Class I
1. Meticulous identification and control of fluid retention
2. Referral for cardiac transplantation in potentially eligible patients
3. Referral to an HF program with expertise in the management of refractory HF
4. Discussion of options for end-of-life care with the patient and family when severe symptoms persist despite application of all recommended therapies
5. For patients with implantable defibrillators, information about the option to inactivate fibrillation
Class IIa
1. Consideration of an LVAD as permanent or destination therapy in highly selected patients with an estimated 1-year mortality greater than 50 % with medical therapy
Class IIb
1. Pulmonary artery catheter placement to guide therapy in patients with persistently severe symptoms
2. The effectiveness of mitral valve repair or replacement is not established for severe secondary mitral regurgitation
3. Continuous intravenous infusion of a positive inotropic agent for palliation of symptoms
Class III
1. Partial left ventriculectomy is not recommended in patients with nonischemic cardiomyopathy
2. Routine intermittent infusions of positive inotropic agents are not recommended
Heart Transplantation
Shortly after Barnard [3] performed the first successful clinical heart transplant in 1967, several medical centers throughout the world established heart transplant programs [4, 5]. At that time, however, the promise of transplantation for treating end-stage heart disease was not fulfilled. Because little was known about transplant immunology and immunosuppression, most transplant recipients died of allograft rejection or opportunistic infection soon after surgery. Therefore, in the early 1970s, only a few centers, including Stanford, continued to perform heart transplants.
The 1980s saw renewed interest in heart transplantation because of the advent of cyclosporine. This potent immunosuppressant made it possible to combat rejection while minimizing the risk of infection [6]. Since then, techniques for detecting [7] and grading [8–10] the extent of rejection have been refined, and immunosuppressive protocols have been improved [11–20]. In the late 1990s, cyclosporine became available in a microemulsion formula (Neoral®) that improves drug absorption and has more predictable pharmacokinetics [15, 21, 22]. Newer immunosuppressants, such as tacrolimus and sirolimus, are being used as substitutes for cyclosporine in selected patients [12, 14, 16, 23]. Currently, the most effective immunosuppression regimens include a calcineurin inhibitor, an adjunct immunosuppressant (e.g., azathioprine, everolimus, or mycophenolate mofetil), and corticosteroids. Their use has reduced the risk of early graft loss due to acute rejection but has not completely solved the problem of late graft loss due to transplant coronary artery disease [18, 19]. Nevertheless, in the early twenty-first century, cardiac transplantation remains the best hope for many patients with end-stage heart failure [24–28].
Preoperative Considerations
Recipient and Donor Selection
Most patients referred for heart transplantation have an ischemic or idiopathic cardiomyopathy. Other indications include refractory valvular disease, congenital heart disease, primary myocardial diseases (e.g., sarcoidosis, amyloidosis), infection (e.g., Chagas’ disease), or drug-induced myocardial disease. In general, patients on the transplant waiting list are in New York Heart Association (NYHA) functional class IV and have a life expectancy of less than 1 year. Class III patients at risk for sudden death related to malignant arrhythmias can also be placed on the waiting list.
Additional selection criteria for transplant recipients vary from center to center. Over the years, the original criteria have been broadened, so that an increasing number of patients are deemed appropriate candidates [29]. For example, two previously excluded groups of patients, those aged ≥60 years [30–32] and those with diabetes mellitus [33–37], now have outcomes that compare favorably with those of routine transplant patients. However, patients with other terminal illnesses besides advanced heart disease are still ineligible for a transplant.
As the number of indications for transplantation has increased, so have attempts to expand the donor pool [38, 39]. Donors are matched with recipients according to ABO blood-type compatibility and, when necessary, other histocompatibility markers [40]. The criteria for donor selection have been expanded to include suitable donors ≥60 years of age [40–42]. In some urgent circumstances, donors receiving high doses of inotropic agents or donors who were potentially infected have been accepted [43–46], usually without adverse effects.
Immunosuppression
At our institution, the immunosuppressive regimen is based on triple-drug therapy consisting of calcineurin inhibitors, steroids, and mycophenolate mofetil. After transplantation, we taper steroid dosages to avoid side effects [47]. The monoclonal antibody OKT3, or the polyclonal antibody rabbit antithymocyte globulin (RATG), is used to induce immunosuppression in patients with renal dysfunction or to treat acute allograft rejection episodes [48].
Donor-Recipient Matching
Generally, we match recipients with donor organs on the basis of ABO compatibility. Gender and size matching are secondary criteria. The patient’s baseline pulmonary vascular resistance (PVR) is an important variable to consider when matching donor organs with recipients. The greatest danger, apart from immunological rejection, lies in primary graft failure. This most often involves failure of the donor right ventricle; hence, if the recipient has a higher-than-normal PVR (i.e., >2.5), we attempt to oversize the donor compared to the recipient. The goal is to attempt to find a heart from a donor with a donor: recipient weight ratio greater than 1.15.
Another critical factor to consider is whether the recipient has pre-formed antibodies to the potential donor. The field of donor antibody management at the time of transplantation has undergone a revolution in the past 10 years. Initially, antibody testing involved performing a complement-dependent cytotoxicity assay to determine immunologic risk at the time of transplantation. More recently, this method of antibody detection has been superseded by the introduction of solid-phase and flow cytometric assays, in which non–cell-based methods are used to detect pre-formed antibodies in the sera of potential recipients [49, 50].
Recipient Management While on the Waitlist
The increasing use of left ventricular assist devices to bridge waitlisted heart transplant candidates to successful transplantation is the greatest change in the management of these patients in the past 10 years. The proportion of patients who are now implanted with some form of ventricular assist device is approaching 50 % of all transplant recipients [51]. Managing patients with significant pulmonary arterial hypertension secondary to end-stage heart failure by implanting a left ventricular assist device (LVAD) and unloading the pulmonary vasculature and decreasing PVR has allowed our program to transition many candidates from the destination therapy strategy to transplantation.
Surgical Techniques
Through the years, the basic techniques for heart transplantation have remained the same [52–54]. In most cases, orthotopic transplantation is performed, but in selected patients, heterotopic transplantation may be necessary [53, 55, 56]. High PVR in the recipient or other conditions that might compromise donor heart function (e.g., a size mismatch in which the donor’s weight is 30 % less than the recipient’s) may warrant heterotopic transplantation. The native heart can then assist the donor heart during the initial period after transplantation.
Orthotopic Technique
For most of the cardiac transplantation era, biatrial orthotopic transplantation has been the standard, but the alternative bicaval technique has been gaining steadily in popularity [57, 58]. At many institutions, including the Texas Heart Institute, the bicaval technique is now the standard. Advantages of bicaval anastomosis include improved survival, atrial geometry, and hemodynamics and decreased valvular insufficiency, arrhythmias, pacing requirements, vasopressor requirements, and hospital stay [58].
Biatrial Anastomosis
Donor
In the case of multiorgan donation, the heart procurement team works along with kidney, liver, and lung procurement teams. After a median sternotomy and pericardial incision have been performed, the superior and inferior venae cavae are dissected up to the pericardial reflections, and 2-0 silk sutures are placed around the superior vena cava. At this point, the procurement team carefully evaluates myocardial function and inspects the heart for abnormalities. Then the other teams dissect the remaining organs to be donated. After heparinization (3 mg/kg) has been achieved, the superior vena cava is ligated, and the inferior vena cava is transected. Next, the aorta is cross-clamped, and cardioplegic solution (4 °C) is infused to induce cardiac arrest. During the operation, the myocardium is protected by means of topical cooling with 4 °C saline solution.
The right superior pulmonary vein or the left atrial appendage is incised to decompress the heart. Then the heart is removed, beginning with transection of the intrapericardial pulmonary veins, followed by transection of the left and right pulmonary arteries (just distal to the bifurcation) and the aortic root (at the level of the innominate artery). The pulmonary arteries and aortic root must be long enough to be anastomosed to the recipient’s vessels.
The heart is placed in a basin with ice-cold saline solution and is prepared for transplantation. The left pulmonary veins are connected to create a common left atrial opening (Fig. 21.1b, c), and the pulmonary artery is incised at the bifurcation. The lateral aspect of the right atrium is then incised from the opening of the inferior vena cava to the right atrial appendage. Care is taken to avoid injuring the sinoatrial node and atrioventricular conduction pathways. Finally, the heart is placed in double plastic bags containing ice-cold saline solution and then in an ice chest for transport to the recipient.
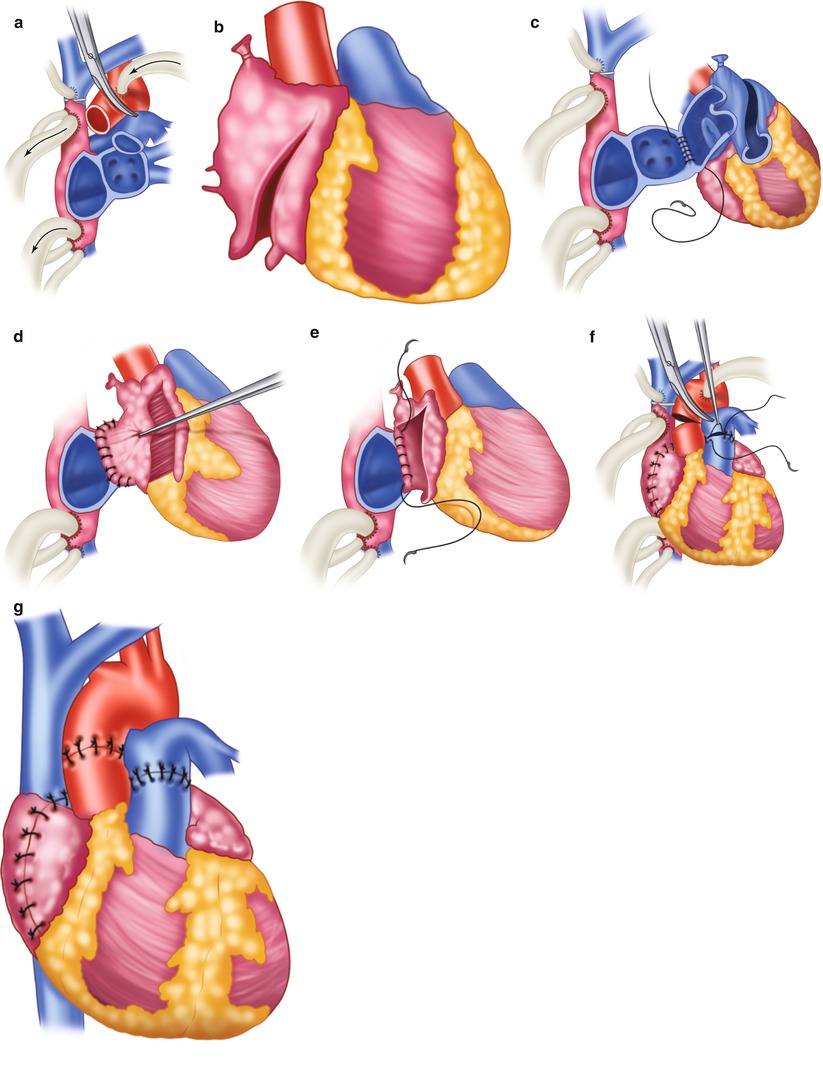
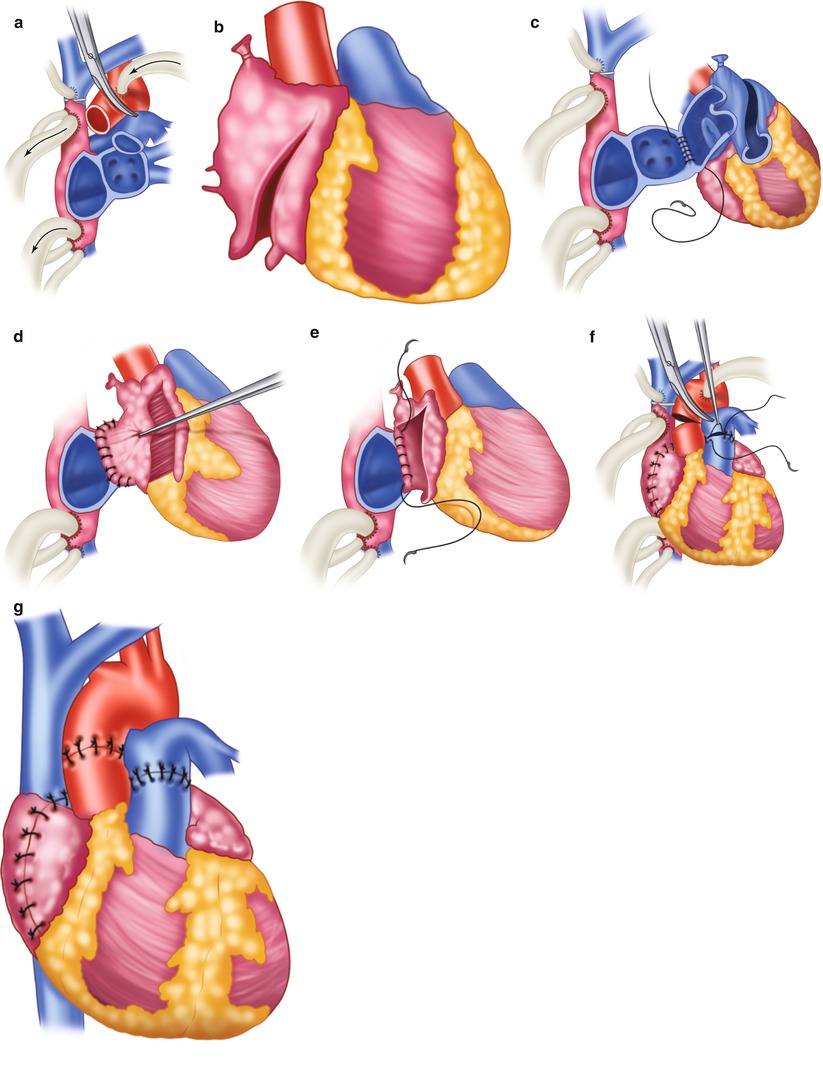
Fig. 21.1
Biatrial technique for orthotopic heart transplantation. (a) Excision of the native heart. (b) Preparation of the donor heart. (c) Left atrial anastomosis beginning at the free wall. (d) Completed left atrial anastomosis. (e) Right atrial anastomosis, beginning at the interatrial septum. (f) Pulmonary artery and aortic anastomoses. (g) Completed transplant
Recipient
After a median sternotomy and a pericardial incision have been performed, heparin (3 mg/kg) is administered intravenously. Cardiopulmonary bypass cannulas are placed in the ascending aorta at the base of the innominate artery and in the venae cavae via the right atrium. The caval cannulas should be close to the atriocaval junction but posterior enough to allow for the right atrial anastomosis. Cardiopulmonary bypass is instituted, and the operation is performed with the aid of systemic hypothermia (28–30 °C). Tourniquets around the venae cavae are tightened, and the aorta is cross-clamped.
The native heart is excised at the mid-level of the atria, just distal to the aortic and pulmonary valves (Fig. 21.1a), and the donor heart is brought to the operative field (Fig. 21.1b). The donor and recipient left atria are anastomosed with a continuous 3-0 monofilament polypropylene suture, beginning at the left lateral margin of the free wall and continuing toward the septum. The right atria are anastomosed in the same manner, beginning at the septum and continuing toward the free wall. While systemic warming is begun, the pulmonary artery and the ascending aorta are anastomosed end-to-end with 3-0 polypropylene sutures. The heart is then aspirated, and blood flow is restored by removing the aortic cross-clamp and releasing the caval tourniquets.
If the donor heart fails to resume normal sinus rhythm, electrical cardioversion should be performed. If the heart rate is slow, isoproterenol should be infused; if necessary, a temporary pacing wire should be placed on the right ventricle (100 bpm). If PVR is high, the isoproterenol infusion is continued. Nitric oxide and epoprostenol (Flolan) may also be helpful in this situation. The patient is gradually weaned from cardiopulmonary bypass, and the cardiopulmonary bypass cannulas are removed. Protamine sulfate is administered to reverse the effects of heparin, and the operation is completed in the usual manner.
Bicaval Anastomosis
Donor
In the bicaval technique, the right atrium of the donor heart is not incised [59]. Otherwise, the method for preparing the donor heart is generally similar to that used for biatrial transplantation.
Recipient
The method for preparing the recipient for biatrial transplantation is similar in most respects to that used in the biatrial technique, but with several important differences. In the bicaval approach, the recipient’s right atrium is totally excised, and the donor heart is anastomosed to the resulting inferior and superior vena caval cuffs (Fig. 21.2) [59]. Usually, the inferior vena cava is anastomosed after left atrial anastomosis, and the superior vena cava is anastomosed during the rewarming phase but before pulmonary artery anastomosis.
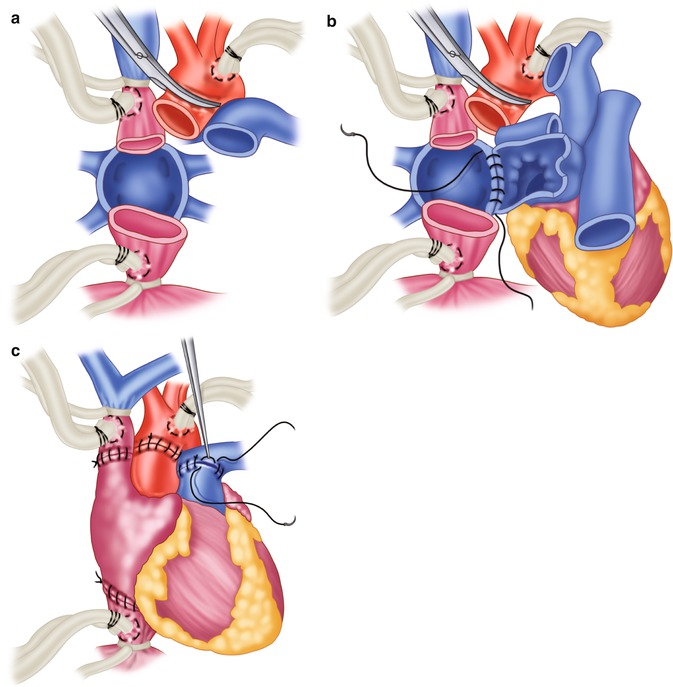
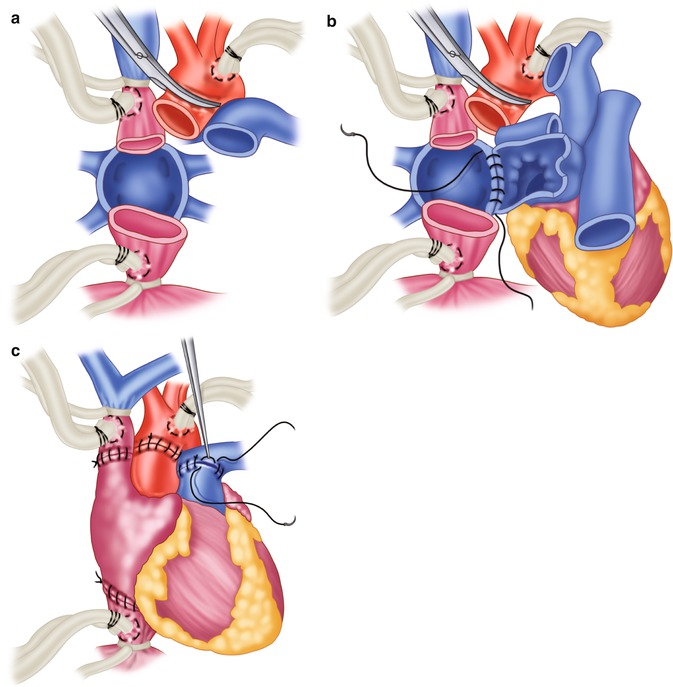
Fig. 21.2
Bicaval technique for orthotopic heart transplantation, as it differs specifically from the biatrial technique. (a) Total excision of the recipient’s atria. (b) First step in the anastomosis of the resulting inferior and superior vena caval cuffs. (c) Completed bicaval transplantation
Heterotopic Technique
Donor
The main difference in removing the donor heart for heterotopic transplantation is that the superior vena cava is ligated at the level of the innominate vein, allowing a larger opening to be created for the right atrial anastomosis (Fig. 21.3a). To prepare the donor heart for heterotopic transplantation, the inferior vena cava and the right pulmonary veins are closed with double-layer 5-0 polypropylene sutures. The bridge of tissue between the left pulmonary veins is incised to form a common opening into the left atrium. At the time of transplantation, an incision is made in the posterior right atrium and is extended to the superior vena cava.
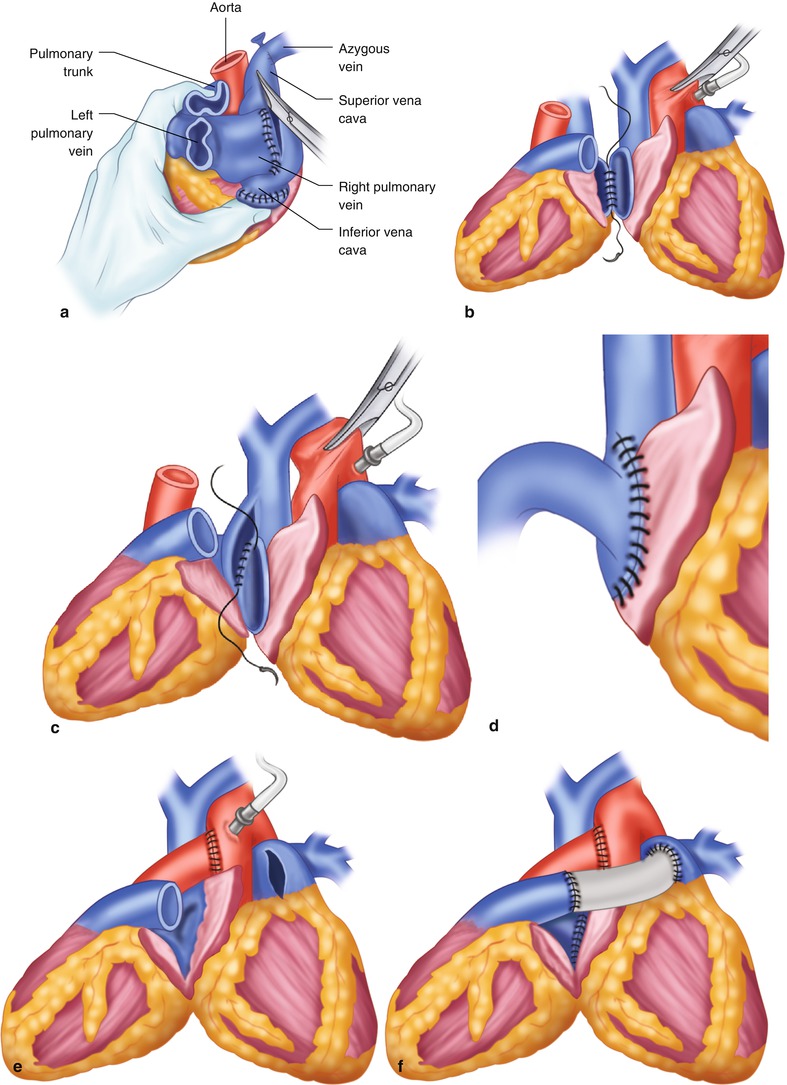
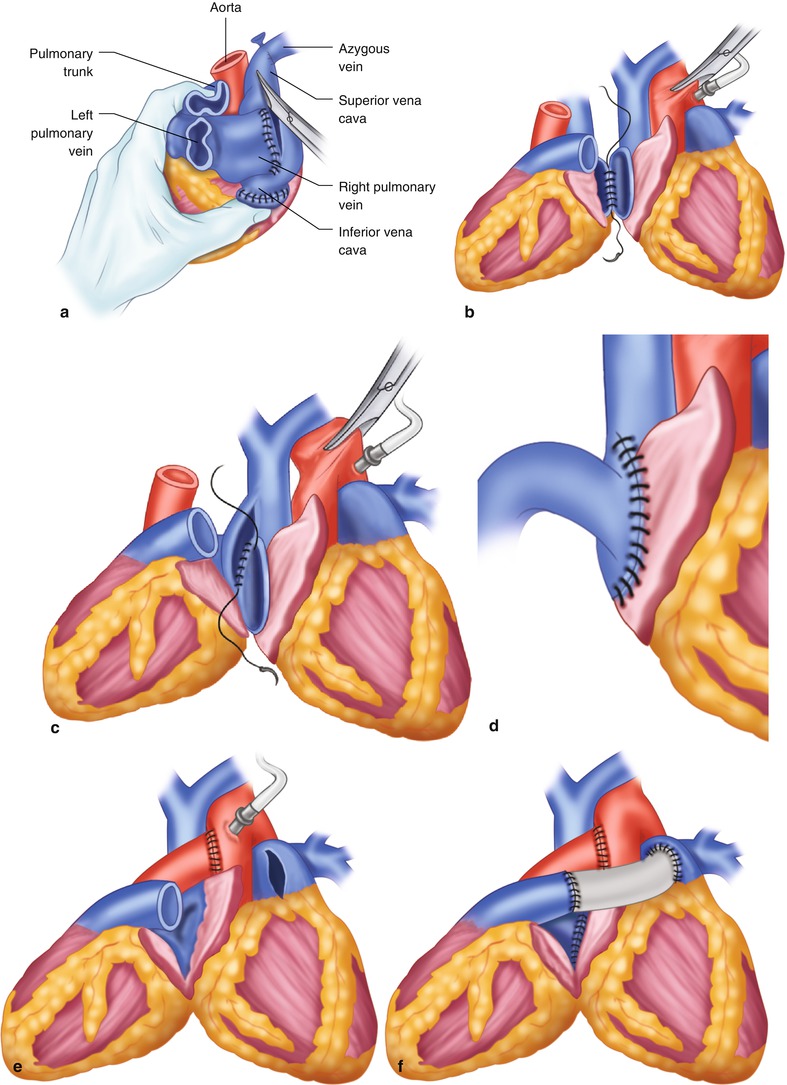
Fig. 21.3
Technique for heterotopic heart transplantation. (a) Preparation of the donor heart. (b) Left atrial anastomosis. (c) Right atrial anastomosis. (d) Completed right atrial anastomosis. (e) Aortic anastomosis. (f) Dacron graft sewn to donor and recipient pulmonary arteries to complete the heterotopic heart transplant
Recipient
After a median sternotomy has been performed, the pericardium is opened to the left of midline, and the incision is extended toward the right phrenic nerve. A rectangular flap is formed to allow the right lung to be isolated. Heparin is given, and the aorta is cannulated proximal to the origin of the innominate artery. The superior vena cava is cannulated through the right atrial appendage, and the inferior vena cava is cannulated at the atriocaval junction. Cardiopulmonary bypass is started, the ascending aorta is cross-clamped, and tourniquets around the venae cavae are tightened. Systemic hypothermia is induced, and the heart is stopped by infusing cardioplegic solution through the aortic root. During the operation, topical cooling protects the myocardium.
The transplant begins with the left atrial anastomosis (Fig. 21.3b). The left atrium of the native heart is incised between the right superior and inferior pulmonary veins. The left atrial openings of the hearts are joined, beginning posteriorly, with a continuous running suture. To prevent a gradient across the anastomosis, the left atrial incision in the donor heart may have to be extended to create a wider opening.
The native right atrium is incised to the atriocaval junction. The incision is continued into the superior vena cava, so that two thirds of the length of the incision is located on the cava. A large diamond-shaped anastomosis is created between the right atria, beginning at the lowest point of the donor incision and the posterior midpoint of the incision in the native right atrium (Fig. 21.3c, d).
The donor’s aorta is then anastomosed end-to-side to the native ascending aorta (Fig. 21.3e). An adequate length of donor aorta is necessary to prevent kinking of the atrial anastomoses. The pulmonary arteries are joined with a preclotted 22-mm Dacron graft. The graft is anastomosed end-to-side to the recipient pulmonary artery trunk, then end-to-end to the donor’s pulmonary artery (Fig. 21.3f).
Air is removed from both hearts, and blood flow is restored. The function of both hearts must be checked before cardiopulmonary bypass is stopped. Finally, temporary pacing wires are placed on both hearts to establish a satisfactory rhythm and to monitor electrocardiographic activity. Using a dual-chamber pacemaker with adjustable atrioventricular delay, one can easily institute counterpulsation to treat hemodynamic instability and minimize inotropic drug use immediately after heterotopic heart transplantation. Moreover, long-term linked pacing may lead to improvements in native heart function and, thus, offer better back-up support in case of allograft dysfunction [60].
Early Postoperative Management
Patients are carefully monitored for hyperacute allograft rejection or other complications that could cause donor heart failure. Right ventricular failure can result from pulmonary hypertension. Therefore, if PVR increases at the time of weaning from cardiopulmonary bypass, aggressive measures are taken to increase ventilation and to correct acidosis. Pulmonary pressure may be reduced with isoproterenol or prostaglandin E1 (PGE1). Again, nitric oxide and epoprostenol may also be helpful in this regard. If right or left ventricular failure occurs, mechanical circulatory support is provided with an appropriate assist device [61–66].
Outcomes and Clinical Results
Most heart transplant recipients enjoy a good quality of life [67, 68], and most of them (particularly the older ones) have a reasonable life expectancy. In following up 62,952 adult heart transplant recipients from 1982 through June 2003, the Registry of the International Society for Heart and Lung Transplantation [69] reported overall estimated survival rates of 80.4 % at 1 year, 66.8 % at 5 years, and 47.8 % at 10 years. Estimated early mortality (within 30 days of transplantation) is 10.3 %.
To further improve long-term survival, transplant researchers have been focusing on analyzing and preventing allograft coronary atherosclerosis [23, 70–78]. This disease, which is one of the primary causes of death after transplantation, seems to be associated with allograft rejection. Furthermore, since the number of donor hearts has remained fairly constant (approximately 2,000–2,500 in the United States between 2000 and 2012) [79], researchers are seeking ways to increase the donor pool and optimize the use of available hearts [38, 39, 41, 80, 81]. Nonetheless, donor scarcity will continue to limit the number of patients who can undergo heart transplantation. Moreover, a large number of patients with end-stage heart disease do not meet the transplant selection criteria. Advances in long-term mechanical circulatory support are offering hope for solving these dilemmas.
Mechanical Circulatory Support
The history of mechanical circulatory support has paralleled, and often overlapped, that of heart transplantation. Experience with the total artificial heart (TAH) has stimulated the evolution of ventricular assist devices and vice versa.
In 1969, Cooley [82] performed the first clinical implant of a TAH (Fig. 21.4). The pump served as a bridge to transplantation in a 47-year-old man who could not be weaned from cardiopulmonary bypass. After 64 h, the patient underwent a successful heart transplant but died of Pseudomonas pneumonia and overwhelming sepsis 32 h later. Twelve years would pass before another TAH was implanted, again by Cooley [83]. In this case, the TAH was used as a bridge to transplantation in a 36-year-old man who had had severe cardiac dysfunction after undergoing double valve replacement. The patient was supported by the TAH for approximately 30 h and then underwent transplantation. He survived for another 7 ½ days before succumbing to overwhelming sepsis. The third Texas Heart Institute patient to receive a TAH as a bridge to transplantation was a 41-year-old man [84], who was supported by a Jarvik-7 TAH for 31 days. Despite infectious complications that arose soon after the transplant, the patient recovered, was discharged from the hospital, and lived for 2 years.
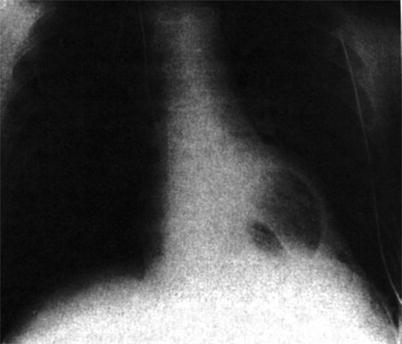
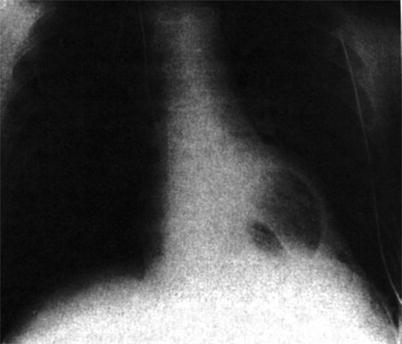
Fig. 21.4
Roentgenogram obtained after the first clinical implantation of a total artificial heart in 1969 by Cooley
In 1982, William DeVries [85] performed the first of five permanent Jarvik-7 TAH implantations. Although the TAH was able to provide total circulatory support for prolonged periods, the patients had to remain hospitalized, tethered to control consoles. In addition, device-related complications, particularly infections and strokes, supervened. Only two of the five patients to receive permanent TAHs lived for more than 1 year afterward [86].
In 1988, the smaller Jarvik 7–70 TAH model was introduced, and 75 implantations were performed that year [48]. By 1991, a total of 230 TAHs had been implanted in 226 patients, including five patients who received the device on a permanent basis. Today, the successor of the Jarvik-7 TAH, now known as the CardioWest pump (SynCardia Systems, Tucson, AZ), is approved for use by the United States Food and Drug Administration (FDA) as a bridge to transplantation.
In the 1970s, problems related to TAH development and cardiac transplantation stimulated interest in long-term left ventricular assistance. Several research centers began to work on pumps for treating patients with advanced heart disease characterized primarily by left ventricular failure. This effort was stimulated by the work of DeBakey [87], who first used the LVAD as a bridge to recovery in 1963. By 1978, Texas Heart Institute investigators were conducting clinical tests of an abdominally implanted pneumatic LVAD in patients with cardiogenic shock [88]. One patient, a 21-year-old man with “stone heart” syndrome, was supported for 5 days before transplantation, proving that the LVAD could maintain circulation in the absence of left and right ventricular function [89]. In the 1980s, with the emergence of cardiac replacement as the standard of treatment for end-stage heart failure, LVADs became widely used as bridges to transplantation. Increasing clinical experience has led to the introduction of improved pulsatile devices and a new generation of continuous-flow devices with the potential for long-term use as bridges to transplantation, destination therapy, or both.
Pulsatile LVADs
Two pulsatile LVADs have been approved by the FDA for use as a bridge to transplantation in patients with advanced heart failure: the HeartMate and the Novacor. Both are similar in design, function, and placement. In 2002, the HeartMate was approved for use as destination therapy.
HeartMate
Designed for long-term or permanent left ventricular support [90–92], the HeartMate was available in both pneumatically and electrically powered versions, the pump being identical in each. Clinical trials in bridge-to-transplant patients were initiated for the pneumatic model in 1986 and for the electric model in 1991. On the basis of these trials, both models were later approved for commercial use.
The intra-abdominally implanted HeartMate pumped blood from the left ventricle into the ascending aorta. The titanium pump, which measured 11.2 × 4.0 cm, was divided by a flexible diaphragm into a blood chamber and a pumping chamber. It had a maximal stroke volume of 83 mL and could provide flows of up to 11.6 L/min. For pneumatic support, the pump was controlled with an external portable console; for electric support, transcutaneous electric leads were attached to rechargeable batteries, which were worn in a shoulder holster. With the electric HeartMate, patients who achieved NYHA class I status were able to await cardiac transplantation at home, even returning to full-time employment in some cases. One patient lived comfortably for more than 500 days with this device [93, 94].
The HeartMate’s unique features included its specially textured surfaces within the blood chamber. As blood flowed through the chamber, elements from the blood adhere to the surfaces, so that, within a few days of pump implantation, a smooth biologic coagulum would begin to form [95, 96]. As a result, strokes—which had hindered long-term support with other devices—were minimized. Moreover, patients could be maintained on antiplatelet therapy, consisting of dipyridamole (75 mg tid) and aspirin (80 mg/day), without the need for other anticoagulants.
Although infection can be a problem with any type of LVAD [97], this complication does not preclude survival to transplantation or reduce the survival rate after transplantation [69, 98, 99].
Indications
The indication for HeartMate implantation was a rapid deterioration in hemodynamic status despite maximal pharmacologic support, intra-aortic balloon support, or both. Specific selection criteria included a pulmonary capillary wedge pressure of ≥20 mmHg, a cardiac index of ≤2 L/min/m2, and a systolic blood pressure of ≤80 mmHg.
Conditions that precluded HeartMate treatment included severe pulmonary hypertension, irreversible renal dysfunction, hepatic dysfunction, and a small body surface area (<1.5 m2).
Surgical Techniques
Implantation Procedure
The HeartMate pump (Fig. 21.5a) is normally implanted through a median sternotomy incision that extends to the umbilicus [100]. (It may also be implanted through a left thoracotomy technique, which may be especially useful in patients in whom resternotomy would greatly increase mortality and morbidity risk [101].) After heparin (3 mg/kg) is administered, cardiopulmonary bypass is instituted. The right atrium and ascending aorta are cannulated for cardiopulmonary bypass. Alternatively, the femoral artery can be cannulated to provide arterial return, which then frees the ascending aorta for anastomosis of the outflow graft. Administering low-dose PGE1 before the start of cardiopulmonary bypass helps prevent increased PVR and resultant right ventricular failure. Use of nitric oxide has helped to reduce PVR without causing the systemic effects produced by prostaglandins.
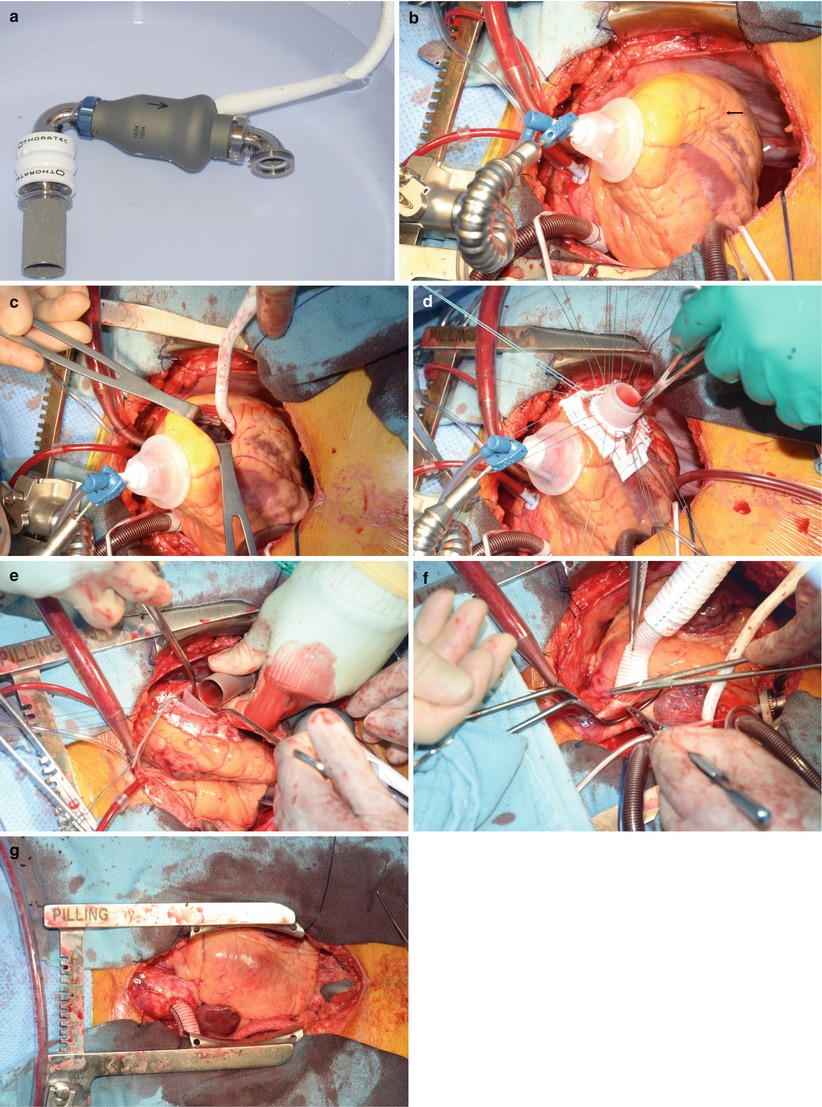
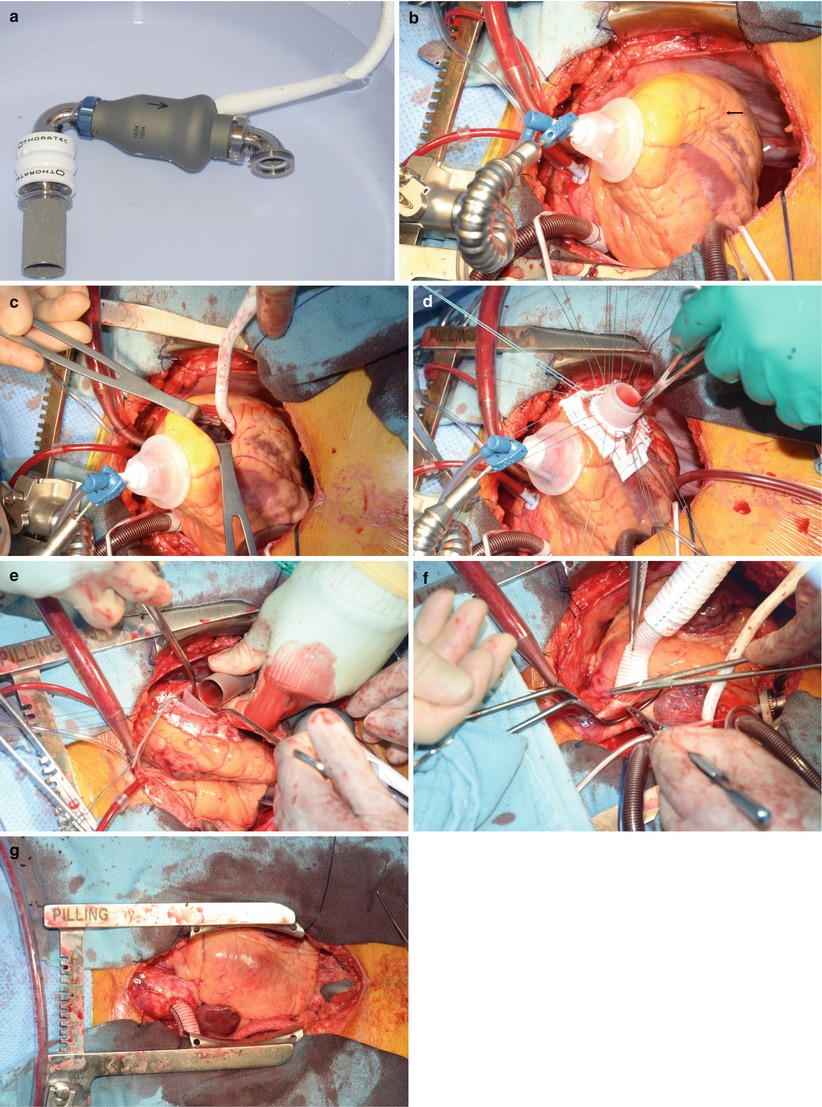
Fig. 21.5
Implantation of the HeartMate II continuous flow ventricular assist device (VAD). (a) The HeartMate II, the most widely used VAD today. (b) Placement of the VAD on the diaphragmatic surface of the ventricle (arrow). This is the ideal location because it avoids septal irritation of the inlet, which can result in arrhythmias. (c) Coring of the insertion site. This allows easy inspection of the ventricle and, in cases of severe mitral regurgitation, Alfieri stitch placement. (d) The Silastic inlet in place. (e) The pump, placed intraabdominally and wrapped with omentum. The inlet is placed through the diaphragm and firmly secured. (f) Proper length obtained for the outlet graft, shown here sewn to the ascending aorta. (g) Final placement of the pump. In this position, the device can easily be avoided if the patient subsequently undergoes heart transplantation
After cardiopulmonary bypass has been instituted, the ascending aorta is cross-clamped, and cold cardioplegic solution is rapidly infused. Systemic hypothermia is maintained at 28 °C, and cold saline solution is applied topically to protect the heart during the operation.
The pump is positioned intraperitoneally or extraperitoneally in the left upper quadrant of the abdomen. The pump inflow and outflow conduits are attached to the device with rotatable joints, so that the conduits can be adjusted to fit the patient. Each conduit contains a 25-mm porcine valve to produce unidirectional flow.
After localization (Fig. 21.5b) of the proper site of implantation on the diaphragmatic surface of the ventricle (parallel to the septum, anterior to the papillary muscle origin), the insertion site is cored with a special circular knife (Fig. 21.5c). A Teflon-covered, reinforced Silastic sewing ring is then sutured to the opening with interrupted, pledgeted sutures (Fig. 21.5d). Next, the inflow and outflow conduits are connected to the blood pump (Fig. 21.5e, f). The inlet conduit is tunneled through an incision in the diaphragm, aligned with the mitral valve, and then secured to the sewing ring. The outflow conduit, a polyester graft, is anastomosed end-to-side to the ascending aorta with a running 4-0 polypropylene suture. To ensure proper seating of the device, the outflow graft must be trimmed to an appropriate length. Therefore, the percutaneous driveline is exteriorized and the device is positioned before the graft is cut. The driveline is tunneled subcutaneously from the left lower quadrant to the right upper quadrant exit site. The long tunnel may help to prevent serious driveline infections. The graft is cut so that it lies over the diaphragm, just to the right of the median sternotomy. Proper placement of the graft helps prevent complications during the resternotomy for transplantation.
If the pump is placed intraperitoneally, the omentum is wrapped around the pump and the intraperitoneal portion of the driveline to prevent bowel adhesion, and the pump is secured to the abdominal wall (Fig. 21.5g).
Before LVAD pumping is initiated, the patient is placed in the Trendelenburg position. The outflow graft is cross-clamped near the anastomosis, and air is slowly vented from the system with a 19-gauge needle placed in the outflow graft. Pump flow is started and gradually increased, while cardiopulmonary bypass flow is gradually decreased. Transesophageal echocardiography helps confirm proper placement of the inflow graft.
The unloading action of the LVAD introduces a new physiologic state in which the left atrial pressure decreases to below normal while the right atrial pressure remains high. In patients with a previously asymptomatic patent foramen ovale, LVAD pumping may cause the foramen to become symptomatic and produce significant shunting. Such an opening, which is best detected with transesophageal echocardiography, must be closed before the patient leaves the operating room.
At the end of the operation, the effect of heparin is reversed, thoracic drainage tubes are placed, and the sternotomy and laparotomy incisions are closed.
Explant Operation
At transplantation, cardiopulmonary bypass is instituted. Cannulation may be done via the femoral artery to allow more room for the aortic anastomosis. The native heart is excised in routine fashion, and the outflow graft is completely removed to prevent infection. If a heterotopic procedure is planned, the left ventricular apex is oversewn with a Dacron patch and pledgeted sutures. After the pump has been removed, the transplant is performed routinely.
Outcomes and Clinical Results
By March 2004, the 4,190 patients worldwide had received a HeartMate—1,323 pneumatic, 2,867 electric—as a bridge to transplantation. Many of the recipients required prolonged support (>30 days). In the multicenter clinical trials that eventually led to FDA approval, both HeartMate models substantially improved the clinical status of patients [102–104]. In most instances, those in NYHA class IV returned to class I within 3–4 weeks after LVAD implantation. In a nonrandomized multicenter trial involving 75 pneumatic HeartMate recipients and 33 non-LVAD patients [102], the HeartMate reduced pretransplantation mortality by 55 %; it also improved the 1-year posttransplantation survival rate to 90 %, compared with 67 % in the control group. In a similar trial comparing 280 electric HeartMate recipients and 48 non-LVAD patients, the 1-year posttransplantation survival rate was 84 % versus 63 % [104]. Both of these studies used a concomitant control group, albeit historical, consisting of patients who met the criteria for LVAD implantation but for whom the device was not available. In the control groups, survival was achieved only by means of cardiac transplantation.
The successful experience in the bridge-to-transplant population led to the HeartMate being tested as destination therapy in patients with end-stage heart failure who were not candidates for transplantation. The NHLBI-sponsored REMATCH (Randomized Evaluation of Mechanical Assistance and Treatment of Congestive Heart Failure) trial showed that treatment with an LVAD is superior to optimal medical treatment alone [105].
Novacor
The pulsatile Novacor pump was first used clinically as a bridge to transplantation in 1984 [106]. A wearable version was introduced in 1993. In 1998, the device’s inflow conduit was modified, reducing embolic events by 50 %. Like the HeartMate, the Novacor pump was implanted intra-abdominally and delivered blood from the left ventricle into the ascending aorta. By 2005, more than 1,500 Novacor pumps had been implanted worldwide. The pump appeared reliable; one patient was supported for more than 6 years. The Novacor was approved as a bridge to transplantation in the United States and as a bridge to transplantation, bridge to recovery, and destination therapy in Europe [107–110].
With the advent of continuous flow devices, these large, pulsatile, intracorporeal devices are largely of historical significance only. Both of these devices are no longer available commercially, nor are they supported by their respective manufacturers.
Continuous-Flow LVADs
Pulsatile pumps are relatively bulky, which limits their use in smaller patients. They also have many moving parts, which may invite mechanical failure and thus limit their longer-term use. Newer pumps are being developed that are smaller and easier to implant, have fewer moving parts, and require less power to run [111]. These pumps utilize axial flow technology that allows pulseless, continuous flow. The resulting diminished pulse-pressure circulation has been shown to be safe in humans [112]. Our institution has extensive experience with three of these pumps, as described below.
Jarvik 2000
The Jarvik 2000 Heart (Jarvik Heart, Inc., New York, NY) consists of a thumb-sized blood pump, an external controller, and portable batteries. The pump, which is connected to the external controller by a power cable, has a single moving impeller enclosed within a titanium housing. The pump operates at speeds of 8,000–12,000 rpm and can provide up to 6 L/min of continuous flow under optimal physiologic conditions [113]. The Jarvik 2000 is implanted directly into the apex of the left ventricle via a midline sternotomy, left thoracotomy, or subcostal approach [114]. An outflow graft connects the pump to the ascending or descending aorta.
Development of the Jarvik 2000 began in 1988, and clinical trials of this pump as a bridge to transplantation began in April 2000. The Jarvik 2000 has been implanted in 106 patients in Europe and the United States, including 80 patients at the Texas Heart Institute.
HeartMate II
The HeartMate II (Thoratec Corp., Pleasanton, CA) consists of a blood pump, an external system driver, a power base unit, and portable batteries. The blood pump contains a single moving, electromagnetically powered rotor. An inflow graft delivers blood from the left ventricle to the pump; an outflow graft delivers blood from the pump to the ascending aorta. A driveline connects the pump to the external system driver. This system uses the same power base unit and portable batteries as the electric HeartMate. The HeartMate II operates at 6,000–15,000 rpm and provides up to 10 L/min of continuous cardiac output [115]. The implantation technique for the HeartMate II is similar to that of the electric HeartMate and requires a median sternotomy [115].
Development of the HeartMate II began in 1991. Its design was based on that of the axial-flow Hemopump used for short-term cardiac support [116]. In the first clinical trials of the HeartMate II, in Europe in 2000, recurrent thrombogenesis was a problem; this led to several design changes. In November 2003, a redesigned version was implanted by surgeons at the Texas Heart Institute in an 18-year-old man suffering from dilated cardiomyopathy [115]. The patient was successfully rehabilitated and discharged home in April 2004 to await a transplant.
The HeartMate II has since been implanted in 14,000 patients worldwide. Results have been favorable, with few complications (thrombogenic or otherwise). This is currently the only pump approved by the FDA for use as both a bridge to transplant and destination therapy.
The HeartWare HVAD (Fig. 21.6) is a miniaturized centrifugal pump that can be inserted into the left ventricle without necessitating the creation of a pump pocket. The hope is that the lack of a pump pocket will result in fewer infections. The pump operates at fewer rpm than the earlier axial flow designs and has no wearable parts. There is one moving impeller that rotates at speeds between 1,800 and 3,400 rpm. The centrifugal design uses a hydrodynamic/passive magnetic suspension mechanism that should minimize trauma to the blood elements. This device was approved for use as a bridge to transplant in December 2012. It is currently in clinical trials for use as a destination therapy device.
