Chapter 11 State-of-the-Art Instrumentation for PET and SPECT Imaging in Small Animals
MICRO–POSITRON EMISSION TOMOGRAPHY
Dedicated, high spatial resolution small-animal PET imaging devices have become available. Some are in-house-developed systems at several research institutions,1–5 but more recently, dedicated small-animal systems have become available through several commercial suppliers. The spatial resolution of these imaging devices is typically in the range of 1.0 to 1.5 mm full-width at half-maximum (FWHM) and enables mapping of the distribution of positron-emitting radiotracer and its changes over time throughout the body of small animals. These imaging systems have been used quite extensively for measuring substrate metabolism and cell replication rates of tumors as indices of tumor growth rates and tumor aggressiveness, and for determining responses of tumor growth to investigational therapeutic agents.6–10 Other research has utilized these small-animal imaging devices for the development and validation of novel noninvasive assays for identifying activation and expression of transgenes.6,9–11
POSITRON EMISSION TOMOGRAPHY IMAGING OF THE RODENT HEART
The small dimensions of the rodent heart pose formidable challenges for PET studies of the cardiovascular system in rodents. In general, dedicated small-animal PET systems employ two different technical concepts: (1) crystal detector–based and (2) multiwire proportional chamber–based systems.12,13 As shown in Figure 11-1, multiwire proportional chamber–based systems may offer superior spatial or volume resolution but generally are less sensitive and require longer image acquisition times. Conversely, crystal-based systems offer higher sensitivity (count rate per unit radioactivity) and offer superior temporal resolution but at the expense of somewhat lower spatial resolution (Fig. 11-2).
Several commercial small-animal scanners are now available since the first prototype scanner was built at University of California, Los Angeles. Figure 11-3 shows one such small-animal PET scanner, the microPET Focus 120.14 This system consists of 96 scintillation detectors arranged in four rings with a 14.7-cm diameter and 7.6-cm axial extent. Each detector consists of a 12 × 12 array of 1.52 × 1.52 × 10 mm lutetium oxyorthosilicate (LSO) crystals coupled to a position-sensitive photomultiplier tube (PS-PMT) via optical fiber bundles. Data are acquired in three-dimensional (3D) mode. With standard filtered backprojection reconstruction algorithms, the system achieves an isotropic (in all three directions) spatial resolution of 1.5 mm in the center of the field of view (FOV) and remains less than 2.0 mm within 5 cm of the center of the FOV. Imaging of the myocardium in mice and rats with the flow tracer 13N-ammonia or the glucose analogue 18F-deoxyglucose typically requires activity doses of 0.5 to 1.5 mCi.15 Count rates at these radioactivity doses remain relatively low so that dead-time losses are small. Image reconstruction with the more recently developed maximum a posteriori probability (MAP) algorithm, now employed for cardiac studies in mice, provides images of myocardial perfusion and glucose uptake of good to excellent diagnostic quality (see Fig. 11-2).16 The MAP reconstruction algorithm improves the spatial resolution in the center of the FOV to 1.2 mm FWHM so that a volumetric resolution of less than 5 microliters is now possible.
The emission data are reconstructed initially into a set of contiguous transaxial slices with a 0.75-mm interplane spacing (Fig. 11-4).17 The transaxial images are typically reoriented into short-axis and vertical and horizontal long-axis cuts (Fig. 11-5)17 that can be assembled into polar maps analogous to those of myocardial perfusion studies in humans (Fig. 11-6).17 Images of glucose uptake obtained with 18F-deoxyglucose are of greater contrast and higher quality than images of perfusion obtained with 13N-ammonia. This is most likely because of the more complete clearance of 18F-deoxyglucose from blood and higher tracer activity concentrations in the myocardium. Another reason is the slower physical decay of 18F-deoxyglucose so that over the total image acquisition time, more counts are recorded. On the other hand, the shorter physical half-life of 13N-ammonia affords the ability to perform repeat examinations of myocardial blood flow during the same study session so that effects of interventions can be evaluated. ECG gated image acquisition is also possible with small PET systems so that left ventricular function can be assessed.18 For example, in normal 300-g rats, the left ventricular ejection fraction was about 60% to 70%, and the stroke volume was about 300 μL.18
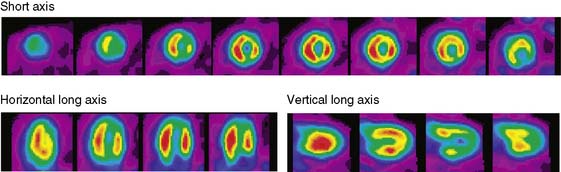
Figure 11-5 Reoriented images of the myocardial 18F-deoxyglucose uptake in the same mouse as depicted in Figure 11-4. Selected short-axis (upper row) and vertical and horizontal long-axis cuts are shown.
(Reproduced with permission from Schelbert HR, Inubushi M, Ross RS: PET imaging in small animals. J Nucl Cardiol 10:513–520, 2003.)
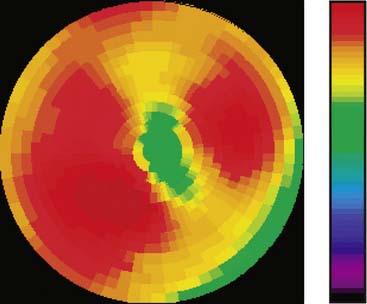
Figure 11-6 Polar map display of the 18F-deoxyglucose uptake in the left ventricular myocardium in the mouse depicted in Figures 11-4 and 11-5.
(Reproduced with permission from Schelbert HR, Inubushi M, Ross RS: PET imaging in small animals. J Nucl Cardiol 10:513–520, 2003.)
The image reconstruction algorithm corrects for radioactive decay and for dead-time losses. It also includes routines for photon attenuation correction. These routines utilize transmission images that are typically acquired prior to radiotracer administration and are performed using a Co57 point source or, for hybrid microPET/CT systems, an x-ray attenuation map. Despite the small body size of rats, photon attenuation causes about 30% systematic underestimation of the true myocardial radioactivity concentration.19 Albeit somewhat less in mice, photon attenuation still amounts to about 17% underestimation of the true activity. Accordingly, even in small animals, proper attenuation correction is important for the measurement of the true absolute intensities in cardiac imaging. Chow et al.19 have shown that CT-based attenuation correction methods result in less noise correction to emission images compared to the transmission-based attenuation corrections.
The stationary imaging gantry of microPET permits rapid serial (or “dynamic”) acquisition of images. Theoretically, images can be recorded at sampling rates of less than 1 second. For practical purposes, however, image acquisition rates depend on the number of counts required for statistically adequate image frames. Framing rates in the range of 2 to 10 seconds per image following an intravenous bolus of 13N-ammonia, 18F-deoxyglucose, or 11C-palmitate produce statistically adequate images for generating tissue time-activity curves and thus are appropriate for radiotracer kinetic studies.20 Figure 11-7 depicts selected frames acquired during and shortly after an intravenous bolus injection of 13N-ammonia in a rat. High temporal-resolution tissue time-activity curves for the right and left ventricular blood pools and the left ventricular myocardium in a rat following an intravenous bolus of 18F-deoxyglucose into a tail vein are shown in Figure 11-8.
IMAGING THE CARDIOVASCULAR SYSTEM IN MICE AND RATS
Experimental Coronary Occlusions in the Rat
The extent and severity of regional myocardial perfusion defects that are experimentally induced by transient coronary occlusions in rats can be accurately determined with microPET.15 In Figure 11-9, myocardial perfusion was imaged with intravenous 13N-ammonia at baseline, 45 minutes later during a 20-minute coronary occlusion, and again 45 minutes following reperfusion. Figure 11-10 shows the corresponding polar map displays. The location and extent of the image perfusion defects corresponded well with the actual regions observed on postmortem stained tissue. Using a threshold of 50% of the maximum myocardial activity concentration as the lower limit of normal, the size of the flow defect derived from polar maps of myocardial perfusion correlated linearly with the defect sizes determined by postmortem examination (Fig. 11-11).
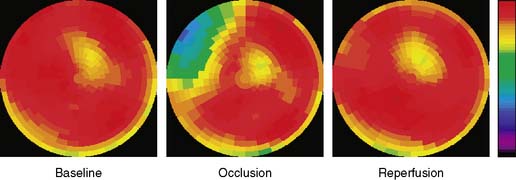
Figure 11-10 Polar map display of the distribution of myocardial blood flow recorded serially before, during, and following a transient coronary occlusion in the rat depicted in Figure 11-7. Note the perfusion defect on the polar map during the occlusion, as indicated in blue.
(Reproduced with permission from Kudo T, Fukuchi K, Annala AJ, et al: Noninvasive measurement of myocardial activity concentrations and perfusion defect sizes in rats with a new small-animal positron emission tomograph, Circulation 106:118–123, 2002.)
Studies in Genetically Modified Mice
Initial studies have demonstrated the possibility of phenotyping genetically modified mice. For example, cardiac myocyte-specific excision of the murine β-1 integrin gene results in extensive myocardial fibrosis.21 In adulthood, the mice develop a form of dilated cardiomyopathy and symptoms of congestive heart failure. MicroPET imaging with 18F-deoxyglucose in β-1-integrin knockout mice revealed heterogeneously distributed tracer uptake consistent with either replacement of myocytes by fibrotic tissue or as a consequence of regional alterations in substrate metabolism (Fig. 11-12). Assessment of myocardial perfusion with 13N-ammonia in the β-1-integrin knockout mouse further revealed heterogeneously distributed myocardial blood flow in addition to patchy regions of reduced tracer uptake.
Development of Novel Radiotracer Assay Approaches
Noninvasive, image-based probe systems for studying location, extent, magnitude, and duration of gene expression have also been explored and are now being used in small animals. One approach used in oncologic studies in mice has been the PET reporter gene/reporter probe system.9–11,22 In one of these systems, a mutant herpes simplex virus thymidine kinase gene is delivered by an adenoviral vector and represents the PET reporter gene. If expressed in tissue, thymidine kinase phosphorylates the radiolabeled 9-(4-[18F]-fluoro-3 hydroxymethylbutyl) (guanine) (18FHBG) that serves as the PET reporter probe. Because the phosphorylated product of 18FHBG is relatively impermeable to the cell membrane, it becomes effectively trapped in tissue and thus can be imaged with PET. Details on this in vivo assay approach and on other probe systems are described in several recent reviews.6,9,10,23
Pilot studies with in vitro imaging approaches had explored the feasibility of adapting these probe systems to the heart.24
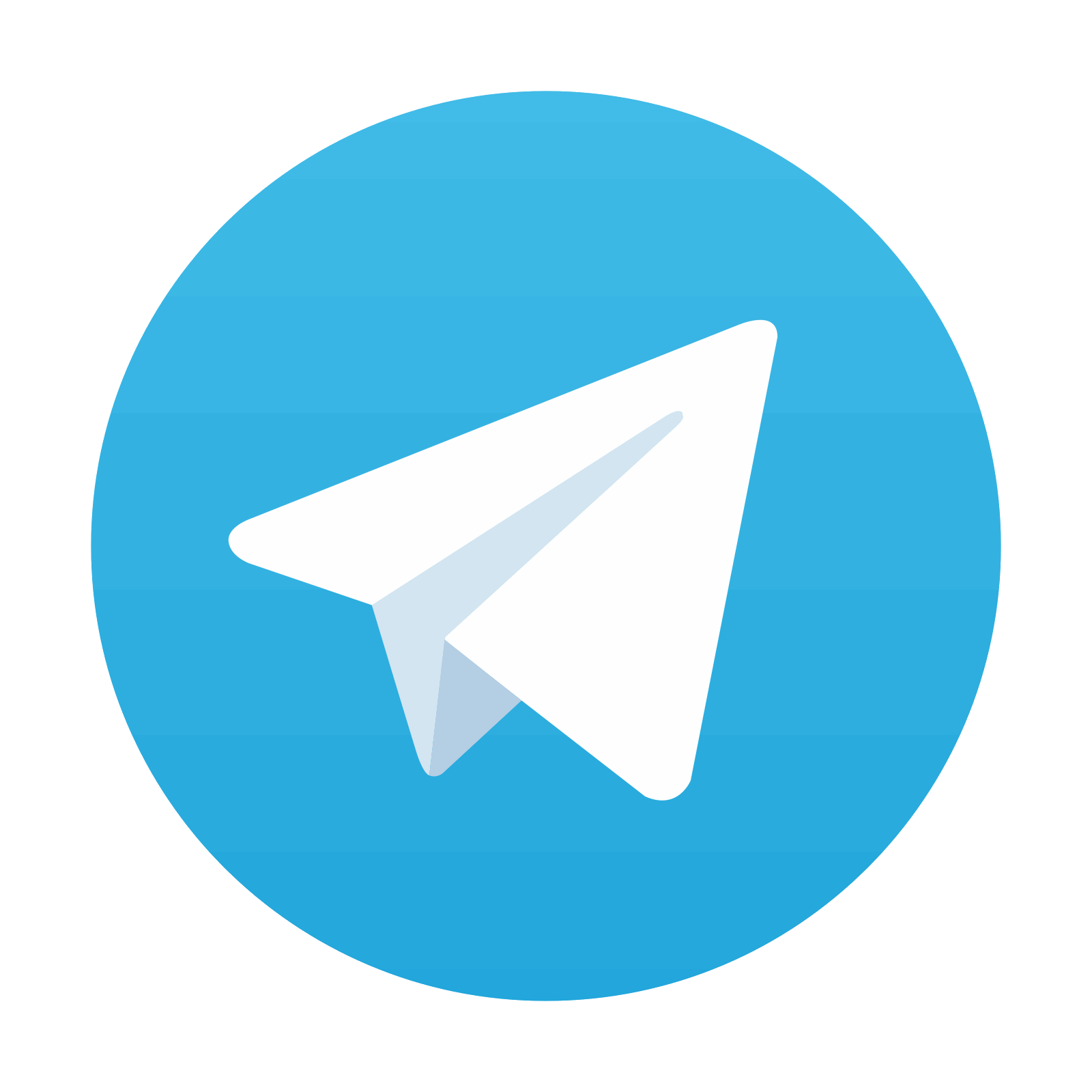
Stay updated, free articles. Join our Telegram channel

Full access? Get Clinical Tree
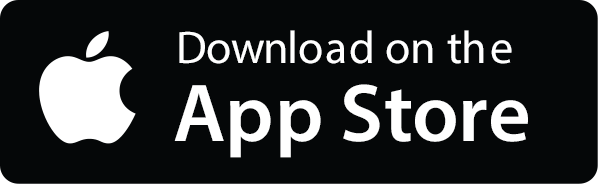
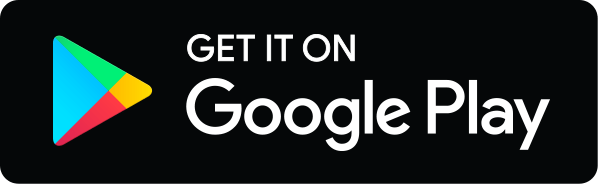