The Athlete’s Heart
Paul D. Thompson
N. A. Mark Estes
Overview
The athlete’s heart or the athletic heart syndrome is a constellation of clinical findings including sinus bradycardia, systolic flow murmurs, and cardiac chamber enlargement with normal or augmented function. All four cardiac chambers may be enlarged. Chamber enlargement may rarely exceed the upper limits of normal, but marked enlargement of the right ventricle or either atrium suggests a pathologic process. Clinical findings of the athletic heart syndrome are limited to athletes whose sports and training require a large aerobic or endurance exercise component. The left ventricular wall can be slightly thickened, but this thickening is generally restricted to athletes with left ventricular chamber enlargement and rarely reaches the thickness of pathologic, states such as hypertrophic cardiomyopathy (HCM). Physicians are often required to evaluate asymptomatic athletes prior to participation and to evaluate symptomatic athletes before permitting their return to vigorous exercise training. In these situations, the athletic heart syndrome must be differentiated from pathologic conditions associated with cardiac complications during exercise. These include HCM, coronary artery anomalies, aortic stenosis, and right or left ventricular cardiomyopathy in young athletes and coronary artery disease in adults.
Glossary
Athlete’s heart or athletic heart syndrome
A constellation of clinical, electrocardiographic, and echocardiographic variants of normal found in well-trained athletes who participate in sports requiring prolonged primarily aerobic exercise training.
Endurance or isotonic exercise
Generally rhythmic physical exertion such as running, swimming, or bicycling, which require significant sustained increases in oxygen uptake.
Strength exercise
Physical exertion requiring lifting or moving objects against resistance. Strength exercises can result in motion against resistance such as weight lifting or require exertion against a fixed object referred to as isometric exercise.
Maximal oxygen uptake
Physiologic and highly reproducible upper limit of an individual’s ability to extract and utilize oxygen during progressive isotonic exercise.
Onset of blood lactate accumulation (OBLA)
The point during exercise where the accumulation of lactate can be detected in blood samples. This point corresponds to an abrupt increase in the respiratory rate often referred to as the anaerobic threshold.
Historical Perspective
The athlete’s heart presently refers to normal cardiac adaptations that occur with prolonged, primarily endurance, exercise training, but the history of the athlete’s heart reflects the historical debate about the risks and benefits of vigorous exercise training and competition (1). Vigorous sports competition in the pre-Victorian era was largely an activity of the laboring class who competed in rowing, bicycling, and running events to test the skills required in their employment. There was little interest in such intense competition among the aristocracy and therefore little concern about its health risks. This changed during the Victorian era, when interest in competitive athletics increased, in part because sports were believed to develop what has been called the “most prized of Victorian virtues,
character” (2). The athlete’s heart in this period was primarily a moral concept referring to the character developed from athletic competition (2). Concern about the possible health risk of extreme exertion increased with growing sports participation among the aristocracy, and the appearance of such events as the Oxford–Cambridge boat race, first held in 1829 (2). In 1867, the “mechanic clause” was added to the rules of the British Amateur Athletic Club to exclude competitors whose work required physical labor. This made amateur athletic competition an activity for the elite (3). Articles on the dangers of the rower’s, runner’s and bicyclist’s heart soon followed (1). These concerns are understandable given the cardiac diagnostic techniques of the day, primarily by palpation of the pulse, percussion of the precordium for heart size, and auscultation for murmurs. The clinical characteristics of the athlete’s heart including sinus bradycardia, cardiac enlargement, and pulmonic and aortic flow murmurs were interpreted as heart block, cardiomyopathy, and valvular obstruction, respectively, conditions with poor prognoses until the later half of the twentieth century. Indeed, it was not until 1942 that the famed Boston cardiologist, Paul Dudley White, reported four cases of marked bradycardia in endurance athletes and concluded that this could be normal in endurance athletes (4).
character” (2). The athlete’s heart in this period was primarily a moral concept referring to the character developed from athletic competition (2). Concern about the possible health risk of extreme exertion increased with growing sports participation among the aristocracy, and the appearance of such events as the Oxford–Cambridge boat race, first held in 1829 (2). In 1867, the “mechanic clause” was added to the rules of the British Amateur Athletic Club to exclude competitors whose work required physical labor. This made amateur athletic competition an activity for the elite (3). Articles on the dangers of the rower’s, runner’s and bicyclist’s heart soon followed (1). These concerns are understandable given the cardiac diagnostic techniques of the day, primarily by palpation of the pulse, percussion of the precordium for heart size, and auscultation for murmurs. The clinical characteristics of the athlete’s heart including sinus bradycardia, cardiac enlargement, and pulmonic and aortic flow murmurs were interpreted as heart block, cardiomyopathy, and valvular obstruction, respectively, conditions with poor prognoses until the later half of the twentieth century. Indeed, it was not until 1942 that the famed Boston cardiologist, Paul Dudley White, reported four cases of marked bradycardia in endurance athletes and concluded that this could be normal in endurance athletes (4).
The term athlete’s heart appears to have been coined by a Swedish physician, S. Henschen in 1899 (5). He percussed cardiac size in cross-country skiers before and after a ski race and concluded that skiing produce a physiologic cardiac enlargement, which enabled the heart to perform more work than the heart of an untrained individual. He also noted that both the right and left sides of the heart were enlarged. Subsequent studies using roentgenographic, echocardiographic, computed tomographic, and magnetic resonance imaging techniques have only confirmed Henschen’s conclusion that exercise training produces a generalized cardiac enlargement.
How could Henschen, using only the “educated finger” for chest percussion, have reached such accurate conclusions? In sum, he picked the right athletes, and this fact highlights several clinical characteristics of the athletic heart syndrome. First, Henschen studied endurance trained athletes. Strength trained athletes, such as weight lifters, have increased cardiac dimensions, but their enlargement is related to body size and muscle mass (6). Only endurance trained athletes, or athletes training with both endurance and strength modalities, develop the athletic heart syndrome. Second, Henschen selected cross-country skiers, endurance athletes whose exercise uses both arm and leg muscles. Cardiac dimensions and stroke volume are greatest in those athletes whose training requires the use of the most muscle mass. This chapter summarizes the clinical principles of the athlete’s heart developed since Henschen’s report 100 years ago.
Basic Principles of Cardiovascular Exercise Physiology
Successful endurance athletes, compared to the general population, have a higher ability to perform maximal dynamic exercise, which can be measured as maximal oxygen uptake (VO2 max) (7). VO2 max is physiologically limited by the ability of the cardiopulmonary system to deliver, and the ability of the exercising muscles to use, oxygen. Rearranging the Fick equation for cardiac output (cardiac output = VO2/A – VO2 difference) demonstrates that VO2 max is the product of maximal cardiac output and the maximal arteriovenous O2 difference (7). Maximal cardiac output is the product of maximal heart rate and stroke volume. Because maximal heart rate among healthy individuals varies primarily by age and because the ability to increase the A – VO2 difference is limited, the major factor responsible for the higher VO2 max among endurance athletes is an increased stroke volume (7). The clinical findings typical of the athlete’s heart are generally manifestations of this increased stroke volume.
Although higher VO2 max values distinguish endurance athletes from sedentary individuals, among endurance athletes, VO2 max is not a good discriminator of superior athletic performance because VO2 max does not measure an individual’s ability to maintain exertion over a long period of time (7). Other factors including the athlete’s mechanical efficiency and the athlete’s anaerobic threshold or onset of blood lactate accumulation (OBLA) contribute to submaximal work capacity and are better discriminators of competitive exercise performance among athletes (7). Enhanced mechanical efficiency means that an athlete can perform a physical task while consuming less oxygen than a less efficient athlete. A higher OBLA means that the athlete can perform more mechanical work without producing lactate. OBLA is associated with a higher respiratory rate stimulated by the production of carbon dioxide from buffering lactic acid (HLactate + HCO3– → H2CO3 + lactate– → H2O + CO2). In addition, the lactate production indicates a level of exertion requiring glycogen catabolism and glycogen depletion is one potential limiting factor in endurance performance. Finally, lactic acid itself contributes to fatigue.
The increase in heart rate early during exercise is due primarily to withdrawal of resting vagal tone. At approximately 50% of maximal heart rate, additional acceleration of heart rate is associated with increased sympathetic activity (8). Peak heart rate is estimated as 220—age with a standard deviation of the estimate of ±11 to 22 beats per minute (BPM) (9) and 95% confidence limits of ±22 to 44 BPM. This emphasizes the problem of estimating heart rate by age. The increase in A – VO2 diff in produced by redistribution of blood from nonexercising tissue to the exercising musculature, increased extraction of O2 over the exercising muscle bed, and hemoconcentration (8). A change in any component of this system can affect exercise capacity. Cardiologists focus on changes in maximum heart rate and stroke volume, but changes in hemoglobin concentration, the ability to fully oxygenate the red cells, the capacity to shunt blood to exercising muscle, and the ability of the muscles to extract oxygen can affect exercise performance. All should be considered in evaluating athletes for problems with exercise performance.
Clinical Components of the Athlete’s Heart Syndrome
Variants Attributed to Enhanced Parasympathetic Tone
Several of the findings of the athletic heart syndrome including resting bradycardia, sinus arrhythmia, and atrioventricular (AV) conduction delay are attributed to changes in the sympathetic nervous system with enhanced parasympathetic and reduced sympathetic tone. Enhanced parasympathetic tone is most important (10). Athletes also may demonstrate ST-T wave changes of early repolarization and T inversions, which are also attributed to sympathetic nervous system alterations. Some of the T-wave changes can be quite bizarre and may be similar to those seen with conditions known to affect the parasympathetic nervous system such as subarachnoid hemorrhage (Figs. 37.1,37.2,37.3).
Many of the changes typical of the athletic heart syndrome, such as sinus arrhythmia and the ST changes of early repolarization, are also characteristic of young, healthy individuals, but
are more marked or more frequent in athletes and may persist into middle age among physically active subjects. All of these abnormalities in sinus rate, A-V conduction, and the ST changes of early repolarization should resolve with exercise with its attendant withdrawal of vagal tone and increased sympathetic activity. This is not always true for marked T-wave inversions (11), however; thus, their failure to resolve does not necessarily imply a pathologic process.
are more marked or more frequent in athletes and may persist into middle age among physically active subjects. All of these abnormalities in sinus rate, A-V conduction, and the ST changes of early repolarization should resolve with exercise with its attendant withdrawal of vagal tone and increased sympathetic activity. This is not always true for marked T-wave inversions (11), however; thus, their failure to resolve does not necessarily imply a pathologic process.
Sinus Bradycardia
Maximal VO2 and maximal cardiac output are increased in endurance athletes, but there is little change in resting oxygen consumption or cardiac output. Consequently, the larger resting stroke volume characteristic of endurance athletes permits a reduction in resting heart rate. Sinus bradycardia, generally defined as a heart rate less than 60 BPM, is typical of the athletic heart syndrome and reported in up to 91% of endurance athletes (12). The bradycardia in athletes can be profound, and a rate of 25 BPM has been reported in one distance runner (13). In addition to sinus bradycardia, sinus pauses or “sinus arrest” of more than 2 seconds have been documented during sleep in endurance athletes (12).
Sinus Arrhythmia
Sinus arrhythmia refers to variation in sinus rate with respiration. Specifically, the sinus rate decreases slightly at the start of the expiratory phase of the respiratory cycle. Sinus arrhythmia is common in young healthy subjects, but is more marked in endurance athletes.
Atrioventricular Conduction Delay
First-degree AV block, defined as a PR interval longer than 0.20 seconds is reported in 10% to 33% of endurance athletes (14). Second-degree AV block of the Mobitz I or Wenchebach pattern, characterized by progressive prolongation of the PR interval before a nonconducted P wave, is also seen more commonly in the athletic heart syndrome (14). Second-degree AV block with Mobitz II appearance, characterized by a nonconducted P wave without preceding PR prolongation, is not typical of the athletic heart syndrome. Mobitz type II block typically occurs at the level of the His–Purkinje system, whereas Mobitz type I block is due to progressive slowing of conduction in the AV node. AV block with Mobitz II appearance may occur in well-trained athletes owing to enhanced vagal tone, but this is rare (12). Its presence should prompt a search for other causes and should be attributed to the athletic training only if the athlete is asymptomatic and no other abnormalities are detected (Fig. 37.4).
The prolongation of the AV interval and decrease in AV conduction velocity described above may also unmask the ventricular preexcitation pattern, part of the Wolff–Parkinson–White (WPW) syndrome when accompanied by symptomatic arrhythmia. Indeed, a WPW conduction pattern is more common in endurance athletes (15). Cardiologists should be cognizant of this fact when evaluating athletes for an asymptomatic WPW conduction pattern because the risk of sudden death in asymptomatic subjects with this abnormality is low.
Vasovagal Syncope
Vasovagal syncope appears to occur more frequently in endurance-trained individuals related in part to their enhanced
vagal tone. Lower body negative pressure is a research technique used to examine blood pressure control and an individual’s response to orthostatic stress. Compared to nonathletes and strength-trained athletes, endurance-trained individuals have a reduced ability to maintain blood pressure during lower body negative pressure (16). The clinical implications of this are that endurance trained individuals are more vulnerable to vasovagal syncope or simple fainting and that positive tilt table responses are almost the norm in well-trained endurance athletes. These athletes have a large venous capacity from exercise training, enhanced vagal tone, and reduced sympathetic tone, all of which make them vulnerable to postural hypotension and a positive tilt table response. Tilt table results should not be interpreted as an adequate explanation for syncope in athletes, therefore, unless the clinical situation also strongly supports this explanation.
vagal tone. Lower body negative pressure is a research technique used to examine blood pressure control and an individual’s response to orthostatic stress. Compared to nonathletes and strength-trained athletes, endurance-trained individuals have a reduced ability to maintain blood pressure during lower body negative pressure (16). The clinical implications of this are that endurance trained individuals are more vulnerable to vasovagal syncope or simple fainting and that positive tilt table responses are almost the norm in well-trained endurance athletes. These athletes have a large venous capacity from exercise training, enhanced vagal tone, and reduced sympathetic tone, all of which make them vulnerable to postural hypotension and a positive tilt table response. Tilt table results should not be interpreted as an adequate explanation for syncope in athletes, therefore, unless the clinical situation also strongly supports this explanation.
Electrocardiographic ST-T Wave Changes
ST elevation of the “early repolarization pattern” is so common in endurance trained athletes that it should be considered the norm rather than the exception. Persistent training into advanced age can preserve this pattern in older athletes. ST depression, in contrast, is rare and should prompt a search for other causes. Peaked, biphasic, and inverted T waves in the precordial leads are also frequently seen in endurance athletes. The biphasic T waves typically occur in the precordial “transition” leads where the QRS complex is changing from a primarily negative deflection in the right precordial leads to primarily positive in the left-sided leads. Deeply inverted T waves can also be normal in athletes, but are rare and require the exclusion of significant disease (11). Among 952 healthy Italian national caliber athletes, only 27 athletes had marked T-wave inversions, 375 had abnormal or mildly abnormal electrocardiograms (ECGs), suggesting HCM in 11 and arrhythmogenic right ventricular cardiomyopathy in 16. Only one of these athletes actually had HCM, however (17).
Cardiac Arrhythmias in Athletes
Athletes frequently present for cardiac consultation because of palpitations. This is often sinus tachycardia, among the more
nervous subjects, or premature atrial or ventricular depolarizations (PVDs) detected by heart rate monitoring or palpation during athletic training. Occasional PVDs may be more frequent in athletes because the prolonged diastole provides extra time for such beats to occur. Premature beats are best treated with reassurance alone, but some athletes may require β-adrenergic blocking or other agents for symptomatic relief. Physicians should avoid β-blockers in elite athletes because they may affect athletic performance and are prohibited in sports such as archery and shooting because they prolong diastole and thereby reduce body movement produced by cardiac contraction.
nervous subjects, or premature atrial or ventricular depolarizations (PVDs) detected by heart rate monitoring or palpation during athletic training. Occasional PVDs may be more frequent in athletes because the prolonged diastole provides extra time for such beats to occur. Premature beats are best treated with reassurance alone, but some athletes may require β-adrenergic blocking or other agents for symptomatic relief. Physicians should avoid β-blockers in elite athletes because they may affect athletic performance and are prohibited in sports such as archery and shooting because they prolong diastole and thereby reduce body movement produced by cardiac contraction.
Atrial Fibrillation
Atrial fibrillation (AF) is unusual in young subjects, but may be more frequent in athletes (18). Among 1,160 individuals with AF, 70 (6%) had no evidence of heart disease, so-called lone AF, and 32 of these were endurance athletes (19). The onset of AF in athletes is often during sleep or postprandially, consistent with the concept that increased vagal tone from sleep and eating plus the exercise training contribute to its onset (19). AF in young, healthy endurance athletes is often transient and requires no treatment (18) once cardiac pathology is excluded usually by an ECG and echocardiography alone.
Ventricular Arrhythmias
It is not clear whether frequent or complex ventricular arrhythmias are a component of the athlete’s heart syndrome. Biffi et al. (20) divided 355 athletes with three or more PVDs on 12-lead resting ECG (n = 337) or palpitations (n = 18) into three groups based on ventricular arrhythmias (20). Arrhythmia in 230 athletes were detected by routine screening, whereas 125 other athletes were referred for suspected disease. Group A had 2,000 or more PVDs in 24 hours and one or more burst of nonsustained ventricular tachycardia (NSVT) (n = 71). Group B had 100 to 2,000 PVDs and no NSVT (n = 153). Group C had fewer than 100 PVDs and no NSVT (n = 131). The presence of cardiac disease increased with the arrhythmia frequency and was 30%, 3%, and 0% in groups A, B, and C, respectively. Group A athletes were disqualified from competition. The only death during a mean 8 years of follow-up occurred in an athlete who ignored her disqualification. The authors suggest that frequent PVDs, and even NSVT, may be a component of the athlete’s heart and have an excellent prognosis in the absence of cardiac disease. This is further supported by the observation that the frequency of arrhythmia decreased in 50 of the group A subjects with detraining (19), although such changes could simply reflect regression to the mean. Several points from these studies require emphasis. First, arrhythmias in most of these athletes were detected during screening, and the prognosis may be considerably different in symptomatic subjects. Second, the prevalence of disease increased with the frequency and complexity of the arrhythmia implying that the most extensive workups should be done in these athletes.
Evidence of Cardiac Enlargement
Habitual endurance exercise produces a global cardiac enlargement that may affect both the right and left atria and ventricles. The most consistent enlargement is seen in the left ventricular chambers where intracavity dimensions, and rarely wall thickness, can be large enough to raise the suspicion of cardiac disease. Mild enlargement of both atria and the right ventricle can occur, but marked enlargement of these structures suggests a disease process and is not observed in the athletic heart syndrome.
ECG Evidence of Chamber Enlargement
The ECG in well-trained athletes may show mildly increased P-wave amplitude suggesting right atrial enlargement, P-wave notching suggesting left atrial enlargement, incomplete right bundle branch block (RBBB), and voltage criteria for right and left ventricular hypertrophy (12). Among endurance athletes, voltage criteria for right ventricular hypertrophy are noted in 18% to 69% of subjects (12). This ECG evidence for atrial and right ventricular enlargement does not usually suggest extreme enlargement so that marked evidence of atrial or right ventricular enlargement should prompt a search for pathologic causes. Similarly, although incomplete RBBB is common, complete heart block is not generally accepted as part of the athletic heart syndrome (12). In contrast to ECG evidence of atrial and right ventricular enlargement, the voltage criteria for left ventricular hypertrophy can be extreme in endurance athletes (Fig. 37.5).
Echocardiographic Evidence of Cardiac Enlargement
Thomas and Douglas (21) summarized the topic of echocardiographic dimensions in athletes. At least 59 studies have used echocardiography to examine cardiac dimensions in athletes (21). These studies consistently documented increased left ventricular dimensions. Thirteen studies demonstrated that the right ventricular transverse dimension was increased an average of 24% in the athletes compared to controls (22 versus 17 mm) (21). Fourteen studies comparing the left atria of athletes and controls demonstrated that the transverse dimension was 16% larger in the athletes. Only one study to our knowledge has documented a larger right atrial size in the athletes (21).
Pelliccia et al. (22) examined left ventricular wall thickness in 947 elite Italian athletes including 209 women. Only 16 athletes (1.7%) had a left ventricular wall thickness greater than 12 mm, the upper limit of normal. Fifteen of these athletes were rowers or canoeists, sports that require both isotonic and isometric effort and involve a large muscle mass. These 15 athletes represented 7% of the rowers and canoeists studied. The only other athlete with increased wall thickness was a cyclist. All athletes with increased wall dimensions had won medals in international competition. The largest wall thickness in any athlete was 16 mm. All of the female athletes had wall thickness values below 11 mm.
Six of the athletes with marked left ventricular wall enlargement discontinued exercise training and were restudied after 40 to 240 (average 90) days of reduced activity. Average wall thickness decreased from 12.8±0.9 to 10.5±0.4 mm, P
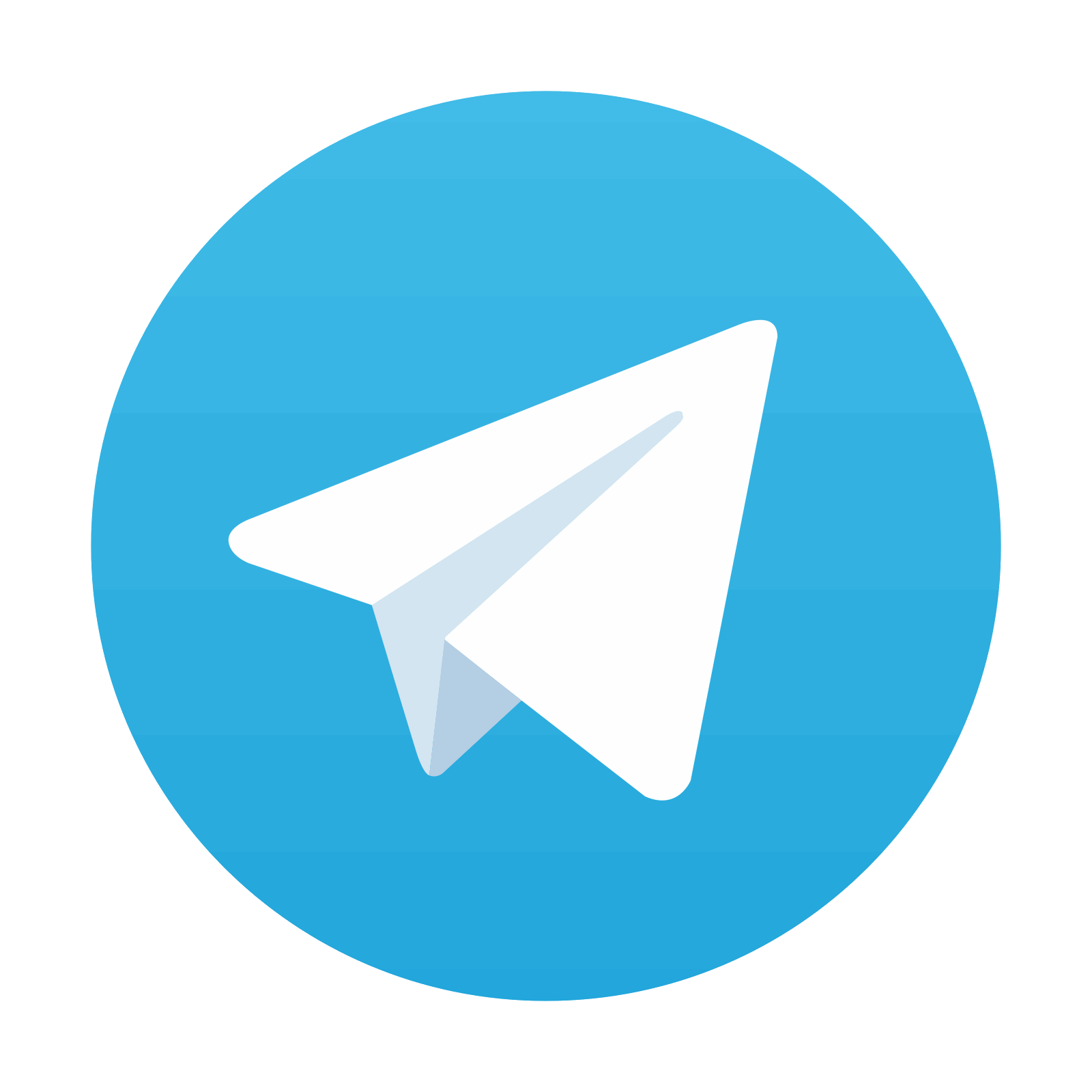
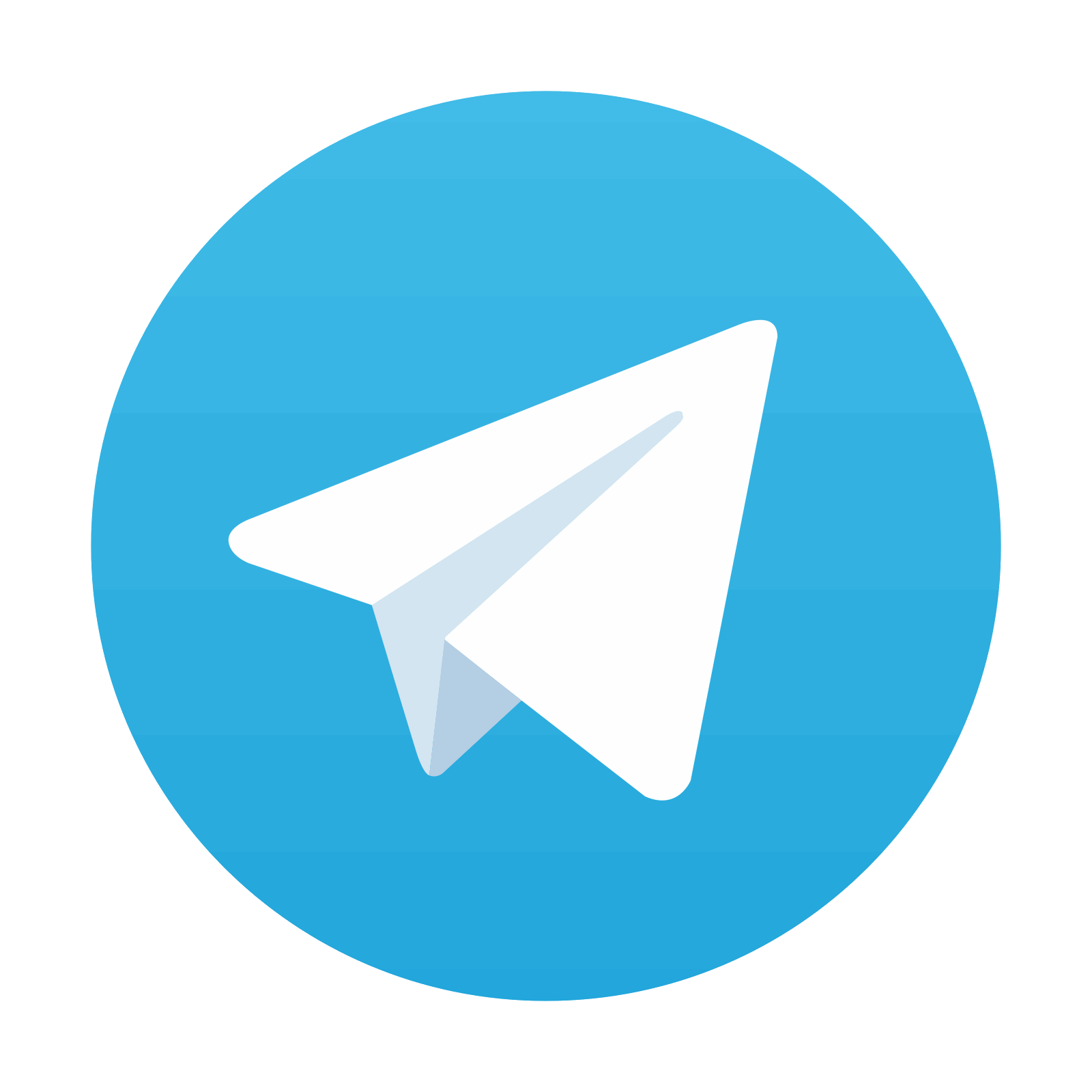
Stay updated, free articles. Join our Telegram channel

Full access? Get Clinical Tree
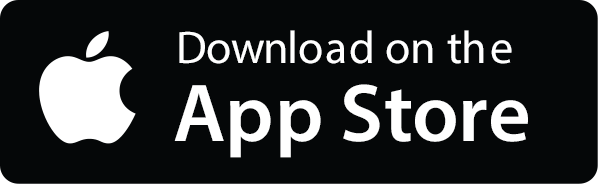
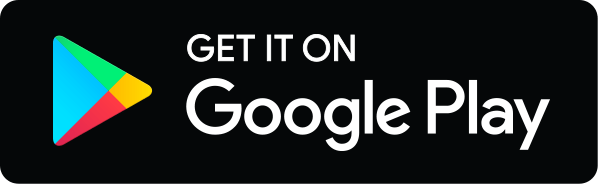
