Fig. 15.1
(a) Transcatheter PDA closure in the NICU using rainbow flex procedural bed and micro-fluoroscopy unit (at white arrow) (authors). (b) Large PDA (*) with hemodynamically important left-to-right shunt in a 59-day-old 1200-g, former 27-week premature infant. The PDA (at star, descending aorta (at triangle)) and left pulmonary artery (white arrow) are demonstrated (authors). (c) Angiographic image of the ductal device still attached to the delivery cable. Note the transthoracic echocardiogram (used to obtain images continuously throughout the procedure) probe at the upper left corner of the photo (authors). (d) The PDA device (at white arrow) in place with no residual ductal shunting an color image (authors). (e) There is no gradient in the descending aorta after device placement (authors). (f) The left pulmonary artery (at white arrow) is unobstructed by the device (authors). (g) Spectral Doppler interrogation with the device in situ (authors)
Transcatheter Valves
Many patients who have undergone repair of tetralogy of Fallot or pulmonary atresia will require pulmonary valve replacement at least once during their lifetime, for residual regurgitation and/or stenosis. Surgical pulmonary valve replacement is associated with significant pain and common complications such as pleural effusion, pericardial effusion, and arrhythmias and has a mortality of 0.5 % (Fuller et al. 2015). It is no surprise that transcatheter pulmonary valve replacement for those with appropriately configured right ventricular outflow tracts has become first-line therapy. The Melody valve (Medtronic, Minneapolis, MN) (Cheatham et al. 2015) is a bovine jugular valve that was the first transcatheter pulmonary valve placed in man (Bonhoeffer et al. 2000). It is ideally suited to the patient with a right ventricular to pulmonary artery conduit as it has a maximum inflated diameter of 24 mm. Unfortunately, the majority of patients requiring pulmonary valve replacement have a reconstructed “native” pulmonary valve that is regurgitant from initial surgical valvotomy and have diameters much larger than the capacity of the Melody valve. The Edwards SAPIEN valve (Edwards Lifesciences, Irvine, CA), a bovine pericardial stent-mounted transcatheter valve, has been successfully used for this indication in selected patients with appropriately sized right ventricular outflow tracts for this indication (Wilson et al. 2015; Phillips et al. 2016) as it is manufactured in sizes up to 29 mm. The internal diameter of the larger SAPIEN valve also increases the likelihood that multiple repeat transcatheter valve-in-valve procedures can be performed when these valves require future replacement. Sizing of the outflow tract to select appropriate patients and appropriate valves requires careful assessment. Some centers are using 3D modeling of the right ventricular outflow tract using magnetic resonance imaging (MRI) (Phillips et al. 2016) to augment balloon sizing (see Fig. 15.2). Compliance of the native RVOT must also be tested with a balloon before valve placement. High compliance can be a contraindication to use of this technique, with risk of distal valve embolization (Phillips et al. 2016). Native RVOT pulmonary valve implantation can also be performed by hybrid technique (using a 2-inch incision at the bottom of the old sternotomy) (see Fig. 15.2) to enter the right ventricle via a periventricular approach with the delivery catheter or by surgically plicating the pulmonary artery to a size amenable to use of this valve (Travelli et al. 2014). The latter technique requires a sternotomy but not the use of cardiopulmonary bypass. For individuals with a large, irregularly shaped RVOT, internal geometric remodeling using covered stents plugged with Amplatzer vascular plug II device(s) (St. Jude Medical, St. Paul, MN) to reduce annular size can be performed (Phillips et al. 2016). Hospital discharge generally occurs at 24 h for transcatheter approaches (O’Byrne et al. 2015) and at 48 h for hybrid approaches (Phillips et al. 2016) in comparison to an average 4- to 5-day hospital stay for surgical pulmonary valve replacement (Vergales et al. 2013; McKenzie et al. 2014; O’Byrne et al. 2015).


Fig. 15.2
(a) 3D model of a right ventricular outflow tract with an Edwards SAPIEN valve in situ with geometric remodeling of the annular area with plugged stent around the valve (authors). (b) Hybrid pulmonary valve incision for periventricular placement of the valve (authors). (c) Hybrid pulmonary valve incision with valve delivery catheter in situ (authors)
Electrophysiologic Devices
A number of minimally invasive and alternative electrophysiologic devices have become available that may benefit selected CHD patients. Subcutaneous leadless implantable cardioverter-defibrillators (ICD) (Emblem S-ICD, Boston Scientific, Marlborough, MA; see Fig. 15.3) can be useful for adult patients with poor venous access to the heart. The extracardiac Fontan patient who requires only defibrillation and not pacing capacity is a perfect candidate for this device (Bordachar et al. 2016). Recent studies (Lambiase et al. 2014; Burke et al. 2015) have demonstrated the safety and efficacy of the subcutaneous ICD. The device requires no fluoroscopy for insertion (Bardy et al. 2010). At present, the size of the device limits the implantation to older children and adults. In addition, there are additional screening requirements that must be met (Bordachar et al. 2016).


Fig. 15.3
Emblem S-ICD subcutaneous defibrillator (Image provided courtesy of Boston Scientific. © Boston Scientific Corporation. © 2016 Boston Scientific Corporation or its affiliates. All rights reserved)
Leadless pacemakers (Medtronic Micra, Medtronic, Minneapolis, MN; St. Jude Nanostim, St. Jude Medical, St. Paul, MN; see Fig. 15.3) have demonstrated early success in adult patients who require only ventricular pacing (Reddy et al. 2014, 2015; Ritter et al. 2015; Reynolds et al. 2016). The devices are lodged in the right ventricle. These devices have great potential for patients with pacemaker-related tricuspid regurgitation and pacemaker lead-related venous obstruction or those patients at higher risk for pocket complications (Reddy et al. 2014) (Fig. 15.4).


Fig. 15.4
Leadless pacemakers. (a) Medtronic Micra (Medtronic, Minneapolis, MN) (Reproduced with permission of Medtronic, Inc) (b) St. Jude Nanostim in situ in the right ventricle. St. Jude Nanostim and St. Jude Medical are trademarks of St. Jude Medical, Inc., or its related companies (Reproduced with permission of St. Jude Medical, © 2016. All rights reserved)
Pacemaker devices that are MRI conditional are now commercially available (Wilkoff et al. 2011; Gimbel et al. 2013). Many CHD patients require regular tomographic imaging, and MRI, in particular, provides unique information about cardiac function, flow, and anatomy that is not available by any other modality. Although patients with conventional pacemakers have been safely placed in a scanner (Pulver et al. 2009), these devices are still considered “unsafe” in a 1.5 or 3 Tesla magnet, and pacemaker-dependent patients can never undergo MRI with conventional pacemakers. Retained temporary pacemaker wires, cut at the skin, are safe for MRI scanning, but permanent epicardial pacemaker leads can heat up in the presence of energy from the magnet (Luechinger et al. 2005). Some of the current limitations of MRI examination in patients with MRI-conditional pacemakers include the necessity to reprogram into sensing mode only or an asynchronous pacing mode that some patients cannot tolerate. Due to lack of sensing, loss of AV synchrony can adversely affect cardiac output measurements made during MR examinations (Pulver et al. 2009). Patients should be screened for abandoned leads or capped leads (even MRI-conditional leads) prior to scanning due to the potential for these leads to heat up (Mattei et al. 2015).
Blood Conservation/Bloodless Cardiac Surgery
Blood transfusion during surgery can be associated with many types of adverse events including transfusion-associated lung injury, allergic reactions, antibody formation (Eder and Chambers 2007), and infection (Allain et al. 2009). Studies have demonstrated that more liberal transfusion strategies are associated with longer lengths of hospital stay (de Gast-Bakker et al. 2013; Iyengar et al. 2013), pulmonary complications (Iyengar et al. 2013; Redlin et al. 2013), infection (Szekely et al. 2009), and renal failure (Iyengar et al. 2013). Sensitization, often a result of blood transfusion, is of concern for CHD patients who may be candidates for transplantation in the future. Jehovah’s Witness patients may decline transfusion for themselves or their children based on religious beliefs. Therefore, an approach that avoids or minimizes blood transfusion is part of a comprehensive strategy to reduce cumulative risk.
Adherence to the Society of Thoracic Surgeons and International Society for Minimally Invasive Cardiothoracic Surgery guidelines for blood conservation (Society of Thoracic Surgeons Blood Conservation Guideline Task Force et al. 2011; Menkis et al. 2012) helps to achieve bloodless surgery. For Jehovah’s Witness patients, this includes the use of erythropoietin (Alghamdi et al. 2006) (300–500 units per kilogram of body weight) injected subcutaneously twice weekly for 2–4 weeks. Supplementation with therapeutic doses of folate, iron, and a daily multivitamin is also used. One should aim to get the preoperative hemoglobin to at least 16–17 g/dL on acyanotic patients. The cardiopulmonary bypass circuit size is minimized to limit prime volumes (Kim et al. 2016). Retrograde autologous priming of the cardiopulmonary bypass circuit (Hou et al. 2009), venous antegrade prime, the use of acute normovolemic hemodilution, dilutional or balanced ultrafiltration during cardiopulmonary bypass, and modified ultrafiltration (Olshove et al. 2010) are also recommended for blood conservation (Society of Thoracic Surgeons Blood Conservation Guideline Task Force et al. 2011; Menkis et al. 2012). Cell saver autologous transfusion is also useful in reducing blood exposure (Wang et al. 2009) and can be used in Jehovah’s Witness patients if it is kept directly connected in circuit with the patient’s circulation. Thromboelastogram-directed transfusion is used in non-Jehovah’s Witness patients to allow earlier detection and treatment of bleeding. It may allow more targeted transfusion to treat bleeding (Niebler et al. 2012) and is associated with reduced transfusion prevalence (Romlin et al. 2011; Kane et al. 2016).
Intraoperative and postoperative point-of-care testing should be used as much as possible to limit blood sampling to a drop or two. All labs that cannot be performed with point-of-care testing should be drawn in pediatric tubes, even for adult patients. The need for lab tests should be reassessed daily to avoid excessive blood draws.
Pain Management
Poor pain management can cause a great deal of psychological and physiologic stress postoperatively. Therefore, strategies to prevent pain and minimize inflammation can be beneficial to early mobility and psychological recovery. Around-the-clock dosing of intravenous acetaminophen 15 mg/kg every 6 h for 72 h and intravenous ketorolac for 48 h (0.5 mg/kg every 6 h except in patients with low platelets or neonates) can be beneficial in reducing the need for narcotics in surgical CHD patients without significantly increased risk of bleeding or creatinine rise (Lieh-Lai et al. 1999; Gupta et al. 2004; Dawkins et al. 2009; Inoue et al. 2009). It also has the potential to reduce delirium associated with narcotics although this has not yet been demonstrated in a study. Local anesthetic regional nerve blockade can also reduce the need for narcotics immediately after surgery although there are no studies demonstrating this at present.
The use of intravenous dexmedetomidine has been demonstrated in a meta-analysis (Pan et al. 2016) to shorten mechanical ventilation times, lower narcotic needs, reduce stress responses as evidenced by lower blood glucose and serum cortisol levels, reduce delirium, and reduce agitation. The reduced stress response has also been shown to decrease the incidence of supraventricular and ventricular tachycardias (Chrysostomou et al. 2011) as well as junctional ectopic tachycardia (Rajput et al. 2014) which can be difficult to treat. It has been demonstrated to be safe and effective even for prolonged use in CHD patients (Bejian et al. 2009). However, there are significantly increased risks of bradycardia and hypotension (Pan et al. 2016); therefore, careful dosing and monitoring are needed in patients at risk for these side effects. A detailed discussion on pain management can be found in Chap. 42, Postoperative Pain Management.
Radiation Issues
The number of imaging studies needed by CHD patients over a lifetime can amount to very significant doses of radiation. Downing et al. (2015) reported 21 % of single-ventricle palliation patients received annualized effective doses of greater than 20 millisieverts per year and well exceed the 6.20 millisievert annual background radiation exposure for the average individual (American Nuclear Society 2016). The annual allowable exposure for those working with radioactive material is a maximum of 50 millisieverts per year (American Nuclear Society 2016). The top contributor to radiation exposure is cardiac catheterization (Ait-Ali et al. 2007; Hoffmann et al. 2007; Downing et al. 2015). Gastric emptying studies (Downing et al. 2015) and computed tomography scans (Ait-Ali et al. 2007; Hoffmann et al. 2007) are also significant contributors. Studies of cancer risk among patients with congenital heart defects indicate a higher incidence of cancer over that of the general population (Olsen et al. 2014; Videbaek et al. 2016) with adjusted hazard rates of 1.19 (95 % CI 0.69–2.04) (Videbaek et al. 2016). Increasing radiation exposure at a younger age in CHD patients, a vulnerable time when exposure correlates with elevated malignancy risk has been documented over the last several decades (Beausejour Ladouceur et al. 2016). Ait-Ali et al. (2007) estimated the lifetime attributable risk of fatal and nonfatal cancer was 1.9–2 times higher when a 1-year-old child experienced the same radiation dose as a 15-year-old child.
Radiation exposure can also shorten leukocyte telomeres in CHD patients, and this is associated with biologic aging (Vecoli et al. 2016). Telomere length is also a predictor of the development of coronary artery disease after radiation therapy (M’Kacher et al. 2015). Therefore, radiation exposure in CHD patients may predispose them to coronary artery disease as they age.
Strategies to document total radiation exposure over a lifetime and limit radiation exposure are needed. The increased use of magnetic resonance imaging (MRI) can reduce the cumulative doses of radiation. Increased implementation of MRI-safe pacemakers should increase the use of MRI in patients who would otherwise require computed tomography angiography or catheterization. Cancer screening will become increasingly important in the primary care of these patients. Due to the potential risk of atherosclerosis from radiation, primary prevention of and screening for coronary artery disease will become important for ACHD specialists.
Neurodevelopmental Issues
Neurodevelopmental issues after cardiac surgery in infancy are a well-described problem (Brosig et al. 2013; Gaynor et al. 2014, 2015; Ricci et al. 2015; Sarrechia et al. 2016). These problems can be compounded when there are coexisting genetic conditions that affect neurodevelopment. A systematic review (Hirsch et al. 2012) could not find much supporting evidence for any of the current neuromonitoring and neuroprotective techniques. However, a more recent report by Hoffman et al. (2016) showed that higher arterial saturation and narrower arterial-cerebral and arterial-somatic near-infrared spectroscopy saturation differences were associated with better or improving motor performance. Preoperative brain imaging has enlightened us to the fact that there can be abnormal brain findings in neonates born with CHD, even in the absence of intervention (Miller and McQuillen 2007; Paquette et al. 2013). Continuing work in this area to identify those individuals at greatest risk and to maximize neurodevelopmental outcomes continues. In the interim, careful assessment of these patients and early referral to occupational therapy, physiotherapy, and speech therapy will be important in trying to optimize the functioning of these individuals.
Psychological Stressors
Cumulative stressors over a lifetime can lead to maladaptive and impaired coping including post-traumatic stress disorder (PTSD) (Alonzo 2000). Surgery along with other invasive or unpleasant treatments used for CHD can precipitate maladaptive and impaired coping. Studies have demonstrated a 12 % (Connolly et al. 2004) to 29 % (Toren and Horesh 2007) incidence of PTSD in children and adolescents who have undergone CHD surgery depending on the duration of time from surgery and the instrument used to measure PTSD. In ACHD patients, Deng et al. (2016) reported an 11 % incidence of PTSD using CHD-specific PTSD measures which is significantly higher than the 3.5 % incidence in the general population. Of the traumatic events, 34 % were related to cardiac surgery, and 41 % were related other cardiovascular events including arrhythmias, heart failure, stroke, and cardiac arrest. The two factors most strongly associated with PTSD were depressive symptoms and year of most recent cardiac surgery. Earlier surgical date correlated with greater incidence of PTSD (Deng et al. 2016). In addition, 14.9 % of mothers and 9.5 % of fathers of children undergoing CHD surgery experienced PTSD 6 months postoperatively (Helfricht et al. 2008), which has the potential to impair family functioning.
Child Life Counseling/Psychological Assessment
Recognizing the high anxiety and PTSD associated with CHD surgery and intervention, the importance of adequate preparation for surgery and procedures in order to avoid traumatic experiences is important. Most pediatric centers use child life counselors to prepare children and other family members for the experience. Role-playing with props, family members, and walking through the procedure help a child and family anticipate the procedure and better cope with their emotions. If a child life counselor is not available or if the patient is an adult, patients should be referred to a psychiatrist or psychologist for pre-procedural assessment and treatment for post-traumatic events and to prepare for an upcoming procedure or surgery.
Conclusion
Thoughtful consideration of the factors discussed in this chapter should help to reduce the impact of the treatment of CHD over the course of a lifetime. This may permit improvement of both the physiological and psychological outcomes in CHD patients.
References
Allain JP, Stramer SL, Carneiro-Proietti AB, Martins ML, Lopes da Silva SN, Ribeiro M, Proietti FA, Reesink HW. Transfusion-transmitted infectious diseases. Biologicals. 2009;37:71–7.CrossRefPubMed
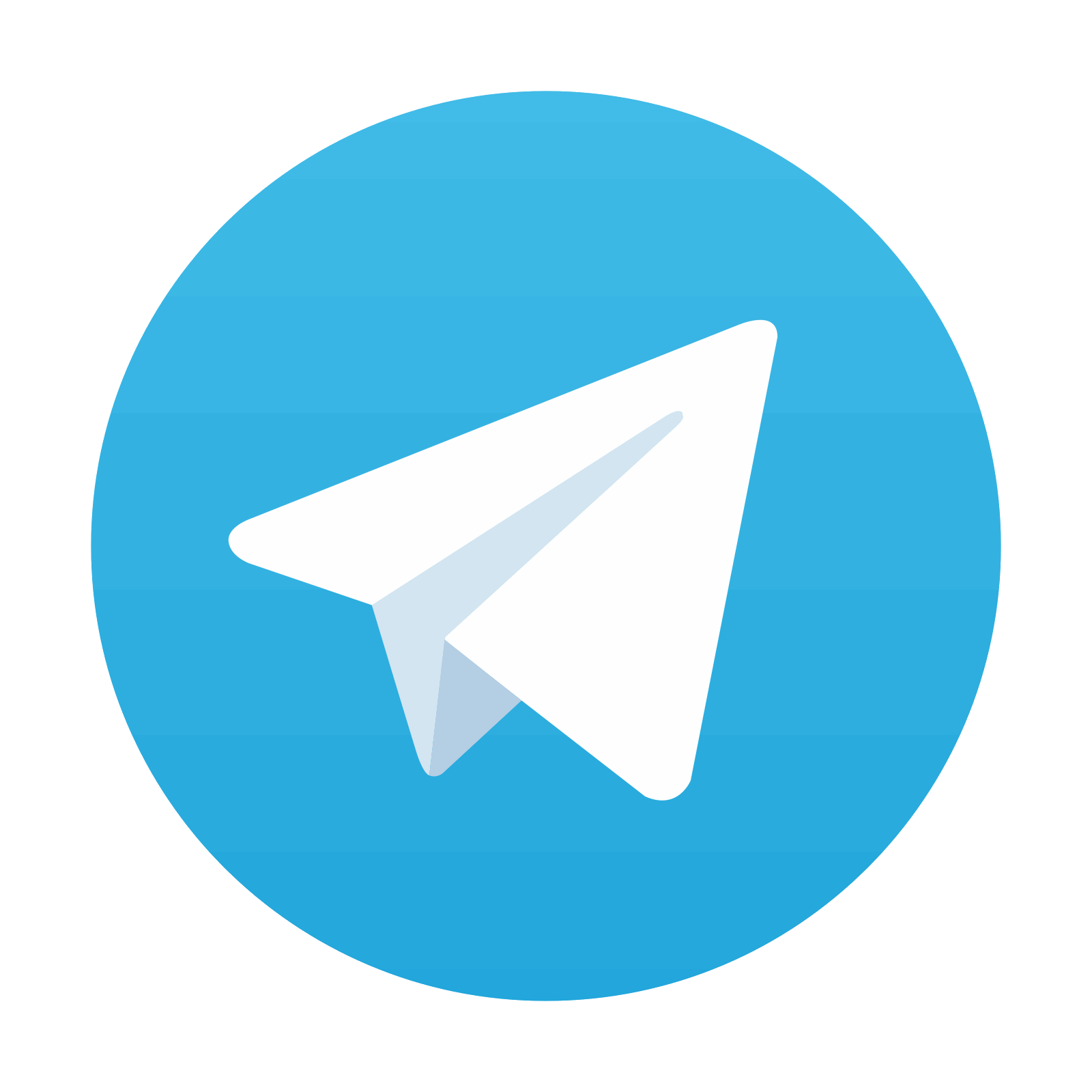
Stay updated, free articles. Join our Telegram channel

Full access? Get Clinical Tree
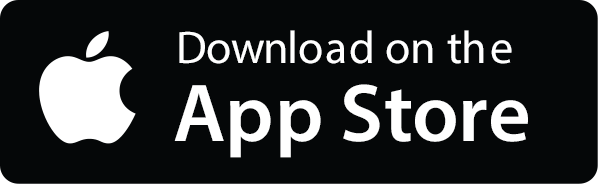
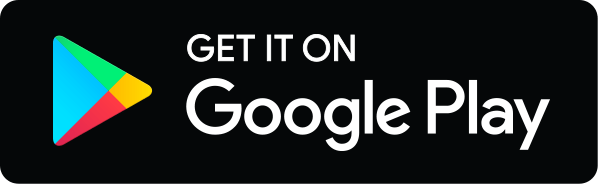