© Springer International Publishing Switzerland 2017
Ali Dabbagh, Antonio Hernandez Conte and Lorraine Lubin (eds.)Congenital Heart Disease in Pediatric and Adult Patients10.1007/978-3-319-44691-2_2121. Right-Sided Obstructive Lesions
(1)
Cedars Sinai Medical Center, Department of Anesthesiology, 8700 Beverly Blvd, Rm 4209, West Hollywood, CA 90048, USA
(2)
Cedars Sinai Medical Center, Department of Anesthesiology, Los Angeles, CA 90048, USA
(3)
Congenital Cardiac Anesthesiology, Operative Transesophageal Echocardiography, Cedars Sinai Medical Center, Department of Anesthesiology, 8700 Beverly Blvd, Suite 8211, Los Angeles, CA 90048, USA
Tetralogy of Fallot
Introduction
Tetralogy of Fallot (TOF) is one of the most common cyanotic cardiac defects, accounting for about 3–5 % of all congenital cardiac disease, with an estimated incidence of 0.2–0.8 per 100 live births (Apitz et al. 2009). Niels Stensen first described it in 1671 with its physiology refined later by Etienne-Louis Fallot in 1888. In 1924, Maude Abbott coined the term tetralogy of Fallot based on the four classic lesions that make up the disease
- 1.
Ventricular septal defect (VSD)
- 2.
Right ventricular outflow tract (RVOT) obstruction
- 3.
Overriding aorta
- 4.
Right ventricular hypertrophy (Stensen 1671–1672)
Today we know that TOF is a family of disease with similar intracardiac anatomy that can be highly variable in terms of pulmonary artery, RVOT, and pulmonary valve anatomy. Due to its involvement with pulmonary artery and valve and the RVOT, it is discussed here in brief; a full discussion is presented in Chap. 19, Tetralogy of Fallot.
TOF is also commonly associated with other cardiac anomalies such as anomalous coronary arteries, right aortic arch, and atrioventricular valve defects.
Although the exact cause of the disease is still unknown, recent studies have shown certain genetic predisposition to the disease. In one study of patients with TOF, 25 % were found to have microdeletion of q11 region in chromosome 22. TOF is also commonly found in patients with 22q11 deletions such as in Di George syndrome and velocardiofacial syndrome. In those without any overt syndromes, the prevalence of deletions has been estimated at 6 % (Apitz et al. 2009).
Anatomy
The physiology of TOF is largely dependent on the severity of the right outflow tract obstruction. The ventricular defect found in TOF is usually large with unrestricted flow between the two ventricular chambers. As a result of this, right ventricular pressure is reflective of left ventricular pressure, and blood flow across the septal defect follows the path of least resistance. If the right outflow tract obstruction is higher than the resistance of blood going through the aorta, then blood flow will preferentially shunt right to left resulting in decreased pulmonary blood flow and desaturated blood entering the systemic circulation resulting in cyanosis. If left ventricular pressure is higher than right ventricular pressure, then blood flow will preferentially shunt left to right with saturated blood diverted away from the systemic circulation and into the pulmonary vasculature resulting in decreased cardiac output.
Presentation
Clinical presentation can vary depending on severity of anatomical right outflow tract obstruction and degree of shunting across the septal defect. Newborn with severe right outflow obstruction will present with cyanosis secondary to decreased pulmonary blood flow and increased mixing of desaturated blood. Those with moderate to mild right outflow obstruction are usually asymptomatic unless agitated in which cyanosis can ensue. Many with mild obstruction are diagnosed incidentally with auscultation of a harsh systolic murmur on exam. Those with mild obstruction can have pulmonary overcirculation and develop heart failure. Although most TOF are symptomatic and cyanotic to a certain degree, there are some who remain well saturated without any cyanosis for a period of time (pink tets).
Majority are asymptomatic when comfortable and at rest. However agitation and crying can worsen the right outflow tract obstruction resulting in cyanosis best seen in the lips and nail beds. As mentioned before a systolic murmur is usually present from the right outflow tract obstruction. Classically, it is described as a crescendo-decrescendo murmur heard best at the left mid-upper sternal border. As RVOT obstruction increases, so does the flow across the septal defect and the turbulent flow through the RVOT and the severity of the murmur. During severe “tet spells” when all blood is diverted across the septal defect, the systolic murmur may become absent.
Physical exam along with EKG and chest X-ray can be used for diagnosis, but echocardiography is used to confirm the disease. EKG will usually demonstrate signs of right axis deviation from increased right ventricular pressure, while chest X-ray may demonstrate the classic boot-shaped heart secondary to right ventricular hypertrophy. Using 2-D echocardiography, multiple components of TOF can be evaluated for preparation of surgical correction. The location and number of VSD should be noted. Both the degree of aortic override and extent of the septal defect can be evaluated via the parasternal and apical view. As mentioned before, the degree of right outflow tract obstruction can vary with location and severity and must be delineated prior to surgical correction. The parasternal short axis and subcostal coronal and sagittal views are best used to evaluate the pulmonary valve and right outflow tract. The pulmonary annulus should be assessed to determine if a transannular patch is needed during surgical correction. The pulmonary artery along with its branches should also be evaluated in the parasternal short axis, looking at size and anatomy. The coronary arteries should also be assessed with the parasternal short axis and long axis view looking for aberrant anatomy, specifically where the left anterior descending coronary originates from. This is important because anterior crossing coronary vessels may complicate surgical repair of right outflow tract obstruction. The aorta should also be assessed using the parasternal notch short and long axis view looking for anatomy and potential aortopulmonary collaterals and a PDA. Echocardiography can also be used to assess the degree of outflow tract obstruction by assessing blood flow via color Doppler and gradient calculation using the modified Bernoulli equation.
Treatment
Surgical repair of TOF usually occurs within the first year of life, usually before 6 months of age. It can be staged if the infant is too small to tolerate cardiopulmonary bypass, and time is needed for the infant to grow or completely repaired shortly after birth. Early repair for appropriate infants is currently the preferred route given the excellent outcomes. Studies have shown that minimizing right ventricular exposure to high pressure prevents irreversible remodeling of the right ventricle, specifically right ventricular hypertrophy and right ventricular failure (Al Habib et al. 2010). Goals of surgical repair are to relive the RVOT obstruction and separate the systemic circulation from the pulmonary circulation by closing off the VSD.
Most full infants undergo complete repair shortly after birth via an intra-atrial or intraventricular approach. Most prefer the intra-atrial approach so as to minimize scarring and injury to the ventricle, which can lead to impaired function and conduction abnormities postoperatively. Using either approach, the surgeon can relieve pulmonary stenosis, resect infundibular and subinfundibular muscle bundles, or create a transannular patch to open up the RVOT obstruction and patch up the VSD (Morales et al. 2009). In addition to minimizing damage to the ventricle, many surgeons attempt to maintain pulmonary valve competency by using a “valve sparring approach” whenever possible. This technique is applied to individuals with adequate pulmonary valve annulus size, as they do not need a transannular patch, unlike individuals with small or borderline annulus who usually require a transannular patch (Airan et al. 2006; Karamlou et al. 2006). Although a transannular patch opens up the RVOT obstruction, it also makes the pulmonary valve incompetent resulting in pulmonary insufficiency.
An alternative surgical approach is to create a valve conduit between the RV and the PA. Valve incompetency, regurgitation, and conduit stenosis are all complications that can occur overtime with a conduit.
Small and premature infants usually require a staged approach to correction due to high mortality associated with going on bypass. As a result they usually undergo a central shunt or BT shunt placed temporarily to provide an adequate source of pulmonary blood flow while they grow to an adequate size to tolerate a complete intracardiac repair on bypass. Once the child is of adequate size, the palliative pulmonary shunt is taken down, and the right outflow tract obstruction opened to allow for adequate pulmonary blood flow. At the same time, the VSD is closed off so to avoid shunting at the ventricular level.
Anesthetic Considerations
Anesthetic management of individuals with TOF should be to promote forward blood flow from the RV to the lungs and limiting right to left shunting of deoxygenated blood through the VSD. As mentioned before, the pathophysiology of TOF is decreased antegrade flow to the lungs secondary to the RVOT obstruction with shunting across the VSD resulting in cyanosis.
Depending on the severity of the obstruction, individuals may require supplemental oxygen therapy. Agitation to the infant should be avoided when possible as crying can increase pulmonary pressures and further decrease pulmonary perfusion and increase the amount of deoxygenated blood shunted systemically through the VSD. Premedication with oral midazolam (0.5 mg/kg) prior to inhalation induction can minimize the amount of stress and agitation and thus minimize “tet spells” that may result in severe cyanosis. Ketamine (1–4 mg/kg) and fentanyl can be used for induction if intravenous access is available. Adequate ventilation and oxygenation is important in these individuals as hypoxemia and hypercarbia can both increase pulmonary vascular resistance and result in overall increased right-sided pressures and obstruction. Tet spells with severe cyanosis benefit from Neo-Synephrine (5–10 mcg/kg) to increase the SVR. The alpha1 agonist results in direct vasoconstriction with increased left ventricle pressures, reversing the right to left shunt through the VSD and limiting deoxygenated blood into the systemic system. Another benefit of Neo-Synephrine is that it has no inotropic effects on the heart, which is beneficial as increased cardiac contractility and tachycardia can further impede pulmonary blood flow if the RVOT obstruction is dynamic such as in infundibular spasm. Increasing the depth of anesthesia with volatile agents can also be used for negative inotropic effects. Although this may help with infundibular spasm, it can also result in vasodilatation and drop in SVR, which can worsen shunting through the VSD. Volume infusion and maintaining adequate preload will help by increasing right heart filling pressure and increase overall blood pressure.
Arterial line should be placed opposite side of planned BT shunt if a stage procedure is being done, as the shunt can cause a steal effect resulting in an artificially lower blood pressure reading. Central line placement should be considered for volume replacement and vasoactive drug infusions post bypass.
Postoperatively, it is not uncommon to see decreased RV function or even RV failure as their RVs have been exposed to high pressures. This, coupled with injury to the ventricles during surgical repair, can result in less than optimal RV function immediately after surgery and may require vasoactive drugs such as epinephrine and milrinone (0.25–05 mcg/kg/min) for hemodynamic support. Abnormal conduction is also a common occurrence postoperatively given the close proximity of the conduction pathway to the VSD repair. As a result, atrioventricular pacing capability should be available to maintain AV synchrony, which will help with the RV function and cardiac output.
Congenital Pulmonic Valve Stenosis
Introduction
The incidence of congenital pulmonic stenosis is approximately 0.6–0.8 per 1000 live births and accounts for about 10 % of all congenital cardiac disease (Hoffman and Kaplan 2002). Pulmonic stenosis is defined as right ventricular tract obstruction at the level of the pulmonary valve. Where along the pulmonary valve, the obstruction occurs can vary.
Anatomy
Classically, the stenosis is at the valve with fusion of the valvular tissue around a fixed orifice resulting in a dome-shaped deformity. Koretzyk described a subset of pulmonary valve dysplasia where instead of the classic fused valves, the valves are not adherent to each other. Instead, obstruction occurs from (1) thickened leaflets rendering them rigid and immobile and (2) occurrence of tissue in the sinuses that restrict lateral movement of leaflets during systole. It is important to delineate between the two, as pulmonary valve dysplasia is associated with higher failure rates with balloon valvuloplasty compared to pulmonary valve stenosis and is commonly seen in Noonan’s syndrome (Koretzky et al. 1969). Less common is subvalvular obstruction from fibromuscular narrowing below the pulmonary valve, which is associated with other congenital cardiac lesions such as TOF. Subvalvular lesions can be dynamic where increased contractility of the ventricle causes increase obstruction. Supravalvular stenosis can also occur with narrowing of the pulmonary artery above the pulmonary valve.
The underlying cause of pulmonic stenosis is unknown. During the fifth week of gestation, the conotruncus (bulbus cordis) divides into the ascending aorta and the main pulmonary artery. The pulmonary valve develops from the distal conotruncus, moving anterior and leftward of the aortic valve. Some have hypothesized that pulmonary stenosis occurs from maldevelopment of the distal conotruncus.
Presentation
Clinical presentation of congenital pulmonic stenosis can vary depending on the degree of stenosis. Those with trivial to mild pulmonary stenosis (gradient <40) are usually asymptomatic besides having a pulmonary ejection murmur at the second intercostal space. Multiple studies have shown those with mild pulmonic stenosis will usually have no progression of their disease and is generally considered benign (Drossner and Mahle 2008; Rowland et al. 1997).
Moderate pulmonary valve stenosis (gradient btw 40–60) can present with poor weight gain secondary to dyspnea and fatigue with feeding. Disease progression is somewhat variable with studies showing RVOT obstruction, decreased cardiac output, and increased right ventricular end-diastolic pressures later on in life (Hayes et al. 1993).
Those with severe stenosis (gradient >60) at birth can present with cyanosis from atrial shunting, heart failure from increased right ventricular end-diastolic pressure, and right ventricular hypertrophy and can lead to irreversible right ventricular dysfunction if not treated promptly (Krabill et al. 1985; Johnson 1962; Stone et al. 1974).
Critical pulmonic stenosis is the most severe with inadequate pulmonary blood flow through the RVOT. Because of this, it is paramount that the ductus arteriosus remains patent for pulmonary blood flow. Presentation is similar to severe pulmonic stenosis and if not treated in a timely manner may become life threatening (Freed et al. 1973).
Depending on the severity of the stenosis, patients may have enlarged right atrium and right ventricular hypertrophy manifesting radiographically as an enlarged cardiac silhouette and with right axis deviation on EKG.
Echocardiography is recommended to confirm the diagnosis of pulmonic stenosis given the relative ease at which the pulmonary valve and right heart can be visualized through transthoracic echocardiogram. Besides visualizing the amount of blood flow across the pulmonic valve, continuous wave Doppler can be used to estimate the gradient across the valve. A transvalvular gradient of <40 mmHg is considered mild stenosis, 40–60 mmHg moderate stenosis, and >60 mmHg severe stenosis.
Treatment
Balloon valvuloplasty is the first-line treatment for pulmonic stenosis confined to the valvular level. Those with critical pulmonic stenosis may require balloon valvuloplasty shortly after birth, whereas those with mild to moderate stenosis may have the valvuloplasty done electively at a later date with observation first. Balloon valvuloplasty is done through percutaneous access via the femoral vein, where a wire is advanced through the pulmonary valve. Once in position, a balloon is placed through the pulmonic valve and inflated to dilate the stenotic valve. It is not uncommon to see pulmonary regurgitation post balloon therapy. The amount of regurgitation can be limited by optimizing balloon size (Rao 2007). Right ventricular enlargement can develop overtime from the regurgitant flow. Currently there is no consensus regarding the timing of pulmonary valve replacement in those with severe pulmonary regurgitation post balloon valvuloplasty.
Those with typical pulmonic stenosis have excellent outcomes with balloon valvuloplasty with majority having gradients less than 20 mmHg post intervention. Potential complications with balloon valvuloplasty include perforation of the pulmonary valve, right ventricle, pulmonary artery, and damage to tricuspid valve resulting in regurgitant flow (Rao 2007; Stanger et al. 1990). Balloon valvuloplasty can also be used to treat dysplastic pulmonary valves, although outcomes are less favorable when compared to dome-shaped pulmonic stenosis and surgical correction may be required (Stanger et al. 1990; Tabatabaei et al. 1996).
Surgical repair is usually needed for dysplastic, supravalvular, and subvalvular pulmonary stenosis. With dysplastic pulmonary valve, surgical excision of the thickened leaflets and sometimes a transannular patch are needed to relive the obstructive lesion. Surgical correction is also recommended for supravalvular lesions due to the close proximity to the pulmonary artery, and most will require a transannular patch. Subvalvular lesions usually have a muscular component to the obstruction making balloon valvuloplasty an ineffective treatment option.
Anesthetic Considerations
The anesthetic goal for individuals with pulmonic stenosis is to promote forward blood flow from the RV to the pulmonary artery and to ensure adequate pulmonary perfusion. Individuals with severe stenosis and cyanosis may require supplemental oxygenation and prostaglandin therapy to ensure adequate oxygenation. For surgical repairs, arterial line should be placed for close blood pressure monitoring. Central access should be obtained for volume replacement and vasoactive drug infusion. Depending on the severity of the disease and preexisting RV function, inotropic support may be needed immediately after the surgical repair. Those with severe pulmonic stenosis may have dysfunctional RV and require inotropic agents such as milrinone (0.25–0.5 mcg/kg/min) and dobutamine (1–5 mcg/kg/min) to maintain cardiac output. Inotropic support should be used cautiously for individuals with subvalvular stenosis, as there is usually a dynamic component to the stenosis and the increased contractility and tachycardia can worsen the obstruction. Volume infusion and adequate preload can help with RV filling pressure and promote forward flow from the RV. However careful titration of volume should be considered for those with borderline RV function as too much preload can add additional stress to the ventricle and result in worsening function and decrease cardiac output. Adequate ventilation strategy is also important as hypercarbia and hypoxemia can both increase PVR and add additional strain to the already dysfunctional RV.
Pulmonary Atresia with Intact Ventricular Septum
Introduction
In pulmonary atresia with intact ventricular septum (PA/IVS), there is atresia of the pulmonary valve resulting in absent connection between the right ventricular outflow tract and the pulmonary artery. In addition, there is no communication between the two ventricles because of the intact septum and thus no way of mixing, making this a fatal structural cardiac defect unless surgically corrected.
PA/IVS accounts for approximately 3 % of all congenital cardiac defects with an estimated incidence of 4–8 per 100,000 live births (Hanley et al. 1993; Ekman Joelsson et al. 2001; Ashburn et al. 2004).Unlike pulmonary atresia with VSD, the cause of the PA/IVS is currently not known. There does not appear to be any associated genetic syndrome associated with the defect. There are hypotheses that it is an acquired defect in utero secondary to viral or inflammatory disease causing pulmonary valve atresia and abnormal fetal blood flow ultimately leading to PA/IVS (Kutsche and Van Mierop 1983).
Because of the wide range of anatomical defects found in PA/IVS, there is now a uniform reporting system defining PA/IVS as a congenital malformation with right ventricular outflow obstruction that is ductal dependent and can include pulmonary atresia, variable degree of tricuspid and right ventricular hypoplasia, and aberrant coronary anatomy (Lacour-Gayet 2000).
Anatomy
The pulmonary atresia in PA/IVS can be either muscular or membranous with majority being membranous. In membranous pulmonary atresia, the pulmonary valve annulus is small with fused leaflets resulting in RVOT obstruction. The right ventricle and infundibulum are usually well formed in membranous pulmonary atresia. Muscular pulmonary atresia occurs in about 25 % of PA/IVS and is associated with poor outcome secondary to severe right ventricular hypoplasia and anomalous coronary arteries (Dyamenahalli et al. 2004; Daubeney et al. 2002; Kipps et al. 2011). In muscular pulmonary atresia, the muscular infundibulum is obliterated resulting in complete obstruction of the RVOT.
The anatomy of the right ventricle in PA/IVS can vary widely from a dilated thin-walled ventricle to a severely hypoplastic hypertrophied ventricle. Normally the RV is divided into three parts or tripartite, the (1) inlet, (2) body, and (3) outlet. Those with a tripartite RV will allow for a biventricular repair, whereas bipartite or unipartite, with absent body and/or outlet, will need univentricular repair due to the inability to support full pulmonary blood flow (Dyamenahalli et al. 2004; Daubeney et al. 2002).
The tricuspid valve (TV) can also vary from small to dysplastic with stenotic or regurgitant flow. The size of the tricuspid valve has been found to correlate with the anatomy of the RV. A TV with a Z score of −4 will usually have a unipartite RV making biventricular repair unlikely, whereas a TV Z score of −2 to 0 is associated with a tripartite ventricle, making biventricular repair possible (Ashburn et al. 2004).
Coronary anatomy can also vary in PA/IVS from absent aortocoronary connection to stenosis and abnormal left to right coronary connections. Normally coronary perfusion is dependent on diastolic pressure and diastolic flow. However in PA/IVS, fistulae can form between the RV and coronary arteries. This combined with absent aortocoronary connections makes coronary perfusion dependent on retrograde flow from the RV during systole or right ventricle-dependent coronary circulation (RVDCC). Recognition of RVDCC is important to note as these patients are dependent on elevated RV pressures providing coronary perfusion via the fistulae between the RV and coronaries, and relieving the RVOT obstruction will decompress the right ventricle resulting in inadequate coronary perfusion, ischemia, infarction, and sudden cardiac death (Calder et al. 2007; Giglia et al. 1992). Because of this, it is important to delineate the anatomy of the RV, TV, and coronary circulation, as all three play a role in determining if the child will have a biventricular, 1½, or univentricular surgical repair.
Unlike PA/VSD, PA/IVS usually has normal anatomy distal to the pulmonary valve. Majority will have normal confluent branches coming off the main pulmonary artery with a left ductus supplying pulmonary blood flow. In some there are abnormal pulmonary artery with arterial connections between the aorta and pulmonary artery called major aortopulmonary collaterals (MAPCA).
In PA/IVS, there is RVOT obstruction from the pulmonary atresia and no blood flow from the RVOT to the pulmonary artery. Instead pulmonary blood flow depends on regurgitant flow into the right atrium where it can cross over to the left atrium via a patent foramen ovale and supply blood to the lungs via the ductus arteriosus. Because of this, all newborns with PA/IVS must have a patent ductus arteriosus in order to survive, as that is the only means of pulmonary blood flow. Those with PA/IVS will usually have a smaller PDA compared to normal infants due to decreased pulmonary blood flow through the ductus in utero. RV pressures in these patients are determined by the extent of RV egress. Those with severe tricuspid regurgitation may have normal RV pressures as blood flows freely back into the right atrium and is shunted to the left atrium via the patent foramen ovale. However in those with limited tricuspid regurgitation, RV pressure can become suprasystemic as the blood cannot move forward or backward. If RV sinusoids exist, blood can flow through these channels into the systemic circulation via the coronary circulation.
Presentation
Infants with PA/IVS will usually present with cyanosis secondary to right to left shunting at the atrial level and complete RVOT obstruction. Most neonates with PA/IVS will have normal fetal and birth history with tachypnea and hyperpnea during the newborn period. However if untreated, PA/IVS is ultimately fatal with approximately 50 % dying within 2 weeks and 85 % dying within 6 months (Leonard et al. 2000). As mentioned before, those with PA/IVS are dependent on a PDA for pulmonary blood supply and survival. Closure of the ductus arteriosus will lead to rapid deterioration including acidosis, hypoxia, cardiogenic shock, and ultimately death. There have been rare reports of PA/IVS surviving on aortopulmonary collaterals once the ductus arteritis closes off (McArthur et al. 1971). Depending on the anatomy, a variety of murmurs can be appreciated on auscultation. Those with severe tricuspid regurgitation will have a systolic murmur, while a continuous murmur might be heard secondary to the PDA. Similarly, chest X-ray in those with PA/IVS can be normal or have an enlarged cardiac silhouette if they have severe tricuspid regurgitation resulting in enlarged right atrium and ventricle. EKG will usually show left axis deviation secondary to absent or hypoplastic RV.
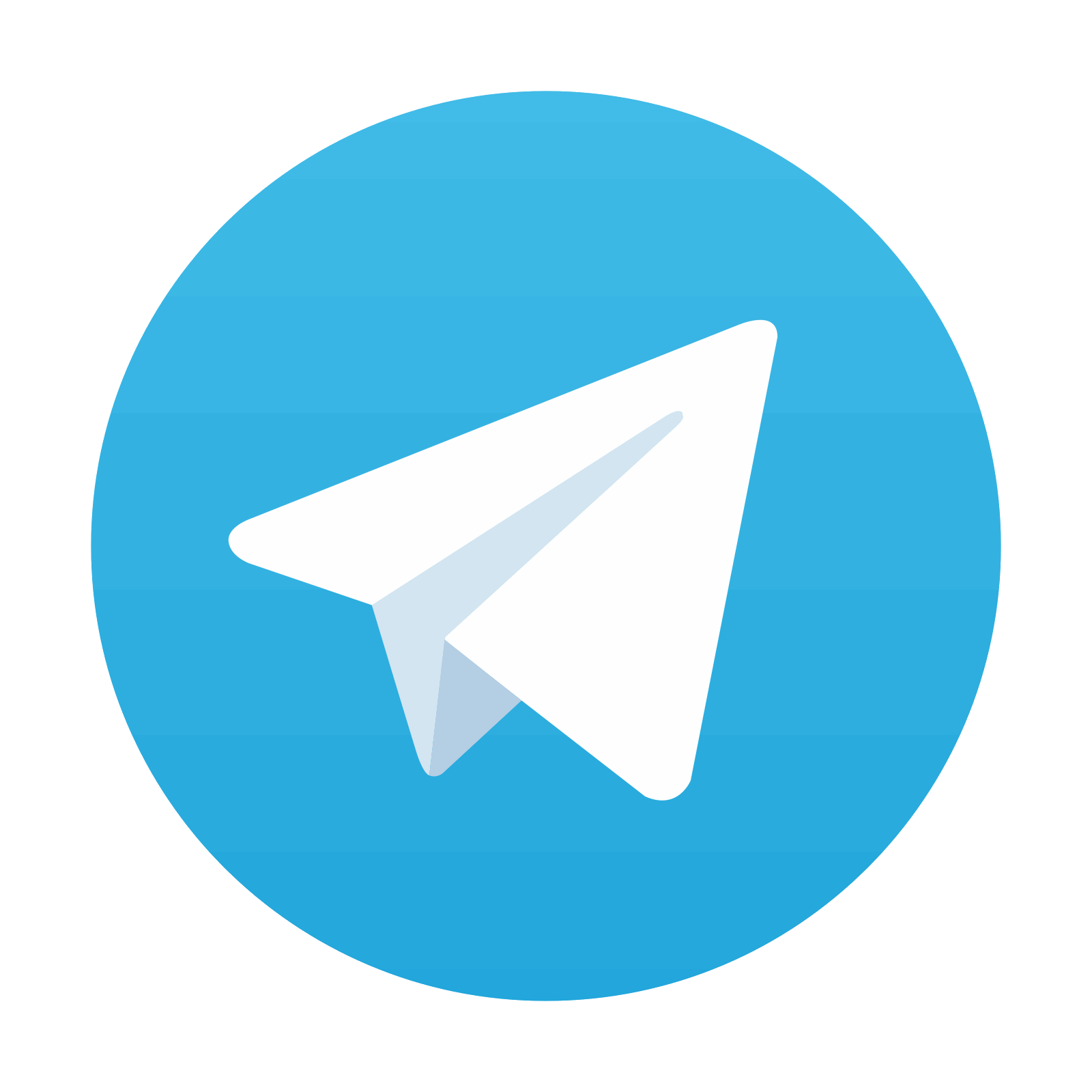
Stay updated, free articles. Join our Telegram channel

Full access? Get Clinical Tree
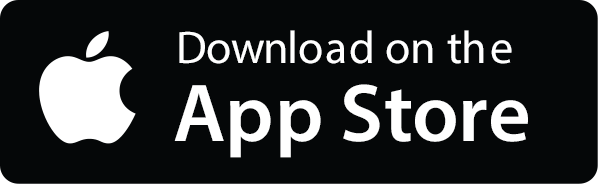
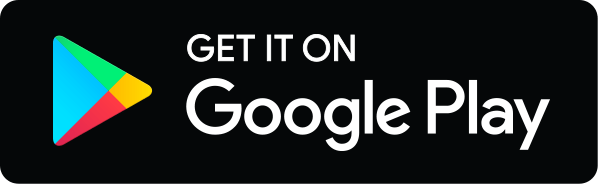