Transposition of the Great Arteries
Aaron W. Eckhauser
Thomas L. Spray
Complete transposition of the great arteries (TGA) is a congenital cardiac defect in which there is anatomic reversal of the relationship of the great arteries. The aorta arises entirely or largely from the right ventricle, and the pulmonary artery arises entirely or largely from the left ventricle (ventriculoarterial discordant connection). The lesion is incompatible with life without surgical intervention because in the resulting physiologic abnormality the pulmonary and systemic circulations exist in parallel instead of in series. Survival, therefore, depends on mixing between the pulmonary and systemic circulations. In spite of the severity of this cardiac defect, surgical therapy has become standardized such that anatomic and physiologic repair can be accomplished in the first few days of life in the majority of affected children.
TGA may coexist with other cardiac lesions, including coarctation of the aorta and patent ductus arteriosus. Transposition is associated with intact ventricular septum in approximately 50% of patients, ventricular septal defect (VSD) in 25%, and VSD with functional or anatomic left ventricular outflow tract obstruction (pulmonary stenosis) in 25%. Transposition with interruption of the aortic arch is a rare association.

Complete TGA is characterized by atrioventricular concordance and ventriculoarterial discordance ({S,D,D} according to the Van Praagh classification system). There have been many interpretations and applications of the term transposition, but in this chapter, complete TGA is defined as atrial situs solitus, atrioventricular concordance, and ventriculoarterial discordance {S,D,D}.
The morphogenesis of the abnormal relationship between the great arteries and the ventricles in TGA is controversial. In normal cardiac development, the subaortic conus is static, and dominant growth of the pulmonary conus forces the pulmonary valve anterior, superior, and to the left. Van Praagh suggested that in TGA the subaortic conus persists during normal looping of the ventricles, whereas the subpulmonary conus undergoes absorption. Differential growth of the subaortic conus in transposition, therefore, pushes the aorta anteriorly and disrupts the aortic-mitral valve continuity. If the subpulmonary conus fails to develop, the pulmonary artery will maintain a posterior location and pulmonary-mitral valve continuity will occur. Consequently, the aortic valve becomes anterior to the pulmonary valve, permitting both semilunar valves to connect with the distal great vessels without the rotation that is hypothesized to occur in normal cardiac development. Because conal development determines the rotation of the truncus arteriosus, the great arteries are similar in relationship at the semilunar valves as they are at the arch. Anatomic variations are often encountered, although the heart is left-sided with atrial situs solitus in 95% of patients. Left-to-right juxtaposition of the atrial appendages is a sign of other intracardiac anomalies. A true ostium secundum atrial septal defect is present in 10% to 20% of cases, but the majority of atrial communications are via a patent foramen ovale. Right aortic arch is present in 4% of patients with intact ventricular septum and up to 16% of those with VSD. Up to 50% of patients with TGA will have an associated VSD, many of which will spontaneously close. The VSDs are commonly perimembranous (conoventricular) in location, although they may be found anywhere in the ventricular septum. Pulmonary stenosis or atresia, overriding or straddling atrioventricular valves, coarctation of the aorta, and interruption of the aortic arch have all been noted in association with transposition and VSD.
The spatial relationship of the great vessels is quite variable, but the aorta is most frequently to the right and anterior to the pulmonary artery {S,D,D}. Less commonly, the aorta can be anterior and to the left with mirror-image branching {I,L,L} or anterior and to the left with normal arch branching {S,D,L}. The sinuses of Valsalva and coronary artery ostia typically face the corresponding pulmonary arterial sinuses of Valsalva, permitting transfer of the coronary arteries in the arterial switch operation. Only a small number of patients who have a coronary artery that originates from a nonfacing coronary sinus pose a problem for arterial switch reconstruction.
The coronary anatomy can be described using a modified Leiden convention. In this description, the surgeon places themselves in the nonadjacent aortic sinus looking toward the pulmonary valve. The sinus to the surgeon’s right hand is labeled “1” and the sinus to the surgeon’s left hand is labeled “2” (Fig. 89.1). Coronary branching patterns can be described as normal, looping, or intramural. In the “normal” pattern, which accounts for 60% to 70% of cases, sinus 1 gives rise to the anterior descending and circumflex arteries. Sinus 2 gives rise to the right coronary artery. In this pattern, none of the major arterial branches crosses in front of or behind either of the major arterial trunks. The “looping” pattern, found in one-third of cases, results when one or more of the three major coronary arteries run in front of or behind the major arterial trunks. Posterior looping results when a coronary artery runs posterior to the pulmonary artery, most commonly the circumflex arising from sinus 2. Anterior looping results when one of the branches runs anterior to the aorta. This can occur when the left anterior descending artery arises from the right coronary artery in sinus 2 or a single coronary artery arises from either sinus 1 (right coronary artery crosses) or 2 (left main equivalent crosses). Branching patterns giving rise to double loops are not uncommon and account for 15% to 20% of cases. Finally, the “intramural” course occurs when a coronary artery appears to arise from one sinus, crosses through the media of the aortic wall behind the valve commissure, and
truly arises from the other adjacent aortic sinus. Occasionally, there is no true circumflex coronary artery, but separate branches arise from the left coronary to supply the corresponding portion of the left ventricle. Abnormal coronary branching patterns are more commonly seen in transposition with VSD than when an intact ventricular septum is present.
truly arises from the other adjacent aortic sinus. Occasionally, there is no true circumflex coronary artery, but separate branches arise from the left coronary to supply the corresponding portion of the left ventricle. Abnormal coronary branching patterns are more commonly seen in transposition with VSD than when an intact ventricular septum is present.
Important left ventricular outflow tract obstruction is unusual in association with TGA but has significant implications for management strategies. The most common type of left ventricular outflow tract obstruction is dynamic, resulting from leftward displacement of the hypertrophied muscular ventricular septum secondary to the development of higher right systemic ventricular pressure. The septum may then narrow the outflow tract, resulting in abnormal systolic anterior mitral leaflet motion and a situation similar to that noted in hypertrophic obstructive cardiomyopathy. Posterior malalignment of the ventricular septum may create a tunnellike obstruction, and fibrous tags arising from the mitral apparatus or membranous septum can result in significant subvalvar obstruction, more commonly when a VSD is present. True valvar stenosis is uncommon, but a nonobstructive bicuspid pulmonary valve is not infrequently present. In rare cases, aortic arch obstruction with coarctation or true interruption of the aortic arch has been observed in patients with TGA, left ventricular outflow tract obstruction, and VSD.
PATHOPHYSIOLOGY
TGA {S,D,D} is a relatively common form of congenital heart disease, accounting for 9.9% of infants with congenital heart disease in a New England study and representing a frequency of 0.206 per 1000 live births. A distinct male predominance is noted, with a male-to-female ratio of 2:1, which increases to 3.3:1 when the ventricular septum is intact. In complex forms of transposition, a sexual predominance has not been noted. Untreated, 90% of children with D-TGA and an intact ventricular septum will die by 1 year of age.
The parallel relationship of the pulmonary and systemic circulations in TGA results in nonoxygenated venous blood passing through the right ventricle to the aorta, whereas the oxygenated pulmonary venous blood passes through the left ventricle back to the pulmonary artery. Mixing between the pulmonary and systemic circulations at the atrial, ventricular, or great vessel level through a patent foramen ovale or atrial septal defect, a VSD, or a patent ductus arteriosus is mandatory for survival. Patients with TGA and an intact ventricular septum survive initially because of aortopulmonary flow through a patent ductus arteriosus. After birth, both ventricles are relatively noncompliant, and infants with transposition often have increased pulmonary blood flow. This results in left atrial enlargement and functional incompetence of the foramen ovale with atrial level mixing of oxygenated and nonoxygenated blood. Inadequacy of mixing, however, will result in marginal tissue oxygenation that does not improve with oxygen administration. Atrial balloon septostomy results in improved admixture and improved tissue oxygen delivery.
Patients with TGA and significant VSD often have higher oxygen saturations by virtue of greater pulmonary blood flow and greater mixing at both the atrial and ventricular levels. In children with high pulmonary blood flow, pulmonary resistance may progressively increase during infancy. The early development of severe pulmonary vascular disease in children with TGA is exacerbated in patients with associated VSD. The rapid development of pulmonary obstructive disease may be related to hypoxemia, increased sympathetic activity, and excessive pulmonary blood flow.
Although neonatal pulmonary vascular resistance is elevated in infants with TGA, the resistance falls progressively during the neonatal period, coinciding with changes in the pulmonary and systemic ventricular compliance. In a normal infant, there is an increase in the left ventricular volume and pressure load and a decrease in the right ventricular volume and pressure load shortly after birth, resulting in a rapid increase in the left ventricular myocardial mass. Normal development of the left ventricle is lost in infants with D-TGA because the left ventricle ejects to the low-resistance pulmonary vascular bed. Thus, the left ventricle does not increase muscle mass relative to the right ventricle and within a few weeks loses the ability to maintain adequate cardiac output against significant afterload. This change occurs despite the fact that the left ventricle maintains a volume load in patients with transposition and intact ventricular septum. However, when a VSD or large patent ductus arteriosus is present, both volume and pressure overload of the left ventricle are maintained. In D-TGA with left ventricular outflow tract obstruction without a VSD, a ventricular pressure load is imposed without a significant volume load. These physiologic changes in the neonatal heart are important for the consideration of surgical approaches because after a few weeks of postuterine life, the left ventricle in D-TGA with intact ventricular septum takes on the characteristics and wall thickness of a pulmonary ventricle and may not be adequate to support the systemic circulation.
CLINICAL FEATURES
The most common clinical finding in an infant with TGA is cyanosis (arterial partial pressure of oxygen 25 to 40 mmHg), which varies in degree depending on associated anomalies. Typically, the cyanosis is more pronounced when the ventricular septum is intact and is often present at birth. The development of cyanosis later in infancy is usually associated with the presence of a significant VSD or left ventricular outflow tract obstruction. Congestive heart failure (CHF) may be the predominant clinical finding in patients with a large VSD or patent ductus arteriosus. Symptoms of cardiac failure, however, are rarely present in the first week of life but commonly appear by 1 month of age as pulmonary vascular resistance decreases and pulmonary blood flow becomes excessive, even in a patient with an intact ventricular septum.
MANAGEMENT
The widespread use of fetal ultrasound techniques has resulted in the common antenatal diagnosis of TGA. In fetuses with TGA and intact ventricular septum, the four-chamber view is normal. The parallel course of the aorta and pulmonary artery differentiates the fetus with TGA from those in which the arterial trunks are normally crossing. Color and pulsed wave Doppler showing inflow and outflow patterns of the left ventricular outflow tract coupled with a typical ejection pattern from the pulmonary artery further confirm the diagnosis of transposition. Postnatally, echocardiography has now generally supplanted cardiac catheterization in the majority of patients with simple complete transposition. Cardiac catheterization is now indicated only in infants in whom inadequate shunting is noted or if associated intracardiac or extracardiac abnormalities require clarification. Echocardiographic views confirming a posterior great vessel that divides into right and left pulmonary arteries and arises from the left ventricle in association with an anterior aorta arising from a right ventricle confirm the diagnosis of TGA. The intracavitary shunts can be determined by Doppler echocardiography, and several echo views can determine the size and location of VSDs with reference to the infundibular septum, the nature and size of atrial communications, the anatomy of the atrioventricular valves, and the presence and location of significant degrees of subpulmonary stenosis. In the majority of cases, the origins and anatomic distributions of the coronary arteries can be adequately visualized by echocardiography without the need for catheterization. Since virtually all coronary patterns can be dealt with surgically, preoperative identification of coronary anatomy is not necessary.
Cardiac catheterization is reserved for patients with significant clinical instability in order to improve the degree of intracavitary shunting by enlarging the interatrial septal communication. At catheterization, the left atrial pressure is usually greater than the right atrial pressure, and the pressure in the pulmonary (left) ventricle depends on the presence or absence of a VSD, the valvar or subvalvar stenosis, the age of the patient, and the magnitude of elevation of pulmonary vascular resistance.
The most important aspect of preoperative management of TGA is that oxygenated left atrial blood gets to the head. A PDA only helps to fill the left atrium, so atrial or ventricular level mixing is critical. If inadequate interatrial shunting results in clinical instability (acidemia, severe hypoxemia), the mainstay of management has been a Rashkind balloon atrial septostomy. A balloon-tipped catheter is passed through the systemic veins, through the right atrium and foramen ovale, and into the left atrium. The balloon is inflated and pulled vigorously across the atrial septum to tear the foramen ovale, improving admixture of pulmonary and systemic venous blood. This procedure may be performed in the intensive care unit with echocardiographic guidance in children who are clinically unstable. In patients with TGA and intact ventricular septum, atrial level mixing results in moderate hypoxemia but rarely CHF. Since an atrial septostomy decompresses the left ventricle, delayed repair may result in poor left ventricular performance. Prostaglandin E1 is often administered to maintain ductal patency and increase pulmonary blood flow helping to stabilize patients before early operative repair. In patients with atrial and ductal level shunting, mixing is improved with only mild hypoxemia but can be complicated by CHF and low diastolic pressures. Patients with PDA and restrictive atrial level shunting often develop pulmonary edema and metabolic acidosis. In patients with TGA and VSD, atrial, ventricular, and ductal shunting results in minimal hypoxemia but severe CHF once the pulmonary resistance falls. Therefore, patients who are good anatomic candidates for an arterial switch procedure who have acceptable arterial oxygen saturations are generally referred for early arterial switch without intervening atrial septostomy.
HISTORY OF SURGICAL REPAIR
Initial surgical therapy for TGA involved creation of an atrial septal defect using a closed technique to increase the mixing between the systemic venous and pulmonary venous circulations. This was first performed by Blalock and Hanlon in 1950. Although early mortality was high with this operative approach, successful creation of an atrial septal defect resulted in significant palliation in many of these children. Initial attempts to reverse the transposed vessels by both Mustard and Bailey were frustrated by an inability to maintain coronary perfusion and poor function of the anatomic left ventricle. Thus, initial surgical therapy was directed toward atrial transposition of the pulmonary and systemic venous returns. In 1952, Lillehei and Varco transferred the right-sided pulmonary veins to the right atrium and connected the inferior vena cava to the left atrium. A successful modification of this technique using an allograft to connect the inferior vena cava to the left atrium was described by Baffes in 1956.
Multiple attempts were made at both atrial and arterial repairs of TGA during the 1950s, and in 1954 Albert suggested the concept of switching the atrial septum so that caval return was directed to the left ventricle and the pulmonary venous return to the right. The atrial switch concept was first successfully accomplished by Senning in 1959 using an ingenious technique for relocating the walls of the right atrium and the atrial septum. Many of the early attempts at atrial repair were frustrated by the fact that the operation was performed on patients between 1 and 2 years of age and significant pulmonary vascular obstructive disease had already developed in many of these children. In 1964, Mustard described an alternate procedure for intra-atrial repair: excising the atrial septum and creating a large interatrial baffle of pericardium to redirect pulmonary and systemic venous blood resulting in a larger atrium than the Senning operation. Early results with the Mustard operation were markedly improved over the previously reported Senning repairs and reflected the significant population of patients in Toronto who had undergone successful Blalock-Hanlon atrial septectomies early in life.
A major development in the surgical treatment of TGA occurred in 1966 when Rashkind and Miller reported the use of a
balloon catheter technique to enlarge the atrial septal defect in patients with TGA, resulting in improved early physiologic stability and decreasing the need for operative atrial septectomy. During the 1960s, the Mustard operation became the most commonly performed procedure for transposition. As surgical techniques improved, it became clear that repair in the first few months of life could be accomplished with a low operative mortality and improved results compared with repair at a later age. In 1970, the Senning procedure reemerged as persistent problems with baffle obstruction and arrhythmias after the Mustard operation became well defined and the ability to use autologous tissue for the atrial reconstruction became a preferred approach.
balloon catheter technique to enlarge the atrial septal defect in patients with TGA, resulting in improved early physiologic stability and decreasing the need for operative atrial septectomy. During the 1960s, the Mustard operation became the most commonly performed procedure for transposition. As surgical techniques improved, it became clear that repair in the first few months of life could be accomplished with a low operative mortality and improved results compared with repair at a later age. In 1970, the Senning procedure reemerged as persistent problems with baffle obstruction and arrhythmias after the Mustard operation became well defined and the ability to use autologous tissue for the atrial reconstruction became a preferred approach.
The success with the atrial switch operations for TGA with intact septum did not translate to repair of transposition with a large VSD. Disappointing results with VSD closure and atrial repair in this group of patients continued to be a stimulus for the development of an arterial switch procedure, which was first successfully performed by Jatene and colleagues in 1975. Yacoub shortly thereafter reported additional successful cases. The success of the arterial switch procedure with reimplantation of the coronary arteries in some patients with transposition and VSD led to reintroduction of this technique for patients with intact ventricular septum. Yacoub’s initial attempts in patients with TGA and an intact ventricular septum were unsuccessful in 1972. However, additional reports by 1976 suggested that such repair was possible in infancy. Early mortality with the arterial switch in infancy was related to the fact that the pulmonary left ventricle was not prepared to sustain systemic pressure. Therefore, initial approaches included pulmonary arterial banding (with or without a systemicto-pulmonary shunt) as a first stage followed by a later arterial switch. However, Yacoub, Quaegebeur, Brawn, and Castaneda subsequently demonstrated the ability to repair simple transposition in the first few days of life by an arterial switch operation while the pulmonary ventricle has relatively high pressure. The rapidly decreasing mortality for arterial switch operations has resulted in this surgical approach becoming the standard corrective surgical procedure, which results in both an anatomic and physiologic reconstruction of this cardiac defect.
Operative approaches to TGA, VSD, and significant left ventricular outflow tract obstruction were less successful in the early period of cardiac surgery. In 1969, Rastelli suggested the combination of an intraventricular tunnel repair: closing the VSD and baffling the pulmonary venous blood from the left ventricle to the aorta, closing the pulmonary artery exit from the left ventricle, and creating an extracardiac conduit from the anatomic right ventricle to the pulmonary bifurcation, resulting in an anatomic repair for TGA/VSD and left ventricular outflow tract obstruction. Obstruction of the reconstructed left ventricular outflow tract remains a common complication of the Rastelli-type repair and has led to recommendation of a variation of intraventricular repair by Lecompte (the “réparation à l’étage ventriculaire” [REV] procedure) and the aortic root translocation (Nikaidoh procedure).
OPERATIVE INTERVENTION: PALLIATIVE OPERATIONS
The advent of the balloon atrial septostomy by Rashkind and Miller has essentially eliminated the need for the Blalock-Hanlon atrial septectomy. Infants with associated cardiac abnormalities and a thick atrial septum who are considered for later atrial baffle repair may benefit from the Blalock-Hanlon technique, although the safety of cardiopulmonary bypass has resulted in the common use of open atrial septectomy in such patients. Pulmonary arterial banding has been used for palliation with TGA and VSD in young infants who have intractable CHF until operative repair at 3 to 6 months of age. As the results with the arterial switch procedure and VSD closure in infancy have improved, banding in most instances is unnecessary because complete repair can be performed safely. Therefore, banding of the pulmonary artery has now been limited to very small neonates who might benefit from a delay in corrective surgery and those patients with transposition and an intact ventricular septum who present late for arterial switch repair and require “training” of the left ventricle to work at a higher pressure in order to become the systemic ventricle. For similar reasons, pulmonary arterial banding is used in patients with TGA who develop right ventricular dysfunction and failure after atrial baffle operations as a component of staged conversion to an arterial switch repair. Pulmonary arterial banding in TGA is a delicate procedure because limitation of pulmonary blood flow results in significant hypoxia and metabolic acidosis, and loose banding results in inadequate protection of the pulmonary vascular bed and poor development of the left (pulmonary) ventricle. Thus, in most situations in which preparation of the left ventricle is undertaken for conversion to an arterial switch procedure, banding must be associated with creation of an aortopulmonary shunt to maintain adequate pulmonary blood flow to prevent hypoxemia and ventricular dysfunction.
SURGICAL CORRECTION
Successful correction of TGA results in the rerouting of systemic venous blood to the pulmonary circulation and the pulmonary venous blood into the systemic arterial circulation. This may be accomplished at the atrial, ventricular, or great arterial level. The earliest repairs of TGA involve rerouting of the systemic and pulmonary venous returns at the atrial level, resulting in an adequate physiologic repair but not an anatomic repair because the morphologic right ventricle continues to be the systemic ventricle. Ventricular (Rastelli) and great arterial (arterial switch) repairs are more anatomic corrections resulting in the morphologic left ventricle as the systemic ventricle.
Atrial Repair
Despite the excellent results with atrial switch operations for TGA, anatomic correction has resulted in the arterial switch procedure becoming the standard surgical repair for TGA. Therefore, the Senning and Mustard operations will not be described in detail in this chapter.
Arterial Repair
The technique of the arterial switch operation involves transection of the great arteries, transfer of the coronary arteries, and repositioning of the great vessels. The procedure is performed through a median sternotomy with the use of cardiopulmonary bypass with mild or no hypothermia. In rare cases, the entire operation can be performed with a single period of circulatory arrest, or circulatory arrest can be used for a brief period for atrial septal defect exposure and coronary transfer. After sternotomy, a portion of the anterior pericardium can be excised for use as an autologous patch for reconstruction of the anterior great vessel. The patch can be used either fresh or fixed in a glutaraldehyde solution to make for easier handling. Currently, we use pulmonary homograft patch material for pulmonary artery reconstruction. The ligamentum arteriosum or patent ductus arteriosus is dissected out, and the branches of the right and left pulmonary arteries are mobilized well out into the hilum of the lungs bilaterally. It is important to freely mobilize the
pulmonary arterial branches out beyond the bifurcation to the lobar branches to permit safe anterior relocation of the pulmonary artery during the Lecompte maneuver without causing compression of the pulmonary arteries by the aorta. As noted in Figure 89.2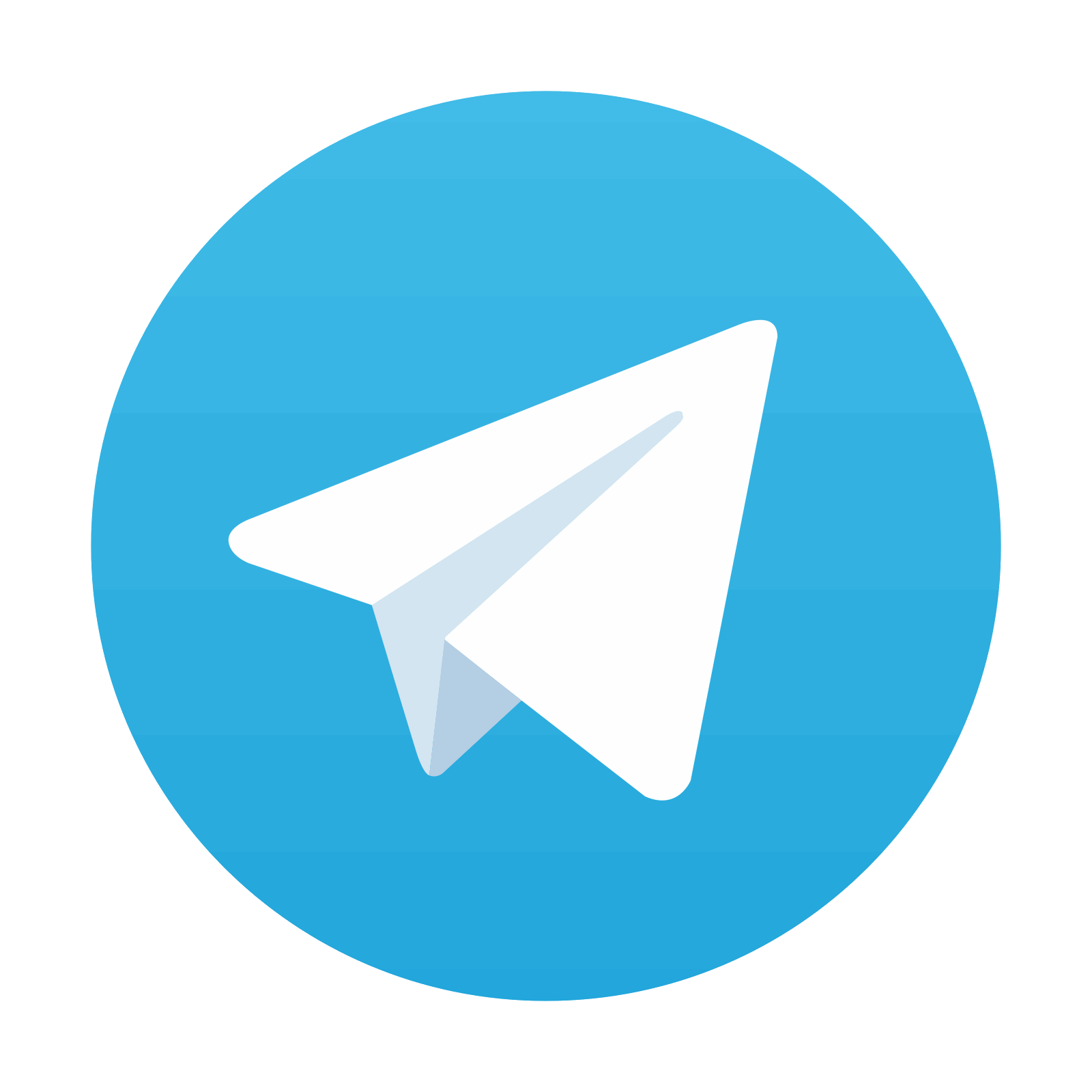
pulmonary arterial branches out beyond the bifurcation to the lobar branches to permit safe anterior relocation of the pulmonary artery during the Lecompte maneuver without causing compression of the pulmonary arteries by the aorta. As noted in Figure 89.2
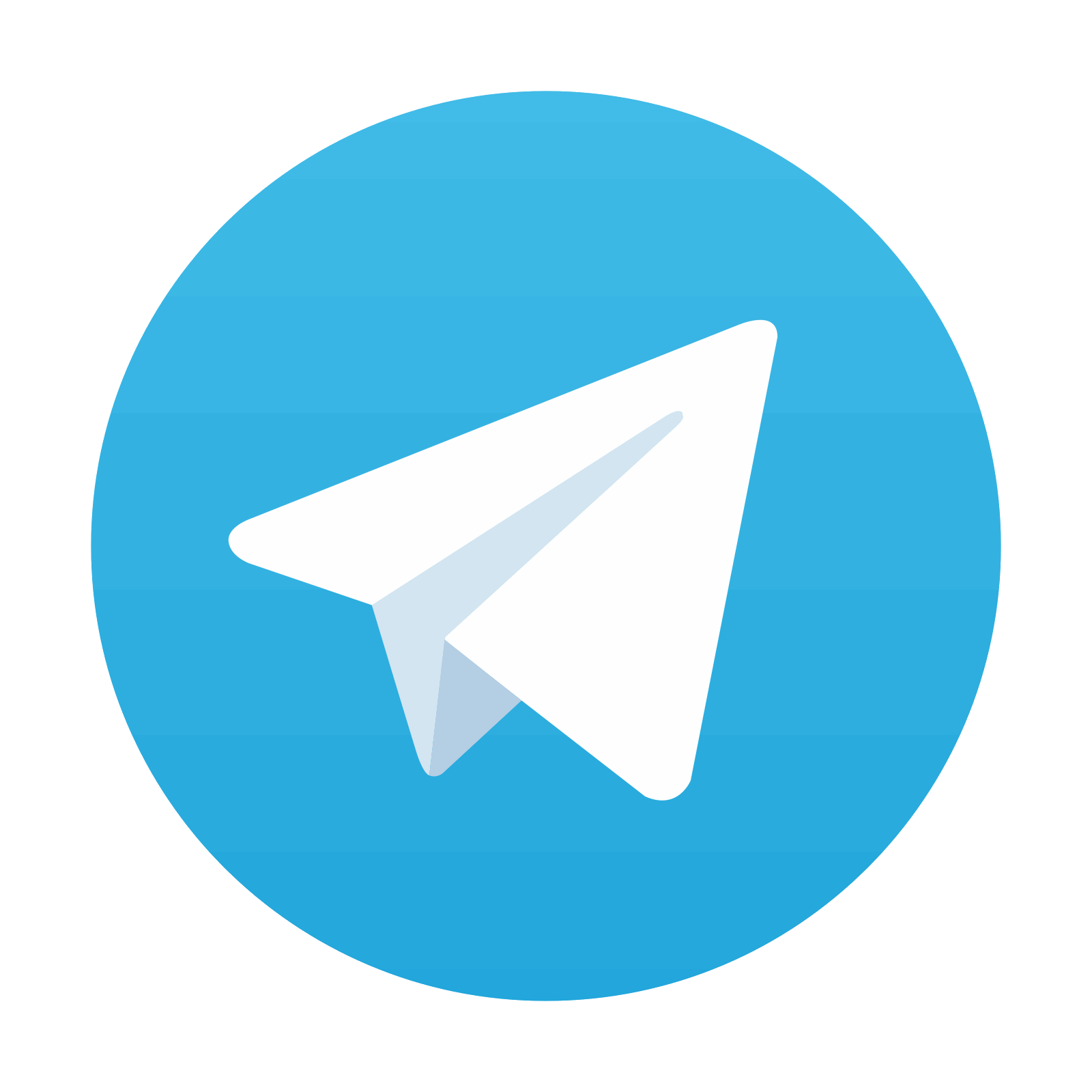
Stay updated, free articles. Join our Telegram channel

Full access? Get Clinical Tree
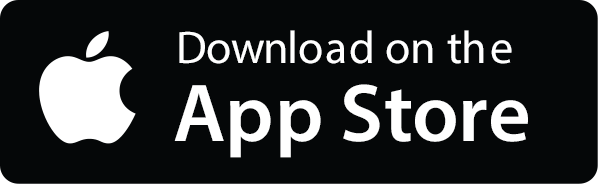
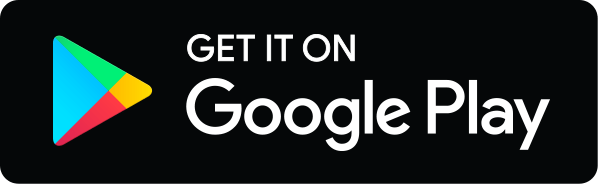
