14
Thoracic and thoraco-abdominal aortic disease
Introduction
Endovascular repair (EVR) of the thoracic aorta has become an established treatment modality despite a relatively poor evidence base. There are good explanations for the lack of randomised data when the clinical outcomes for pathologies with established indications for treatment are compared between endovascular procedures and open thoracic surgery. Case series and registry data would appear to show that the endovascular procedures offer significantly lower mortality and morbidity rates when compared to open thoracic repair of thoracic aneurysms or acute dissections.1,2 The lack of randomised trials does, however, pose a problem for endovascular techniques, as much of the evidence base is composed of small retrospective series and registries, which do not offer sufficient detail to facilitate the subgroup analysis that is mandatory to refine indications for treatment.
Imaging of the thoracic aorta
The main imaging method used for the assessment of patients with thoracic aortic pathology is computed tomography (CT). Assessment should involve scrutiny of axial and multiplanar images. In general, the axial images are more useful images for assessment of aortic diameters and the multiplanar reconstructions are used for assessment of aortic length. In the present day, endovascular planning should be performed on a workstation that allows three-dimensional reconstruction and centre-line analysis. Magnetic resonance (MR) angiography does not have the required spatial resolution to facilitate the planning of endovascular procedures and there remains concern over the development of nephrogenic fibrosis when certain gadolinium agents3 are used in patients with renal failure. CT angiography, however, involves the use of ionising radiation and potentially nephrotoxic contrast agents, and as such is largely unsuitable for long-term imaging follow-up of patients with thoracic aortic disease. MR in general is therefore particularly useful for the follow-up of those patients with thoracic aortic disease.
Thoracic aortic aneurysms (TAAs)
The descending thoracic aorta is the most common location for aneurysms. Descending thoracic aneurysms are classified on the basis of whether they involve the upper half, the lower half or the entire descending aorta, with the thorax divided at the sixth intercostal space (types A, B and C).4
The majority of thoracic aneurysms are non-specific or degenerative, and approximately one-quarter are caused by chronic thoracic aortic dissections. Less frequently, aneurysms are related to Marfan syndrome, Ehler–Danlos syndrome, mycotic aneurysms5 and connective tissue disorders, e.g. ankylosing spondylitis, rheumatoid arthritis, Reiter’s disease and systemic lupus erythematosus. False aneurysms may follow trauma or complication of a previous coarctation repair. Aneurysms of the descending aorta may extend into the abdomen and are referred to as thoraco-abdominal aortic aneurysms.
Incidence and clinical presentation
Aneurysms of the descending thoracic aorta are a disease of increasing age and the male to female ratio is 3:1. TAAs are estimated to occur in 10 per 100 000 patient-years.6 Thoracic aneurysms are most frequently discovered incidentally on routine chest radiography or CT scans. Clinical presentations include substernal, back or shoulder pain, superior vena cava syndrome, dysphagia, dyspnoea, stridor and hoarseness (due to laryngeal nerve compression), and rupture. The survival of patients with untreated TAAs is bleak and is estimated to be 13–39% at 5 years.7 Eighty per cent of thoracic aneurysms detected at autopsy have ruptured. The risk of rupture increases with the size of the aneurysm, but data regarding estimated annual rupture rates and aortic diameter are sparse and less robust than for the abdominal aorta. Some data suggest that the rupture rate of thoracic aneurysms is 2.7 per 100 000 patient-years as compared to 9.2 per 100 000 patient-years for abdominal aneurysms.
Indications for treatment
The main indications for intervention are symptoms and size. There is controversy regarding the size criteria for treatment of TAAs. Juvonen et al. reported that the 2-year rupture rate for TAAs was 23% in aneurysms less than 7 cm,8 whereas Elefteriades observed a 30% 5-year rupture rate when the aorta exceeded 6 cm.9 Practically, most clinicians regard 5.5–6 cm as an indication for possible repair in an asymptomatic patient, the threshold obviously being balanced by the surgical risk. Diameter thresholds should be reduced in patients with defined connective tissue disorders as rupture may occur at smaller aortic diameters.
Endovascular repair of thoracic aneurysms
A segment of normal aorta above and below the lesion to be treated is required (landing zone), so that a seal can be achieved between the endograft and the normal aortic wall. The landing zone length should be at least 15 mm on the lesser curve, although 20 mm is optimal. With respect to the aortic arch, the landing zone length should be measured on the inner curve of the aortic arch and not the outer curve. If the landing zone is considered to be of inadequate length, surgical bypass may be performed to debranch the aortic arch or abdominal aorta so that an effective sealing zone is created. This is often referred to as a hybrid endovascular procedure and examples of surgical bypasses would include ascending aorta to innominate and left common carotid (Fig. 14.1), left to right carotid bypass (Fig. 14.2) and left carotid–subclavian bypass.
Figure 14.1 Operative photograph of ascending aorta to innominate and left common carotid. Surgery performed to create an adequate landing zone in a patient with a very proximal thoracic aneurysm.
Figure 14.2 Angiogram demonstrating a large thoracic aneurysm with the landing zone involving the left common carotid artery. To enable endovascular aneurysm repair a right to left common carotid bypass has been performed with ligation of the proximal left carotid artery (arrow).
The endograft delivery system is advanced over the guidewire to the desired deployment site. Accurate positioning is achieved by serial aortography. When the correct position is achieved, the stent graft is released (Fig. 14.2). Further endografts are placed as necessary with at least 4–5 cm of overlap between endografts.
Management of the spinal cord during endovascular thoracic procedures
One of the most feared complications of thoracic aortic intervention is paraplegia. Placement of an endograft in the thoracic aorta causes coverage of many critical intercostal vessels (T6–L1), with the risk of spinal cord ischaemia (SCI). Some centres advocate prophylactic CSF drainage whilst others adopt an expectant policy. No independent precipitating factor of SCI has been identified, although coexisting infrarenal pathology or previous abdominal aortic repair may reduce the collateral network supplying the spinal cord.10 Clearly, if there is any sign of SCI the cord perfusion pressure must be increased by reducing CSF pressure (target 10–12 mmHg) through spinal drain insertion, and increasing mean arterial pressure (MAP; target MAP 85–100 mm Hg) with inotropes.11 This selective approach appears safe for patients developing SCI after thoracic endovascular repair (TEVR), which has an acceptably low permanent neurological deficit, although overall survival of patients experiencing SCI after TEVR is diminished relative to non-SCI patients.12
Controversy has surrounded the management of the left subclavian artery during thoracic endografting. Early experience suggested that it was reasonably safe to cover the origin of the subclavian artery with respect to upper limb perfusion. However, more recent evidence has demonstrated that stroke and paraplegia rates are lower when subclavian perfusion is preserved, presumably due to the contribution of the vertebral artery to the anterior spinal artery.13,14 Routine revascularisation of the left subclavian artery (via left carotid–subclavian bypass) is recommended in the authors’ practice for the majority of cases where coverage of the left subclavian origin is planned,15 and this approach is supported by the recent recommendations from the Society for Vascular Surgery (SVS).
Outcome of treatment
Results of open repair in centres of excellence are good, with 30-day mortality rates for all types of thoracic aneurysm below 12% and paraplegia rates below 4%.16 Community results that incorporate several centres are more realistic of patient-centred outcomes and demonstrate 30-day mortality rates approaching 20%.17 Mortality is predicted by renal insufficiency, age and emergency presentation.18 Outcome data for EVR of thoracic aneurysms are available from several sources. Leurs et al., on behalf of the EUROSTAR collaborators, reported data in 249 patients with 30-day mortality for elective total endovascular repair (TEVR) of 5.3% and paraplegia of 4%.1 A cohort of patients with TAAs who underwent endografting with the Gore TAG device was compared retrospectively with the results of a cohort of 94 patients who underwent open surgical repair. The perioperative mortality (2.1% vs. 11.7%), paraplegia (3% vs. 14%) and freedom from major adverse event (48% vs. 20%) rates were all better in the endovascular group.18 Similarly, the European Talent Registry reported technical success of 98%, in-hospital mortality in 5% (4.1% and 7.9% for elective and emergency procedures respectively), paraplegia in 1.7% and stroke in 3.7%.19 Similar outcomes have been reported for the newest generation of endografts, despite the fact the patients in these later data series had more challenging anatomy compared with earlier series.13 Endovascular thoracic aneurysm repair has a spectrum of complications unique to the procedure. The most common is endoleak. There seems little doubt that the most common endoleak is an attachment site (or type 1) endoleak. These may occur immediately or during follow-up and should be treated with additional devices. Type 3 leaks (between adjacent devices or through disrupted grafts) may occur and should also be treated with additional devices.
Recommendations for practice
The management of patients with Marfan syndrome has been the subject of much debate since the introduction of endovascular therapy. Patients with Marfan syndrome develop both thoracic aneurysms and dissections. However, the patients are usually younger and fitter than patients with non-specific aneurysms, have excellent outcomes from conventional surgery20 and equivocal outcomes from EVR.21,22 At present, there is no body of evidence to support the routine use of EVR in patients with Marfan syndrome.
Thoraco-abdominal aortic aneurysms (TAAAs)
Thoracic aortic aneurysms that involve the visceral segment of the abdominal aorta are traditionally described as thoraco-abdominal aneurysms and are classified according to the extent of the aneurysmal disease (see Fig. 14.3 for a description of the Crawford classification). The aetiology of thoraco-abdominal aneurysms differs from infrarenal aneurysms, with medial degenerative disease and chronic dissection particularly prevalent.23 In contrast to infrarenal disease, the majority of patients with thoraco-abdominal aneurysms are symptomatic, the presence of back pain is particularly common, and this symptom may precede aortic rupture or intramural dissection.24 The rationale for treatment of thoraco-abdominal aneurysms largely derives from a natural history study from Crawford and DeNatale, who reported a series of 94 patients unsuitable for surgery.25 After 24 months’ follow-up, only 24% of these patients were alive, which contrasted with the 59% 5-year survival of a concurrent cohort of patients who underwent operative repair. On the basis of these results, it was concluded that patients with significant thoraco-abdominal aneurysms should have an operative repair unless precluded by coexistent medical conditions.
Surgical management
A left thoracotomy is usually required, although some type IV aneurysms may utilise an abdominal approach. The abdominal aorta is usually exposed by left medial visceral rotation. The diaphragm is partially divided with a circumferential incision preferred to preserve nerve supply. After the dissection has been completed the aneurysm is clamped at its proximal and distal extents, and an arteriotomy made in the lateral aneurysm wall. It is important that the arteriotomy is sited away from the visceral origins in the abdominal portion of the aneurysm. The proximal anastomosis is performed with a completely transected aorta to prevent aorto-oesophageal fistula. If possible, the proximal anastomosis is fashioned to include adjacent intercostal or visceral arteries, whilst any remaining intercostals are directly reimplanted into the graft or revascularised by separate jump grafts. The visceral arteries are then directly anastomosed into elliptical openings in the graft, utilising an inclusion technique. If possible, the coeliac, superior mesenteric and right renal arteries are taken on one patch; anastomosing the distal graft to the aortic bifurcation or iliac arteries completes the reconstruction (Figs. 14.4 and 14.5).
Figure 14.4 Operative picture demonstrating exposure of a type III TAAA using a left thoracolaparotomy. The diaphragm has been completely divided in this case. The left renal artery is controlled.
Adjunctive surgical techniques
During thoraco-abdominal aneurysm repair, prolonged visceral ischaemia is the main cause of postoperative renal dysfunction and can also contribute to multiple organ failure. To reduce the severity of organ hypoperfusion, partial left heart bypass with a centrifugal pump may be utilised to drain blood from the left atrium or upper pulmonary vein, and return it via the femoral artery or aorta. This facilitates renal and visceral perfusion whilst the proximal anastomosis is fashioned as long as the distal aortic clamp remains proximal to the visceral vessels.26 During the abdominal part of the aneurysm repair, the coeliac trunk, superior mesenteric artery and both renal arteries can be selectively perfused with catheters that are connected to the left heart bypass.27 Paraplegia may complicate thoraco-abdominal aneurysm repair in up to 20% of cases and is increased in more proximal aneurysms, with lengthy clamp time, renal impairment, advanced age and emergency presentations. Paraplegia results from damage to the spinal cord due to a combination of division of spinal cord arteries, prolonged spinal cord ischaemia, reperfusion injury and postoperative hypotension. Maintenance of spinal cord blood supply may be achieved by reimplantation of patent intercostal arteries and by distal aortic perfusion. CSF pressure increases during aortic clamping, and CSF drainage has been advocated to reduce paraplegia.28,29 A functional approach to the problem of neurological deficit aims at intraoperative monitoring of the spinal cord function. Somatosensory evoked potentials are widely used to detect spinal cord ischaemia during aortic cross-clamping and to identify vessels critical to spinal cord blood supply, which may then be perfused and reimplanted. An alternative approach is to use motor evoked potentials, which have been reported to improve paraplegia rates.30,31
Results of surgical repair of thoraco-abdominal aneurysms
The mortality and morbidity rates following conventional repair of TAAAs are still significant, with specialised centres reporting mortality rates of 5–16%, with paraplegia rates of 4–11%.32–34 However, these excellent results are not representative of outcomes outside of these centres, with national/community mortality rates exceeding 20% at 30 days and 30% at 1 year.35–37 Identifiable patient risk factors predicting adverse outcomes include emergent presentation, previous aortic replacement, diabetes, smoking history, renal and cardiorespiratory impairment, age beyond 80 years, acute dissection and the extent of TAAA.
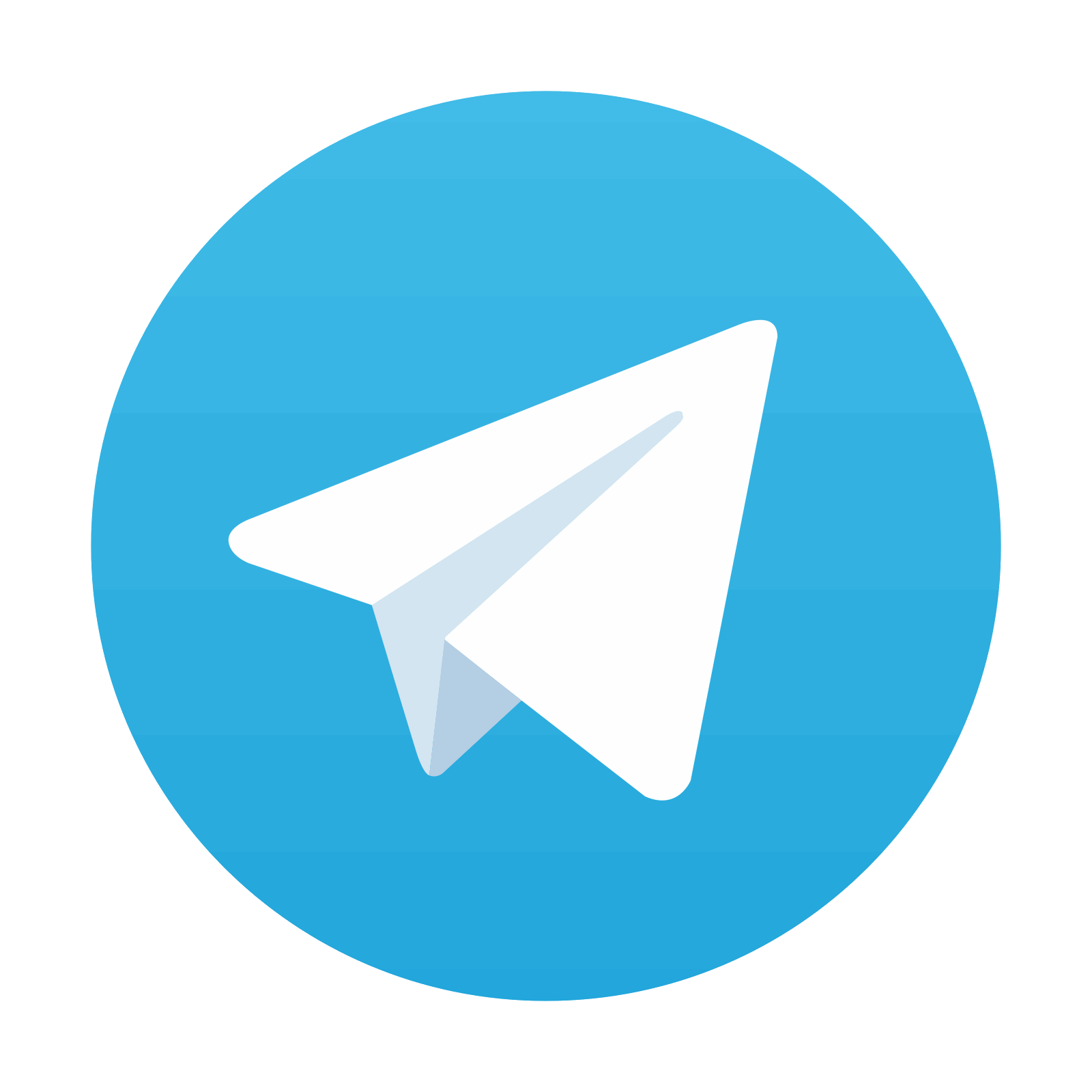
Stay updated, free articles. Join our Telegram channel

Full access? Get Clinical Tree
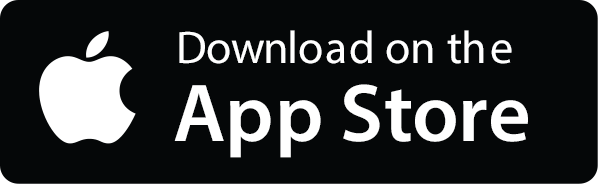
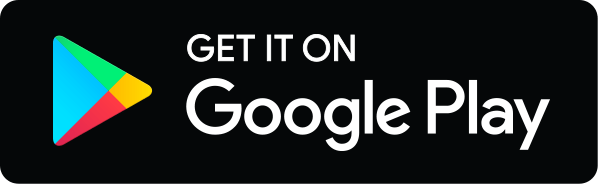