Stem Cells and Respiratory Disease: Prospects for the Future
INTRODUCTION
Advances in stem cell research offer unprecedented opportunities to develop new treatments, disease models, and drug screens for previously untreatable conditions. These discoveries have captivated the imagination of the lay press and have inspired hope in patients, clinicians, and scientists. Inevitably, in an emerging new field, there are false starts that accompany promising discoveries. If rigorous researchers in the field have difficulty discerning hype from hope, what is a busy clinician to do when confronted with basic questions, such as: “Is stem cell therapy available for my lung disease?” or “Shall I bank my baby’s cord blood in case he/she develops cystic fibrosis or emphysema?”. This chapter is designed to describe the quickening pace of stem cell and regenerative medicine research related to lung disease and to place the latest discoveries in a historical context, before discussing future prospects.
Stem cells have been found in an increasing number of tissues whose biology is characterized by rapid turnover of differentiated cells. In these tissues, for example, blood, skin, and intestine, a stem cell hierarchy has been described where rare stem cells proliferate occasionally, giving rise to stem cell daughters or to progenitors that can proliferate rapidly, and differentiate into mature cells required for the function of that tissue. These properties of self-renewal and differentiation are the classic hallmarks of stem cells, and their importance in homeostatic maintenance of the blood, skin, and intestine, are well accepted.1–3 These features also make stem cells attractive vehicles for clinical applications such as the reconstitution of injured or diseased tissues. Several decades of research focused on animals and humans, including the seminal bone marrow transplantation work of Till and McCullough4 has rigorously proven, for example, that the hematopoietic stem cell, a cell that comprises 1 in 10,000 bone marrow cells, can be delivered to a recipient by simple intravenous infusion, reconstituting all cells of the bone marrow and circulating blood for the life-time of the recipient.1
Given the virtually unlimited self-renewing capacity and blood differentiation repertoire of hematopoietic stem cells, most pulmonologists are surprised to learn that cells of similar capacity have not been reproducibly proven to exist in the lung and may not be necessary for the homeostasis of an organ with a quiescent epithelium that contrasts with the rapidly self-renewing epithelia of intestine and skin.
LUNG EPITHELIAL RESPONSES TO INJURY
Because the unperturbed adult lung epithelium displays remarkably slow cell turnover, the post-injury or disease responses of human or animal lung have been studied to elucidate both the proliferation potential and differentiation repertoire of various lung cell types. These injury models have been used to search for potential specialized lung cells that might exhibit stem cell properties, such as self-renewal and multipotent differentiation (reviewed by Rawlins and Hogan).5 Beginning in the 1970s, morphologic studies of human and animal lungs defined subsets of lung epithelia with proliferative capacity, revealing basal, secretory, and club cells (previously called Clara cells) of the proximal airway, and type 2 alveolar cells of the distal lung parenchyma all had the capacity to enter cell cycle in response to lung injury.6–14 More recently, thymidine-labeling techniques have been replaced by newer methods of identifying proliferating cells or tracking their progeny. These studies have emphasized that most lung epithelial cells, except for airway ciliated cells or type 1 alveolar cells, can proliferate after injury in a remarkably resilient organ where many differentiated epithelial subtypes contribute to tissue repair.5,15–18
Beyond assessing the proliferation of cells in the adult lung, the classic developmental biologist’s method of “lineage tagging” cells has allowed investigators to follow the origin of lung epithelial cells during development, and to assess where they go upon ageing or after injury.15,19–21 It is now well established that the many types of epithelial cells in the adult lung arise during development from a few progenitor cells in the embryonic foregut endoderm.22 The concept has become generally accepted that a limited number of lung epithelial cell types are specified during embryonic development and sustain their committed differentiated state throughout life.21 In the nascent field of stem cell research, most investigators agree that multipotent, bona fide lung epithelial progenitors exist, if only fleetingly early in embryonic development.22,23 It remains unclear, however, whether any specialized progenitors or stem cells similar to these embryonic endodermal precursors remain in the lung postnatally or are rederived after injury.
EVOLVING STUDIES IN THE SEARCH FOR LUNG STEM CELLS
With so many proliferation-competent epithelial cells in the adult lung able to participate in post-injury repair, perhaps equally, some investigators have begun to wonder whether some cells are “more equal” than others. The search for specialized reparative lung cells in recent years has been propelled by the emergence of specific and sensitive molecular biology tools and laboratory instruments that can refine our previous understanding of lung biology (Fig. 8-1). New studies suggest that a much greater diversity exists within the previously identified broad classifications of lung airway and alveolar epithelial cells.24 For example, the response of mouse lung to recover from naphthalene exposure, an injury that depletes the lung of most club cells (identified by the marker club cell secretory protein [CCSP; also known as Scgb1a1 or CC10]), revealed a small subset of airway progenitors, called “variant club cells” (previously variant Clara cells).25,26 These cells expressed CCSP, but were resistant to naphthalene injury and rapidly reconstituted both secretory and ciliated populations of the injured airway. Most intriguing was the observation that, this rare subset of CCSP+ cells appeared to reside in two specialized microanatomical locations or niches: adjacent to neuroepithelial bodies of the airways and at broncho-alveolar duct junctions. This observation remains one of the most convincing examples to date, suggesting the lung contains progenitor niches, or microanatomical cell compartments with specialized stem or progenitor cells.
Figure 8-1 Lung epithelial stem and progenitor cell candidates. Schematic of proposed lung epithelial candidate stem or progenitor cells and their niches in the proximal conducting airways and distal alveoli. Cells whose localization or existence is not yet clear or accepted are indicated with dashed boxes and/or question marks. NEB, neuroepithelial body; BADJ, bronchoalveolar duct junction; Gland, submucosal gland duct; AEC2, type 2 alveolar epithelial cell; Marker abbreviations used for each cell subtype include the following: Itg, integrin; K, cytokeratin; CCSP, club cell secretory protein; SPC, surfactant protein-C. (Modified with permission from Kotton DN: Next-generation regeneration: the hope and hype of lung stem cell research. Am J Respir Crit Care Med. 2012;185(12):1255–1260.)
One remarkable and controversial study by Kim et al. has proposed that some of these rare CCSP+ progenitors located at the bronchoalveolar duct junction may possess the capacity to reconstitute both airway and alveolar epithelia, suggesting the lung contains rare, multipotent, “bronchoalveolar stem cells” (BASCs).27 However, the existence of BASCs has been contested in later work by Hogan et al.19 who employed lineage tagging to trace the progeny of club or variant club cells expressing the CCSP marker which would presumably include the putative BASC population. These investigators found that these cells, either following an injury or during normal fetal lung development, gave rise only to conducting airway but not alveolar epithelia. In later work, Hogan et al. found that under some circumstances, such as following bleomycin-induced lung injury in mice, CCSP+ lineage tagged cells could give rise to alveolar epithelial cells. This confusing literature continues to cause controversy and uncertainty in the field and has dampened enthusiasm for the concept of a lung stem cell hierarchy that might follow the classical paradigm of hematopoietic stem cells of the blood-forming bone marrow.
In the more proximal airways, such as the mouse trachea or human bronchi, investigators have also found remarkable subsets of basal cells that contain extensive proliferative potential and a multipotent differentiation repertoire consisting of the capacity to give rise to basal, secretory, and ciliated lung epithelial cells.28,29 With the advent of modern flow cytometry, these cells can now be sorted to purity, allowing the cataloging of protein markers (such as p63, NGFR, CK5, or CK14) that identify these cells as well as the delineation of their global transcriptome by microarray analysis.28,30 The capacity to purify, expand in culture, and differentiate basal cells has resulted in a number of publications in recent years with important implications for those searching for lung cells with stem or progenitor potential.28,30,31 First, these studies have confirmed that heterogeneity and diversity within the previously limited subsets of lung epithelial cells is indeed much greater than that appreciated a decade ago.24,32 Second, the studies have revealed the impressive proliferative potential and differentiation repertoire of some subtypes of single lung epithelial cells (such as basal cells), which can expand almost indefinitely in vitro, similar to classically studied stem cells of the skin.28,31 Taken together, this body of work supports the concept that basal cells can function as tissue-specific stem cells of the proximal conducting airway epithelium.
While there is a quickly growing list of stem/progenitor candidate cells able to reconstitute the conducting airway epithelium, the type 2 alveolar epithelial cell to date remains the best accepted progenitor of the lung alveolus.33 Basic scientists are on the trail of new candidate alveolar progenitors, however, as evidenced by a notable new study demonstrating the clonal expansion in culture of cells from human or mouse lungs that express basal cell markers yet possess both airway and alveolar differentiation potential in vitro.31 The authors who described these cells have proposed them as candidate alveolar stem cells, and other labs are working on reproducing these findings before their differentiation repertoire or nomenclature can be established. Most importantly, the p63+ or CK5/14+ lung stem cells described in this exciting study appeared to emerge during recovery from influenza-induced injury in regions of distal lung alveoli, an area of the lung that does not normally harbor cells expressing the basal cell markers p63 or CK5. Other recent studies have utilized flow cytometry to isolate new candidate lung progenitors, identified by coexpression of alpha6 and beta4 integrins.34 This population appeared to proliferate in response to lung injury, and after purification from mouse lungs displayed remarkable potential for multipotent airway and alveolar differentiation.
While the therapeutic application of these progenitor populations remains years away, it is already clear that the lessons learned from studying the biology of progenitor cells in both mouse models and humans with lung disease can be applied to clinical settings. For example, many publications now demonstrate that communications between some of the best studied lung epithelial progenitors and their neighbors, either in the epithelium or surrounding lung mesenchyme, helps to regulate the fine balance between progenitor self-renewal and differentiation.17,32,35 Perturbations in these communications, controlled by familiar developmental signaling molecules (such as Wnt, Notch, FGF, retinoic acid, and TGFβ) disturb the balance of lung epithelial homeostasis36 and contributes to the disordered histopathology and physiology of many lung diseases, including cystic fibrosis, emphysema, idiopathic pulmonary fibrosis, and asthma.32,37,38 The increased understanding provided by studying the basic biology of lung progenitors and epithelial–mesenchymal interactions has thus allowed a more complete understanding of clinical disease.37,39 and already is being applied for the development of new pharmaceuticals designed to modulate the above signaling pathways. The long-term goal of achieving sophisticated drug therapies for a variety of lung diseases is likely to ultimately involve activation of endogenous lung progenitors or mature epithelia to accomplish reparative reepithelialization to avoid pathologic lung remodeling.40
Common to most of the aforementioned studies evaluating the differentiation repertoire of purified lung progenitor candidates is the suggestion that if an effective technique for grafting these cells into injured lungs were available, these progenitors might be used to regenerate injured epithelia in patients. The prospect of engineering entirely new bioartificial lungs from these cells has also been proposed to meet the needs of growing numbers of patients with end-stage lung diseases who require lung transplantation. Pioneering studies in tissue engineering41,42 have attempted to develop methods for preparing lung scaffolds or bioartificial lungs that might surmount this highest hurdle in our field: a method for delivering candidate progenitors or lung reparative cells in vivo for engineered lung regeneration. For example, the laboratories of Harald Ott and Laura Niklasson in 2010, both published a technique of engineering bioartificial lungs through the method of detergent-based decellularization of rodent lungs. This method leaves the 3D architecture of the lung matrix intact while stripping away rodent cells. This matrix then served as a scaffold upon which rodent or human cells were adhered to generate “recellularized” lung tissue able to carry out gas exchange in vitro or even in vivo after transplantation.41,42
CONTROVERSIAL CLAIMS OF PARADIGM SHIFTING STEM CELL RESEARCH DISCOVERIES
This aforementioned studies of lung epithelial injury, proliferation, and regeneration are slowly defining and refining our understanding of candidate lung epithelial progenitors, but there are occasional studies that challenge earlier paradigms of lung development and repair after injury. For example, the laboratory of Anversa et al. in 2011 reported the discovery of a rare cKit+ lung stem cell that they claim can be purified from human lung tissue, can be expanded indefinitely in simple culture media supplemented with serum alone, can be differentiated into multiple lung epithelia by adding dexamethasone, and most importantly, can be injected into injured mouse lungs giving rise to essentially all lung tissues, including mesodermally derived vasculature and endodermally derived airway and alveolar epithelia.43 This property of naturally occurring pluripotency, defined as the capacity to give rise to multiple cell types across multiple germ layers, is without proven precedent in any adult tissue outside the germline and contradicts many decades of developmental biology research, according to some leaders in this field.44
Perhaps it is easiest to understand this new work when placed in the historical context of other prior studies that also initially proposed to shift paradigms in lung biology, including the work of this writer45 who found that advances in techniques and methods often reveal established paradigms to be correct, and data suggesting paradigm shifts often have simple, alternative explanations that are not obvious at first glance.46 In the 1990s and early 2000s, for example, a wave of publications claimed that, cells within the bone marrow, such as marrow stromal cells (also known as mesenchymal stem cells [MSCs]) or hematopoietic stem cells, could circulate to the lung and give rise to almost any type of differentiated lung epithelial or vascular endothelial cell. Similar findings were reported when examining injured hearts, brains, livers, and other organs, creating understandable excitement (reviewed by Weiss et al. and Wagers and Weissman32,47
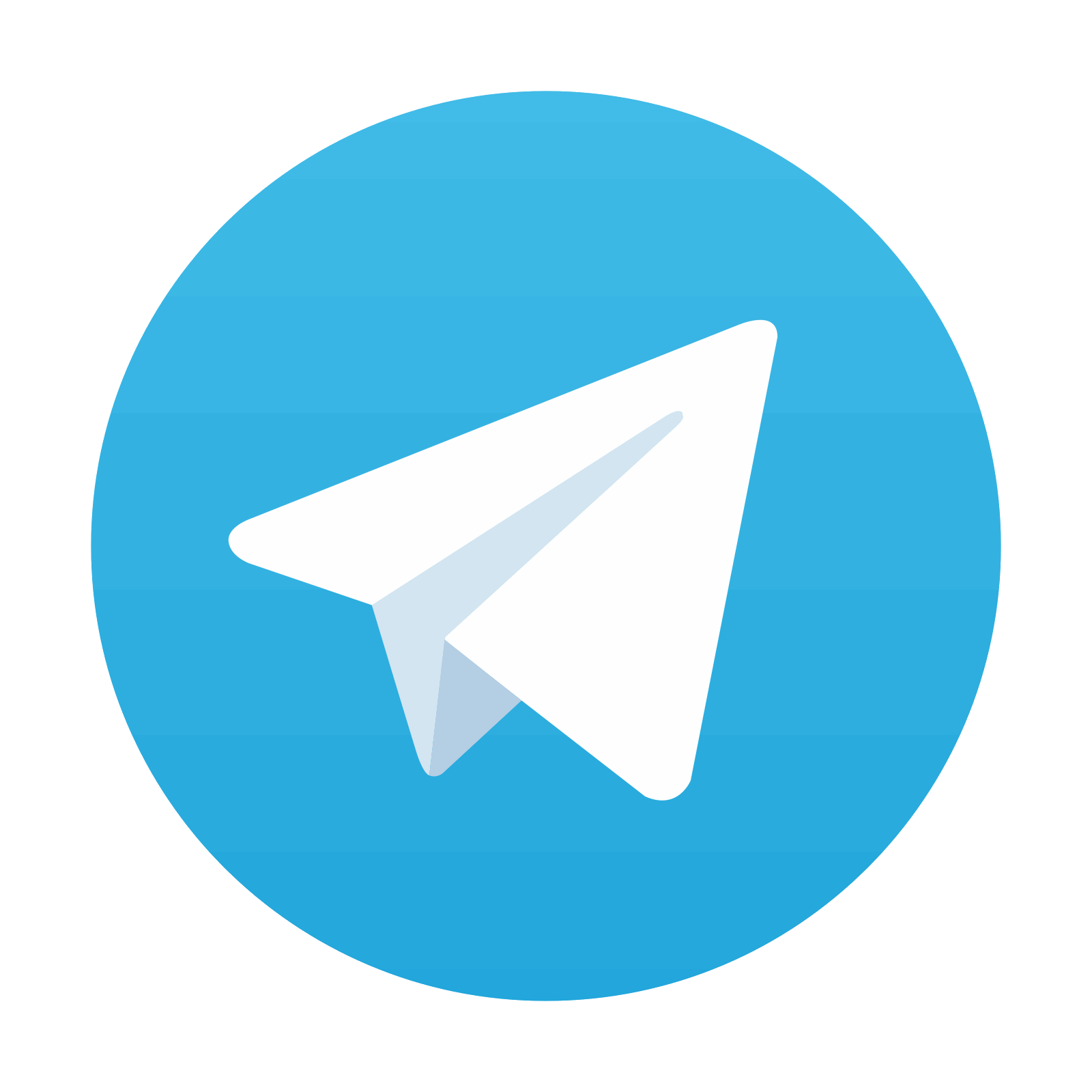
Stay updated, free articles. Join our Telegram channel

Full access? Get Clinical Tree
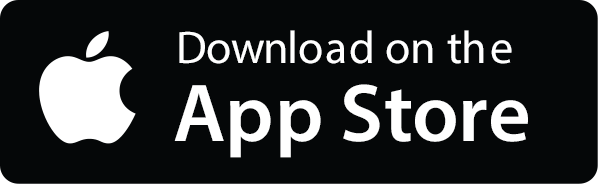
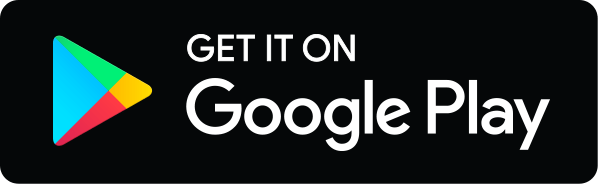