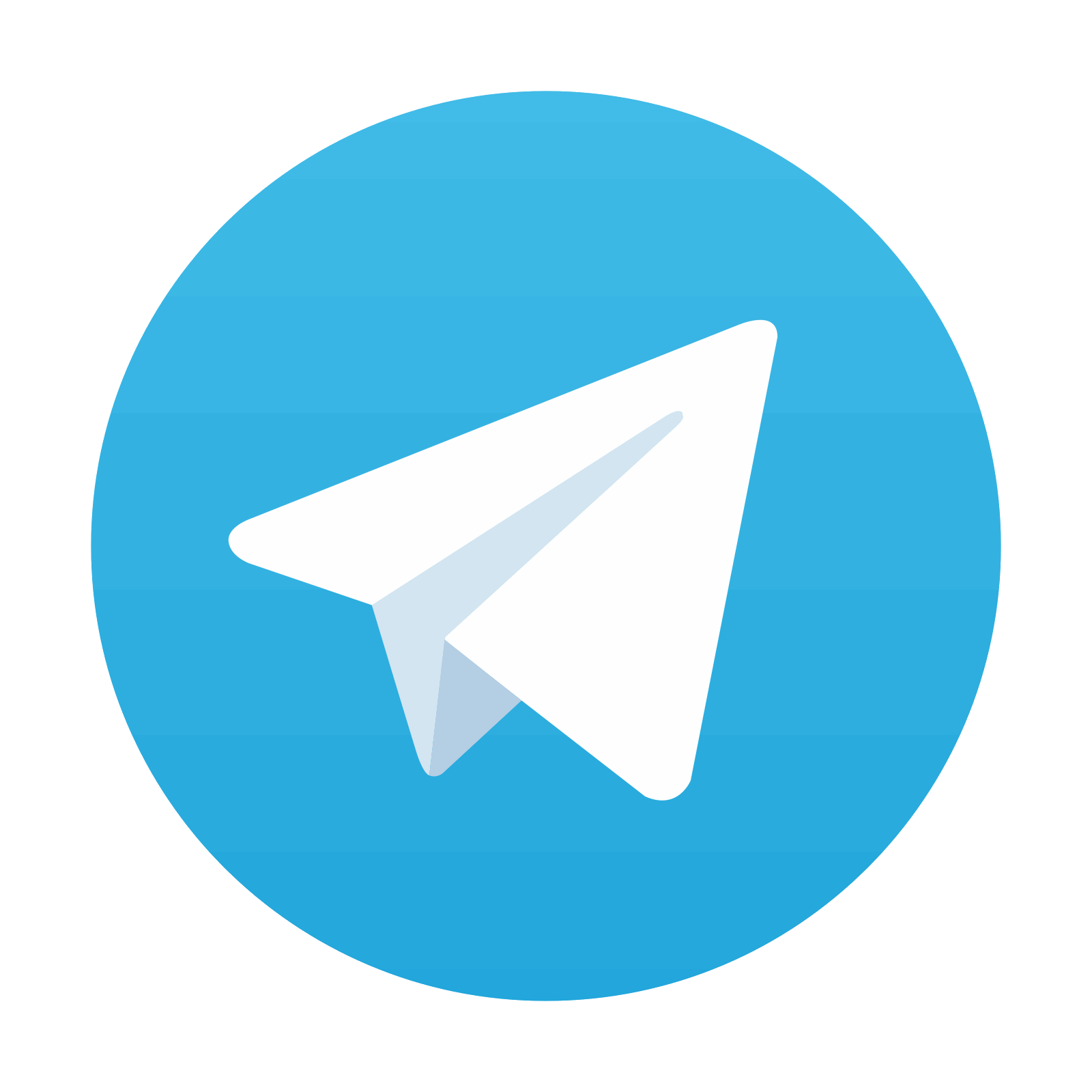
Stay updated, free articles. Join our Telegram channel

Full access? Get Clinical Tree
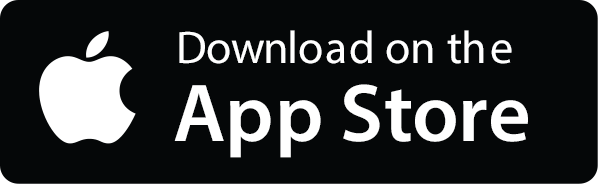
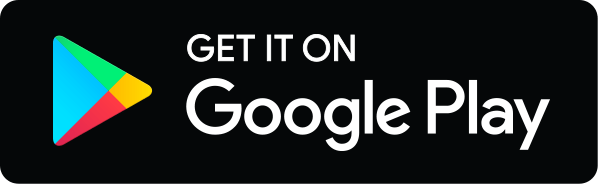