Time-of-Flight MR Angiography
The three main techniques for clinical MR angiography are time-of-flight (TOF), phase–contrast, and gadolinium-enhanced imaging. TOF techniques rely on flow-related enhancement and do not use exogenous contrast material. Methods that are used include 2D and 3D gradient echo techniques and a combined approach called multiple overlapping thin-slab acquisition (MOTSA). 2D TOF is the most commonly used technique for most cardiovascular imaging. Applications include MR imaging of the carotid arteries; some peripheral vascular MR angiography, particularly in the calves and feet; and MR venography. At the conclusion of this chapter, sample TOF MR protocols are provided for carotid artery imaging and MR venography. Gadolinium-enhanced carotid and peripheral MR angiography protocols are contained in Chapter II-4.
KEY CONCEPTS
[right half black circle] With TOF imaging, strategies to reduce background signal must be balanced against saturation of flowing protons.
[right half black circle] Saturation bands can be combined with TOF to create selective MR arteriography or venography.
[right half black circle] Flow compensation, together with reduced TE and small voxel size (high spatial resolution), reduce overestimation of stenoses.
[right half black circle] 3D TOF has higher spatial resolution and is less susceptible to turbulent flow than 2D TOF, but it is also less sensitive to slow flow.
[right half black circle] Clinical Protocols:
2D TIME-OF-FLIGHT MR ANGIOGRAPHY
The goals of time-of-flight imaging are to produce high-spatial-resolution images where flow-related enhancement is maximized, flow-related dephasing is minimized, and background tissue is suppressed. Background tissue suppression is achieved because TOF imaging is a gradient echo technique, and so exposure to repeated excitation pulses in rapid succession saturates most tissue in an imaging slice (Chapter I-4). Flowing blood enters the slice fully magnetized (not having experience any excitation pulses), so it can generate high signal following the RF pulse. This creates bright signal in vessels with flowing blood relative to a dark background of stationary tissues. To maximize flow-related enhancement, the imaging slices are positioned perpendicular to the direction of blood flow. Flow compensation gradients (see Chapter II-1), applied in one or more directions, reduce flow-related dephasing. A typical pulse sequence diagram for 2D TOF imaging is shown in Figure II2-1.
With TOF, any inflow of unsaturated protons will produce signal. Therefore, both arteries and veins are bright. A spatially selective saturation band is usually applied to saturate venous signal when MR arteriography is desired.
Sequence parameters that can be manipulated to optimize TOF imaging are described in the following paragraphs.
Parameter Selection
TR
One challenge in TOF angiography is the need to balance flow-related enhancement and background tissue suppression. Selection of TR is the key to the nature of this balance.
Recall from Chapter II-1 that a long TR and thin slices favor flow-related enhancement. With thinner slices, shorter TRs can be used. The gain in time with shorter TR compensates for the need to image more slices for the same anatomic coverage. However, thinner slices result in higher spatial resolution, and shorter TR achieves greater background suppression, and so this strategy can be advantageous.
In the typical setting, TRs vary in the range of 25 to 50 msec. Longer TRs make the sequence more sensitive to slow flow, at the cost of longer acquisition time.
IMPORTANT CONCEPT:
Long TR favors flow-related enhancement, decreases background suppression, and increases acquisition times.
![]() FIGURE II2-1. Pulse sequence diagram for 2D TOF imaging. Flow compensation gradients are shown in both the slice-select and frequency-encoding directions. |
![]() FIGURE II2-2. Effect of flip angle on TOF imaging. Higher flip angles cause greater saturation of background tissues. Note that slowly moving blood has signal intermediate between those of fast-moving blood and stationary tissues. |
Flip Angle
Selection of the flip angle is important for TOF angiography. Recall from Chapter I-4 (Figure I4-6) that when the TR is less than the T1 relaxation time, the flip angle significantly affects the magnetization available to generate signal. Specifically, the higher the flip angle, the greater the suppression of background tissue (Figure II2-2).
Higher flip angles produce more signal and consequently greater contrast between flowing and stationary tissues, provided that the flow into the slice is sufficiently fast for all spins in the vessels to be completely replaced with each excitation. However, with slow-flowing protons, higher flip angles will cause partial saturation. Typical flip angles vary from 45° to 60°, but flip angles can be as low as 30° or as high as 90°.
IMPORTANT CONCEPT:
Higher flip angles are advantageous with fast-flowing vessels because they lead to more signal from flowing blood and less signal from the background stationary tissue.
Voxel Size
Decreases in voxel size reduce intravoxel dephasing and improve spatial resolution. Smaller voxels are achieved by increasing the number of phase-encoding steps (NPE) or reducing the field of view. Higher NPE, together with thinner slices, usually come at the expense of increased acquisition times and lower SNR.
TE and Flow Compensation
To minimize flow-related dephasing, TE can be shortened or flow compensation used. Flow compensation gradients in read and slice-select directions (as shown in Figure II2-1) minimize effects of velocity on phase dispersion. Higher-order flow effects are minimized by keeping TE as short as possible (allowing for the flow compensation gradients). TE values are typically on the order of 8 to 9 msec.
Acquisition Times
For a typical TR of 25 msec and a 256 × 128 imaging matrix, the acquisition time per slice is 3.2 sec. To image a slab of 64 2D slices would require approximately 3 minutes of imaging time. With 2D TOF, contiguous slices are typically imaged with a small degree of overlap. This facilitates image reconstruction (as described subsequently) by avoiding imaging artifacts associated with discontinuities at the slice edges. For example, if the slice thickness is 3 mm and a 1 mm overlap is used, then the total slab thickness for 64 slices is 64 × (3 mm − 1 mm) = 128 mm.
Other Considerations
In vessels with pulsatile blood flow, imaging during systole can improve quality of TOF images. As will be discussed in Chapter III-1, synchronization can be achieved using central electrocardiographic gating or peripheral gating. The acquisition times increase considerably with gating, because imaging is performed only during a short portion of each cardiac cycle.
TOF images have a component of T1 weighting, so tissues other than vessels, such as subcutaneous fat and bone marrow, will increase the signal. One solution is to suppress signal from fat, using either a frequency-selective fat saturation pulse, selective water excitation, or a 180° inversion pulse to null fat (Chapter I-9). Because each of these options lengthens acquisition times, it is common to rely on post-processing methods to reduce the effects of unwanted fat signal (as described subsequently). Another option for suppressing background tissue, particularly for intracranial angiography, uses magnetization transfer pulses (Chapter I-3).
Arteriography vs Venography
If a sequence is sensitive to slow flow
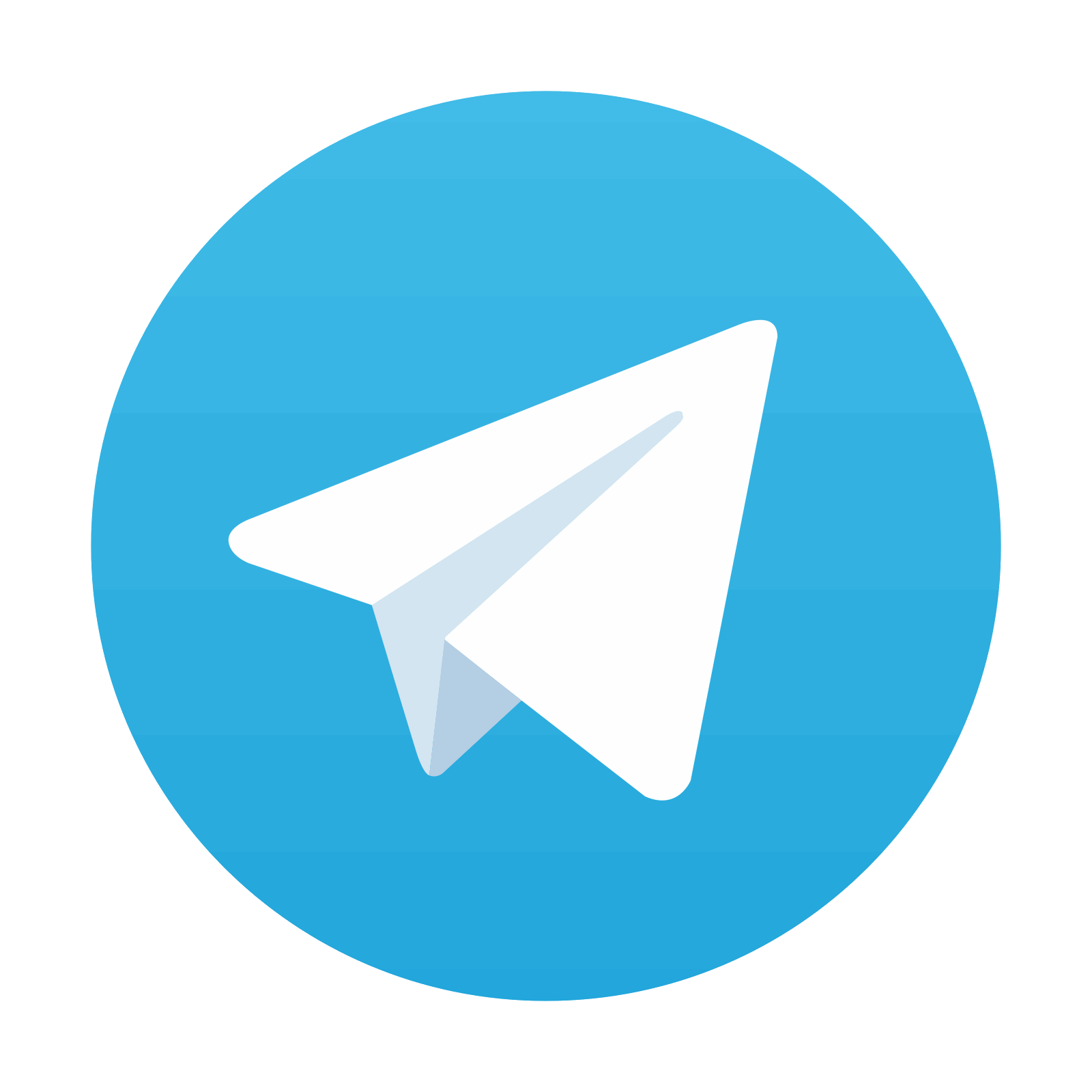
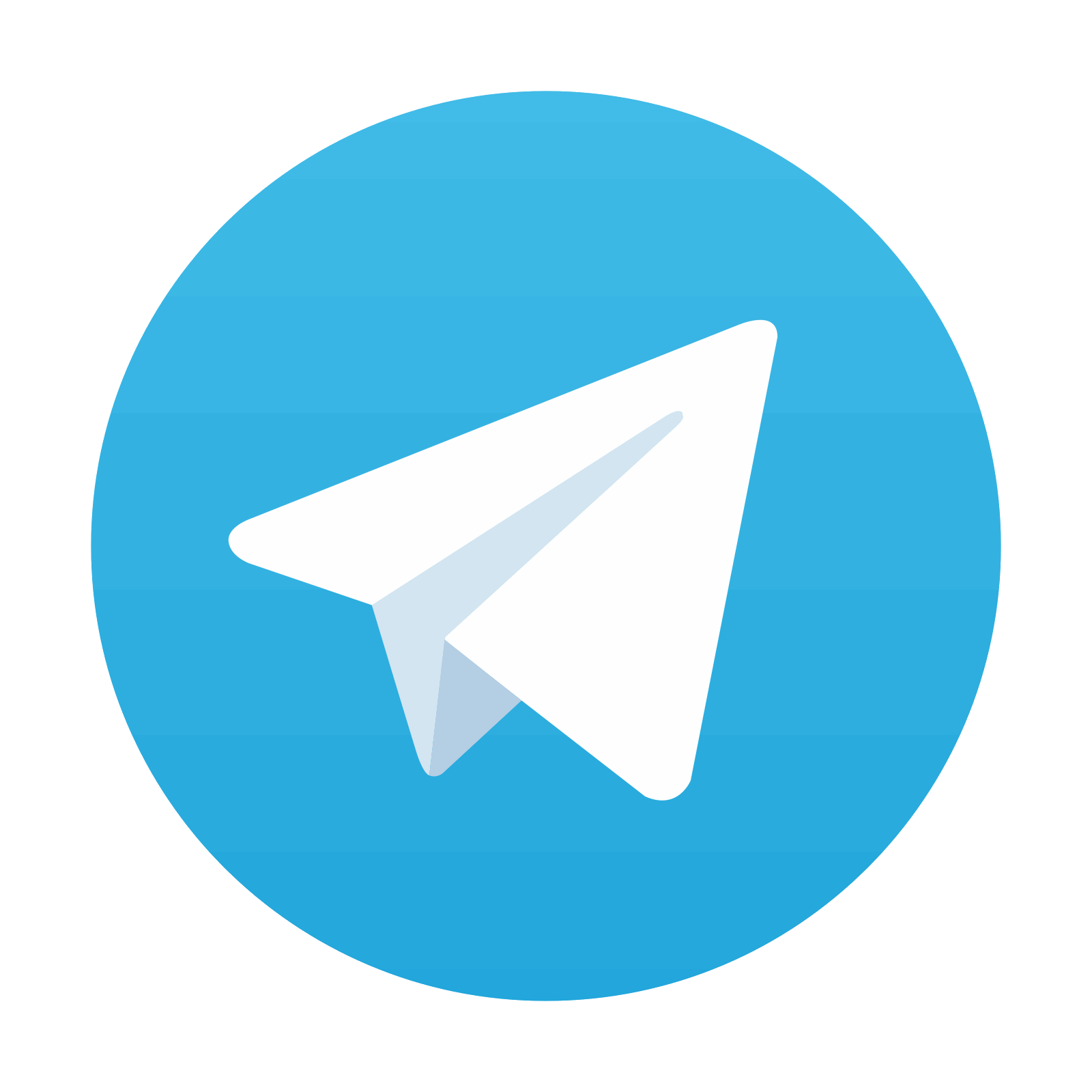
Stay updated, free articles. Join our Telegram channel

Full access? Get Clinical Tree
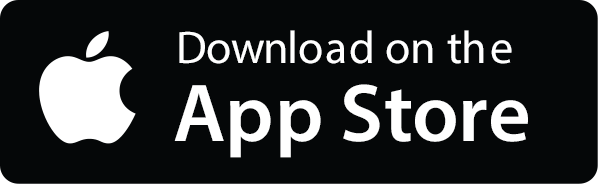
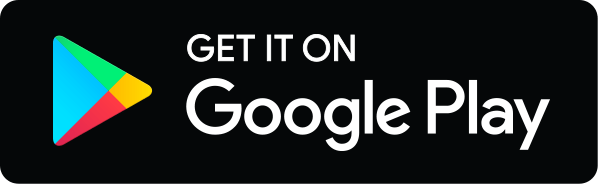
