Tetralogy of Fallot, truncus arteriosus, and double outlet right ventricle with conotruncal anomalies postrepair
Secundum atrial septal defect
Ventricular septal defect
Complex single ventricles post-Fontan procedure
Coarctation of the aorta postrepair
Congenital aortic valve stenosis
Atrioventricular canal defects postrepair
Congenitally corrected transposition of the great arteries
Sinus venosus atrial septal defects with partial anomalous pulmonary venous return
Transposition of the great arteries after atrial or arterial switch
Major Categories of Congenital Cardiac Structural Defects
Adult congenital heart disease (ACHD) is composed of a variety of structural anomalies with hemodynamic consequences, which result from both the native anatomy and compensatory physiology. Due to the multitude of structural malformations, including complex forms with combined lesions, a classification system based on physiologic effects is the most useful. The four categories, which are most commonly used, include shunt lesions, mixing lesions, obstructive lesions, and regurgitant lesions. Over time, a compensatory physiology will evolve and produce aberrant loading conditions and potential impairment in ventricular function. In some patients, the compensatory changes are imperceptible and may cause little impairment in functions, while other lesions or their combined anatomy may cause significant cardiovascular compromise.
Shunt Lesions
Shunt lesions result in an aberrant communication between cardiac structures. The direction and volume of blood flow depends on the diameter and length of the communication and its relative position in the heart. The shunt lesion can occur at the level of the atria, ventricles, and great vessels or even be extra-cardiac. The flow of blood across the shunt or a vascular structure is determined by a number of factors, including the pressure drop across the structure, length, radius, resistance, and fluid viscosity. Poiseuille’s law describes this relationship in the following equation:

where Q = blood flow, ∆P = pressure change in the fluid as it flows across the shunt, r = radius of the structure, L = length of the structure, and
= the viscosity of blood. From this equation, it is seen that blood flow increases dramatically with increases in radius, increases to a lesser degree with increase in the pressure gradient across the structure, and decreases with an increase in length of the structure and blood viscosity. With a decrease in radius, a consequential decrease in blood flow to the fourth power is observed. Ohm’s law describes the change in pressure, blood flow, and vascular resistance by the following equation:
In this equation, P = the pressure generated within the cardiac chamber, Q = flow, and R = vascular resistance.


The direction and magnitude of shunt defects at the ventricular level and at the level of the great vessels, for example, a PDA, are determined by the radius of the defect and the difference in pulmonary and systemic vasculature resistance. For shunts at the level of the atrium, the ventricular diastolic pressure must also be taken into account. For small shunts, which persist into adulthood, the indication for repair is based on the potential risk of strokes from paradoxical emboli and the occurrence of bacterial endocarditis. Moderate to large shunt defects can lead to ventricular failure due to volume overload. Shunt lesions that result in chronic increases in pulmonary blood flow may result in Eisenmenger syndrome. Eisenmenger syndrome is an irreversible increase in pulmonary vascular resistance (PVR) that ultimately leads to pulmonary hypertension, right-sided heart failure, and right-to-left shunting. An anesthetic consideration regarding right-to-left shunting is that inhalation inductions may be delayed. However, these delays may be more marked with larger shunts and insoluble anesthetic gases.
Mixing Lesions
Mixing lesions occur when blood from the pulmonary and systemic circulations combine to create a mixture of oxygenated and deoxygenated blood. As with shunt lesions, mixing defects may occur at the level of the atria, ventricles, or great vessels. The ratio of pulmonary and systemic flow is again based on vascular resistance. Increased pulmonary blood flow results with elevated systemic vascular resistance (SVR). This flow pattern results in ventricular volume overload and pulmonary hypertension. Vascular medial hypertrophy of the pulmonary vessels occurs and results in increased PVR and pulmonary hypertension. In severe cases of pulmonary overcirculation, systemic malperfusion occurs with resulting metabolic acidosis, shock, and cardiovascular collapse. Conversely, elevated PVR results in increased systemic flow, decreased pulmonary flow, hypoxemia, and cyanosis. Depending on the underlying anatomy, most patients with mixing defects receive palliation or complete repair in the neonatal period. Patients with ductal-dependent lesions frequently require a mixed circulation before repair. D-Transposition of the great vessels is a defect in which a mixing lesion is required for survival and an atrial septal defect may need to be created by the Rashkind procedure (balloon atrial septostomy) to allow mixing at the atrial level and prevent a circulation in parallel. Depending on the degree of systemic hypoxemia secondary to mixing of saturated and desaturated blood, deficits in oxygen delivery may exist with resulting compensatory mechanisms, including erythrocytosis and polycythemia with resulting hyperviscosity. Patients with mixing lesions are also at risk for bacterial endocarditis and paradoxical emboli and stroke. Other considerations for patients with mixing lesions include the degree of biventricular dysfunction and loading conditions as well as the function of other organ systems.
Obstructive Lesions
Obstructive or stenotic lesions impose a ventricular pressure load proximal to the defect that results in increased intracavitary pressure and concentric ventricular hypertrophy. Initially, the wall stress is preserved; however, over time the vascular supply becomes inadequate to support the hypertrophied ventricle. The ventricle becomes relatively ischemic and dilated with increased wall tension and decreased contractility. Chronic obstructive lesions also lead to decreased ventricular compliance and diastolic dysfunction, even in cases with preserved systolic function. Patients with obstructive lesions are extremely preload dependent for adequate diastolic filling and cardiac output. Lesions, which are severely obstructive, can present with inadequate cardiac output and perfusion of the pulmonary, coronary, cerebral, and peripheral tissue beds. Significant decreases in exercise tolerance and cardiovascular reserve may be seen in patients with obstructive lesions.
Regurgitant Lesions
In general, regurgitant lesions do not usually exist as primary defects. They more commonly develop as sequelae or as a result of intervention secondary to CHD. However, worsening valvular incompetence or regurgitation results in decreases in ventricular ejection volume and function. The compensatory mechanisms to increase stroke volume cause ventricular dilation with increased wall tension and a propensity for ventricular failure. A significant number of interventional cardiac catheter-based procedures and even cardiac surgical interventions are preformed to correct lesions such as pulmonary regurgitation after tetralogy of Fallot repair in the adult congenital heart population.
Acyanotic Congenital Heart Disease
Dextrocardia
Dextrocardia is usually described as the heart having a mirror-image location in the right hemithorax. Dextrocardia with situs inversus is a mirror-image location of the intrathoracic structures as well as the intra-abdominal structures. This cardiac anomaly causes the heart to lie in the right hemithorax with the liver and gallbladder under the left portion of the diaphragm. The stomach is located under the right portion of the diaphragm and the appendix is located in the left lower quadrant. Dextrocardia with situs inversus may be functionally normal. Isolated dextrocardia with normally related abdominal viscera frequently indicates the presence of CHD. In some patients, the stomach bubble may be indistinguishable from large bowel gas because the stomach is not in its usual position, either left or right, and the liver appears to be uniformly distributed across the right and left diaphragm. This suggests visceral heterotaxy or situs ambiguous, associated with either polysplenia or asplenia and a high incidence of congenital heart malformations. In these patients, the apex may point to the left, right, or anteriorly and is referred to as mesocardia or atrial isomerism.
Dextrocardia with situs inversus usually exists in otherwise structurally and functionally normal hearts. These patients will generally have their congenital malpositioning noted when they present for noncardiac-related medical attention. The risk of noncardiac surgery in patients with dextrocardia with situs inversus and otherwise structurally normal hearts is the same as for patients with situs solitus (normally positioned abdominal viscera) and normally positioned hearts. The importance of diagnosing this anomaly lies in the correct interpretation of symptoms related to the underlying anatomic location of the organ in question. For example, patients with dextrocardia with situs inversus will experience the pain of acute appendicitis in the left lower quadrant rather than the right lower quadrant. The diagnostic importance of recognizing this anomaly has obvious surgical implications.
Congenitally Corrected Transposition of the Great Vessels
In uncorrected D-transposition of the great vessels (D-TGV), the aorta arises from the anatomic right ventricle; in corrected L-transposition of the great vessels (L-TGV), the venae cavae drain into the right atrium, which empties into an anatomic left ventricle through a mitral valve. The anatomic left ventricle ejects into the pulmonary artery (PA). In this form of transposition, the atrioarterial connections are concordant, and therefore systemic venous return enters the lung for oxygenation, and pulmonary venous return enters the left atrium and passes into an anatomic right ventricle through a tricuspid valve. Saturated blood then enters the aorta from the anatomic right ventricle. Without another major structural anomaly such as a ventricular septal defect (VSD) with or without pulmonic stenosis, patients with L-TGA may be entirely asymptomatic. There may be people with L-TGA who remain undiagnosed. However, there are a number of known associations with L-TGA, including complete heart block, VSD, pulmonic stenosis, left-sided atrioventricular (AV) valve regurgitation, Ebstein’s anomaly of the tricuspid valve, and other complex lesions. Complete heart block may result from the abnormal alignment of the ventricles and interventricular septum. This malalignment of the connection between the bundle of His and the AV node may worsen as the heart grows with a loss of continuity between the two structures and resulting third-degree or complete heart block. Eventually, these patients may require a permanent pacemaker. With L-TGA, the morphologic right ventricle (systemic ventricle) is at risk of depressed function. There is also a risk of bacterial endocarditis secondary to left AV valve regurgitation. The long-term function of the anatomic right ventricle as the systemic ventricle remains controversial. By 45 years of age, 67 % of adults with L-TGA with associated anomalies such as ASD or VSD have developed congestive heart failure (CHF). Even without associated anomalies, approximately 25 % of patients with L-TGA will have developed CHF by 45 years of age. To decrease complications from L-TGA, the “double switch” procedure can be performed in which the venous return is transposed using an atrial switch procedure and either the ventricular or arterial connections are transposed via a Rastelli procedure or an arterial switch procedure. In caring for the patient with L-TGA undergoing noncardiac surgery, one must consider the possibilities for disturbances in AV conduction, the ventricular function, and the risk of endocarditis. A preoperative ECG should be obtained, with intraoperative and postoperative ECG monitoring performed. For patients with 2:1 heart block (second-degree block), it may be prudent to place a temporary transvenous or transesophageal pacemaker. If the ventricular function is impaired, arterial line and/or transesophageal echocardiographic monitoring may be warranted.
Congenitally Bicuspid Aortic Valve
A congenitally bicuspid aortic valve may result from abnormal differentiation of the primitive truncal valve, leaving two of the aortic valve cusps fused together, producing a bicuspid instead of tricuspid valve. The bicuspid aortic valve orifice is eccentric and opens in an abnormal fashion. The abnormal orifice produces varying degrees of obstruction, which results in stenosis and increased flow velocity with a systolic ejection murmur. The risk to the patient with a bicuspid aortic valve undergoing noncardiac surgery is determined by the degree of stenosis of the valve and the ventricular function. Bacterial endocarditis is also a risk factor and prophylactic antibiotics should be considered. For patients with severe bicuspid aortic stenosis, balloon valvuloplasty may substantially reduce the gradient across the valve and offer significant symptomatic relief. Balloon valvuloplasty is only an option if the valve is thin and mobile. Adult patients with severe fibrocalcific bicuspid aortic stenosis account for 50 % of the surgically significant cases of aortic stenosis. These patients are similar to patients with acquired aortic stenosis with calcific disease of a trileaflet valve and incur similar surgical risks and anesthetic management. Calcific bicuspid aortic stenosis is even less amenable to balloon valvuloplasty than a calcified, stenotic trileaflet valve. However, in the age of transcatheter aortic valve replacement (TAVR), these patients may be candidates for a minimally invasive replacement which leaves them open in the future for a valve in a valve replacement with the deferral of open cardiac surgical intervention. As with all adults with congenital heart disease, it is important to consider the possibility of acquired coronary artery disease (CAD), and it is advisable to screen these patients for CAD. It needs to be determined whether angina is secondary to CAD or solely to the aortic stenosis with the increased oxygen demands of the hypertrophied left ventricle. For patients with bicuspid aortic stenosis, appropriate preoperative evaluation and optimization will help decrease the perioperative risk factors. Intraoperative management should be guided by the patient’s functional status and the complexity of the surgical procedure. Conventional monitoring and the use of arterial line, pulmonary artery catheter (PAC), and transesophageal echocardiography may be useful in guiding perioperative therapy. Patients must be monitored for signs of ischemia and ventricular dysfunction. Also, acute decreases in SVR will not be compensated by an increase in stroke volume secondary to the fixed left ventricular outflow tract (LVOT) obstruction.
Volume losses and hypotension must be promptly treated. Volume replacement should be pursued cautiously to avoid pulmonary edema which may result depending on the functional status of the left ventricle or systemic ventricle. Pharmacologic support of SVR and ventricular dysfunction may be necessary with inotropes. The requirement of inotropic support is best guided by the previously mentioned monitoring modalities.
Patients with bicuspid aortic stenosis are also at risk for aortic regurgitation secondary to the thickened, calcified leaflets that may become incompetent secondary to their immobile nature. The bicuspid aortic valve may also become incompetent secondary to poststenotic aortic root dilatation. Aortic regurgitation imposes a volume load on the already pressure-overloaded systemic ventricle, which may further impair ventricular function and increase the risk of bacterial endocarditis. In patients with impaired ventricular function, the mode of aortic valve replacement needs to be considered with either surgical replacement or TAVR.
Coarctation of the Aorta
Coarctation of the aorta occurs in 8–10 % of all congenital heart defects. It is more common in males than females, with a ratio of 2:1. Patients with Turner’s syndrome have a 30 % incidence of coarctation. Coarctation is an obstructive lesion that may present early and acutely in the neonatal period as critical coarctation (approximately 9 % of coarctation cases) or may be detected later in life as an incidental finding, manifesting as right upper extremity hypertension with diminished lower extremity pulses. The majority of coarctations are located close to the insertion of the ductus arteriosus into the aorta. These juxtaductal coarctations are thought in some way to be produced by contraction of the arterial wall musculature that occurs with constriction of the ductus early in the neonatal period. This may account for the acute presentation of systemic hypoperfusion in affected neonates. Other forms of coarctation are not as easily explained. Coarctation may have only mild gradients at rest, but the gradient will significantly increase with any hyperdynamic state. Unrelieved coarctation leads to upper extremity hypertension, and the consequence of sustained hypertension secondary to coarctation is that the arteries of the head and neck as well as the coronary arteries are exposed to an increased risk of developing atherosclerotic changes. The ventricular myocardium also hypertrophies, increasing the demands on the coronary arteries. The diminished pulsatility of the blood flow distal to the coarctation promotes renal secretion of renin and angiotensin, which may further promote hypertension. The most common sequelae are systolic hypertension, recurrent or residual coarctation, aortic aneurysm, aortic dissection, intracranial aneurysm, and intracranial hemorrhage secondary to intracranial aneurysm formation and sudden death. Due to the significant long-term sequelae and potential disastrous comorbidities, it is of the upmost importance to repair coarctation in a timely fashion. The longer the delay in treatment, the greater the risk of persistent hypertension after the repair. Additionally, the actual life expectancy is significantly reduced, the longer the lesion is left untreated. The overall survival after repair is 91 % at 10 years, 84 % at 20 years, and 76 % at 30 years. For patients who have undergone surgical repair, 30 % will have hypertension with a higher incidence than those repaired within the first years of life. If a coarctation is treated within the first few years of life and no significant gradient persists, the patients are expected to be able to lead essentially normal lives.
The surgical approaches to coarctation repair include resection of the stenotic area with direct end-to-end anastomosis, subclavian flap aortoplasty, or aortoplasty with a homograft or synthetic graft material. All of these techniques require the use of aortic cross clamping around the area of coarctation, with the surgical risks of paraplegia or paresis secondary to spinal cord ischemia. Residual obstruction or re-coarctation occurs in 6–33 % of all patients. The manner in which residual obstruction or re-coarctation is treated is dictated by the severity and anatomic location of the stenosis. Minimally invasive, catheter-based techniques have become the standard of care and are frequently required more than once in a given patient. Stented graft placement and balloon angioplasty of the aorta are the most common therapy required after the initial surgical repair.
Patients with a history of coarctation both unrepaired and repaired also require the following issues to be considered perioperatively:
The potential presence and functional status of a concomitant bicuspid aortic valve (as many as 85 % of patients with coarctation will have a bicuspid aortic valve)
The presence of hypertension
Systemic ventricular function
The potential for premature or concomitant CAD
The need for lifelong bacterial endocarditis prophylaxis
In addition to a complete history and physical examination, an ECG, echocardiogram, and ventricular stress test will provide a preoperative assessment of myocardial function, ventricular hypertrophy, and potential for ischemia. The patient with coarctation at the highest risk undergoing a procedure is the older patient with coexisting stenotic or an incompetent bicuspid aortic valve, depressed systemic ventricular function, and acquired CAD. If the patient is to undergo a surgical procedure and it is not urgent, intervention including coarctation repair, aortic valve replacement, coronary artery bypass or angioplasty/stenting, and medical management of the hypertension and depressed ventricular function may be undertaken to decrease the operative risk and provide optimal patient management. In patients with sequelae secondary to coarctation including systemic hypertension and depressed ventricular function, an arterial line and PAC placement are recommended to guide perioperative management. Depending on the degree of ventricular function impairment, intraoperative transesophageal echocardiography (TEE) may be helpful.
Pulmonic Stenosis
Pulmonic stenosis (PS) is an obstructive lesion of the right ventricular outflow tract that produces a pressure overload situation and compensatory changes associated with such lesions. Pulmonic stenosis may be subvalvular, valvular, or supravalvular. PS may also occur near the bifurcation and involve the branch pulmonary arteries. PS may be an isolated lesion or occur as an associated lesion with other complex malformations (tetralogy of Fallot) or as part of a syndrome such as Williams syndrome or Rubella syndrome and others. Mild PS generally causes no major hemodynamic perturbations. However, if the stenosis is severe, right ventricular hypertrophy and dysfunction may ensue. Most importantly for the patient undergoing a procedure is the limitations of compensatory responses of the systemic circulation to critical hemodynamic changes such as hypovolemia. In the case of mild PS, the risk of endocarditis exists with little hemodynamic compromise. However, for patients with severe PS and with impaired right ventricular function, their hemodynamics may be significantly improved with balloon valvuloplasty if the lesion is amenable to intervention. For emergent procedures in patients with severe pulmonic stenosis, perioperative arterial line monitoring and central venous pressure monitoring may be beneficial. Intraoperative transesophageal echocardiography (TEE) should be used for patients with PS and right ventricular or biventricular dysfunction in which loading conditions and ventricular function monitoring are required but in whom a PAC is not an option.
Primary Pulmonary Hypertension and Pulmonary Vascular Disease
Primary pulmonary vascular disease or primary pulmonary hypertension (PPH) is characterized by a decrease in the cross-sectional area of the pulmonary vascular bed caused by pathologic changes in the vascular tissue. PPH is characterized by progressive, irreversible vascular changes similar to those seen in Eisenmenger syndrome but without intracardiac anomalies. PPH is extremely rare in pediatric patients and is primarily a condition of adulthood and is more prevalent in women. It has a poor prognosis and may progress to a cyanotic condition with biventricular heart failure. Pulmonary vascular disease results from any process that produces prolonged elevation of PA pressure, such as large left-to-right shunts, obstructive airway disease, and chronic lung disease with hypoxia, and causes progressive medial hypertrophy of the pulmonary vasculature with an ultimate decrease in the cross-sectional area. The cause of PPH is not fully understood, but endothelial dysfunction of the pulmonary vascular bed may be an important factor. If severe pulmonary hypertension develops suddenly in the face of an unprepared or non-hypertrophied right ventricle (RV), right-sided heart failure will result. In patients with chronic pulmonary hypertension, gradual hypertrophy and dilatation of the RV develop and the RV pressure may ultimately exceed the systemic pressure.
A decrease in cardiac output may result from at least two mechanisms: (1) A volume and pressure overload of the RV impairs cardiac function, primarily by impaired coronary perfusion of the hypertrophied and dilated RV and decreased left ventricular (LV) function resulting from the dramatic leftward shift of the interventricular septum caused by increasing RV volume. The latter also alters LV structures and decreases LV compliance, resulting in an increase in both LV end-diastolic pressure and left atrial (LA) pressure. (2) The second mechanism may result if a sudden increase in PVR occurs with decreased pulmonary venous return to the LA and hypotension and circulatory shock results as a consequence. Pulmonary edema can occur with or without elevation of the LA pressure. Direct disruption of the walls of the small arterioles proximal to the hypoxic/constricted arterioles may be responsible similar to the mechanism which is proposed for high-altitude pulmonary edema.
Irrelevant of the cause, the clinical manifestations of pulmonary hypertension are similar when significant hypertension exists. The patient’s history will often reveal dyspnea, fatigue, and syncope on exertion. A history of CHD or CHF in infancy is present in most cases of Eisenmenger syndrome. Some patients may have a history of angina and headache. Hemoptysis is a late and occasionally fatal manifestation. Cyanosis with or without clubbing may be present. The neck veins may be distended with signs of right-sided heart failure such as hepatomegaly and ankle edema. The ECG will likely show right-axis deviation secondary to RVH, and right atrial enlargement may manifest as arrhythmias occurring in the later stage.
PPH and other types of pulmonary vascular disease are difficult to treat and nearly impossible to reverse unless the etiology is eliminated. Measures to remove or treat the underlying cause include timely surgical correction of the congenital heart defects such as ventricular septal defects (VSDs) or patent ductus arteriosus (PDA) before obstructive anatomic changes occur in the pulmonary vessels. The anesthetic management of pulmonary hypertension is guided by the underlying cause. Strategies for the intraoperative management including cases requiring cardiopulmonary bypass (CPB) and non-bypass cases need individualized assessment. The incidence of symptomatic pulmonary hypertension after repair of an intracardiac lesion does not often justify the placement of pulmonary artery catheters (PACs); however, centers that are familiar with their use have low associated morbidity. The most common strategies to manage pulmonary hypertension include avoiding maneuvers which elicit vasoconstrictive responses, maintaining oxygenation to lower pulmonary vascular resistance (PVR), maintaining alkaline pH, minimizing tidal volumes or using spontaneous modes of ventilation, and the use of pulmonary vasodilators such as nitric oxide, inhaled prostacyclin (PGI2), and, in the ICU, oral sildenafil. Nitric oxide is a direct pulmonary vasodilator with no significant systemic effect as it is rapidly metabolized by red blood cells. Nitric oxide is administered by inhalation with a special delivery system which can be added to a ventilator or anesthesia machine.
Noncardiac surgery or procedures for patients with PPH, PH, or PVD can be formidable. Fixed or elevated PVR may limit perioperative hemodynamic compensation. The potential for hypotension, RV or biventricular dysfunction, and hemodynamic instability is significant. An abrupt fall in systemic vascular resistance (SVR) may precipitate intense cyanosis. An intraoperative arterial line and PAC monitoring are recommended. In patients in whom placing a PAC is not feasible or in whom the risk of arrhythmia, RA/RV perforation, or PA rupture is believed to be unacceptably high, a TEE may be important in accessing loading conditions, in evaluating biventricular function and the magnitude of right-to-left shunting and air embolism, and in estimating PA pressures from the tricuspid regurgitant jet. TEE must be performed and interpreted by a qualified practitioner in all settings. The perioperative assessment of volume status and necessity for circulatory with inotropes is critically important in the patient with PH. The use of regional anesthesia for patients with pulmonary hypertension is recommended if appropriate for a given procedure as long as the patient does not have associated sedation which incurs significant hypoventilation. In the case of a general anesthetic, appropriate hemodynamic monitoring, airway and ventilatory management along with an appropriate depth of anesthesia will decrease the risk of perioperative instability.
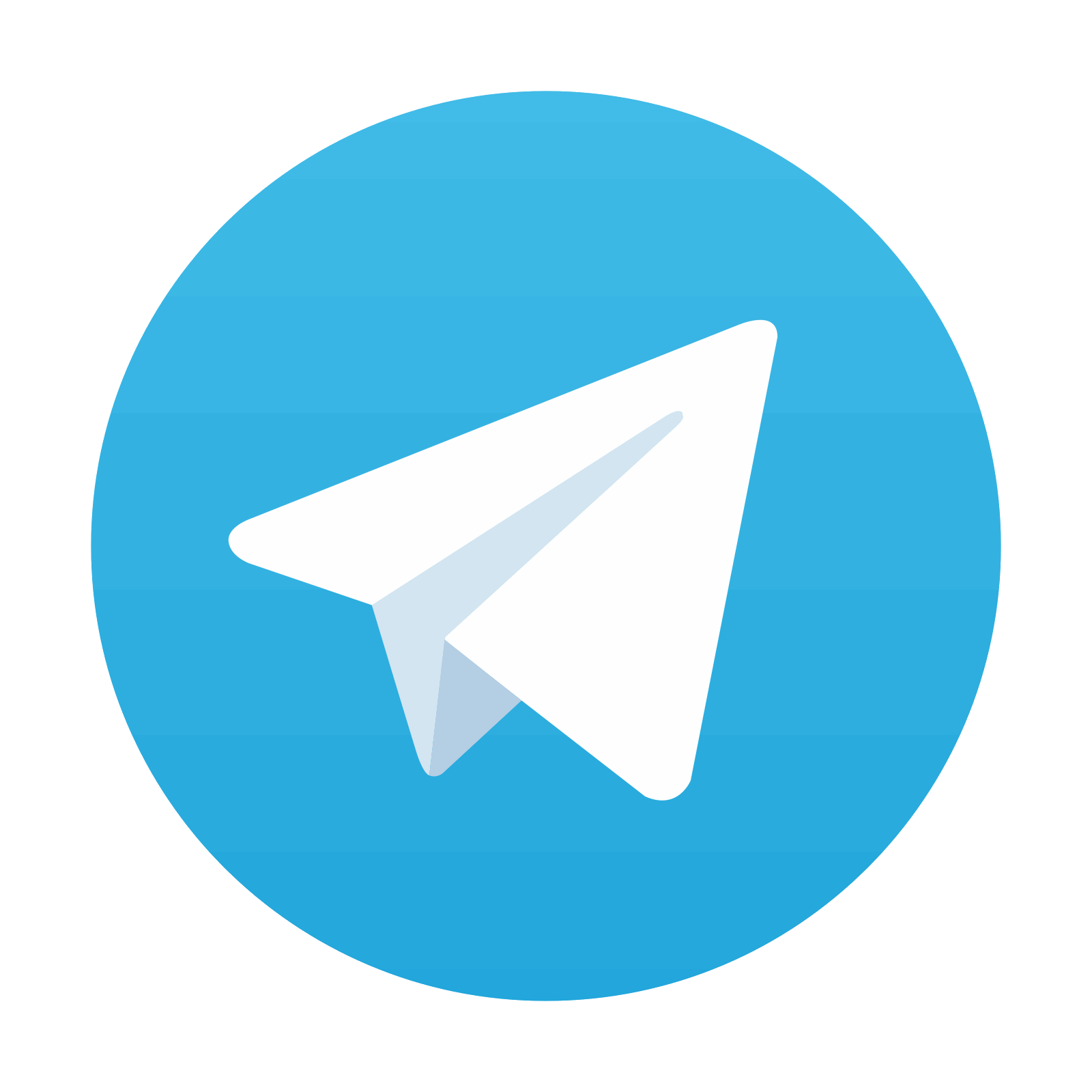
Stay updated, free articles. Join our Telegram channel

Full access? Get Clinical Tree
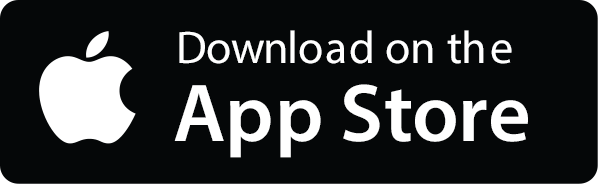
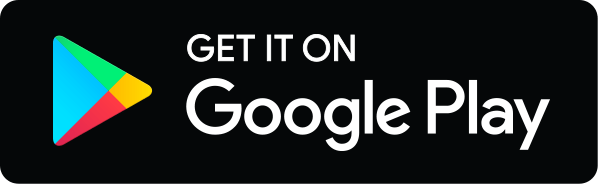