Fig. 4.1.
Fungal PAMPs. The fungal cell wall is rich in carbohydrates such as α-mannan (mannosylated proteins), β-glucan, and chitin. The cell wall is a dynamic structure capable of considerable changes, particularly during morphological transition such as yeast to hyphae. Furthermore, the composition of the cell wall varies between different fungal species. Several CLRs have been identified that recognize these cell wall structures, including transmembrane and soluble CLRs. No receptors have been identified that recognize chitin; however, it has demonstrable immunomodulatory properties
II. Pattern Recognition Receptors and Their Fungal Ligands
A. Toll-Like Receptors
The TLRs are the best-characterized family of PRR, and are part of a larger “interleukin-1/Toll-like receptor” superfamily of interleukin (IL) and Toll-like receptors. The members of this superfamily share a characteristic intracellular TIR (Toll-IL-1 receptor) domain, which functions as a protein–protein interaction motif for TIR-containing adaptors such as MyD88 and Trif. TLRs are single membrane-spanning noncatalytic receptors that bind microbial ligands and initiate signaling via MyD88 and Trif pathways. The extracellular ligand binding domain of TLRs has the characteristic horseshoe-like structure of the leucine-rich repeat (LRR) family of proteins. Upon ligand binding, TLRs homo- or heterodimerize with other TLRs or the adaptor protein MD2. TLR ligands include such diverse microbial products as bacterial lipopolysaccharide (LPS), lipoproteins, glycolipids, flagellin, double-stranded RNA, and unmethylated CpG DNA.
A number of TLRs have been implicated in fungal recognition, including TLR1, TLR2, TLR4, TLR6, TLR7, and TLR9 (Netea et al. 2008; Brown 2011; Wuthrich et al. 2012). Fungal ligands are not determined for every TLR; although we do know that TLR2 and TLR4 recognize phospholipomannan and N- or O-linked glycans, respectively, and that TLR9 recognizes fungal DNA. The individual roles of each TLR in their ability to initiate immune responses to fungi are not fully defined and often controversial. For example, TLR4 stimulates tumor necrosis factor-α (TNF-α) production and intracellular signaling during Aspergillus fumigatus, Candida albicans, and Cryptococcus neoformans infections, yet TLR4-driven cytokine production in response to C. albicans is highly variable between different strains (Netea et al. 2010), and this may be reflected by strain-dependent alterations in cell wall structure. Further examples include TLR2 recognition of C. albicans, whose activity in some mouse models is immunosuppressive, while in others it is protective (Netea et al. 2004; Villamon et al. 2004).
Mice lacking the TLR adaptor protein MyD88 are susceptible to infections with many fungal species, including C. albicans, Paracoccidioides brasiliensis, A. fumigatus, and C. neoformans, thus TLR signaling pathways are crucial for the control of fungal infection. Furthermore, even if the specific roles of TLRs in fungal infection are unclear, polymorphisms in specific TLRs have been linked to human susceptibility to fungal disease in immunosuppressed patients (Netea and van der Meer 2011). It is important to note, however, that immunocompetent individuals who lack MyD88 and other critical downstream signaling components are not predisposed (von Bernuth et al. 2008). Recent evidence demonstrates that TLRs and their signaling components can cooperate with other types of PRR, particularly CLRs, to initiate immune responses to fungi. The interaction of TLRs or MyD88 with CLRs is an integral component of antifungal immunity and will be discussed in more detail in later sections of this chapter.
B. C-Type Lectin Receptors
The CLR superfamily consists of soluble and transmembrane proteins defined by a characteristic carbohydrate recognition domain (CRD) or, more accurately, a C-type lectin-like domain (CTLD). The “C” denotes a historical requirement for calcium binding, although calcium binding is not required for all the CLRs known to date. Collectively, CLR bind nearly every fungal species that infects vertebrates, including C. albicans, A. fumigatus, Pneumocystis carinii, Coccidioides immitis, P. brasiliensis, Histoplasma capsulatum, Malassezia sp., Trichophyton sp., and C. neoformans (Taylor et al. 2005; Brown 2006; McGreal et al. 2006; Sato et al. 2006; Wuthrich et al. 2012). Many CLR are coupled either directly or indirectly to the Src family signal transduction kinase Syk, which activates MAPK, NFAT, and through the PKCδ-CARD9-Bcl10-MALT1 axis, nuclear factor-κB (NFκB) (Fig. 4.2) (Sato et al. 2006; LeibundGut-Landmann et al. 2007; Robinson et al. 2009). Genetic deletion of Syk or CARD9 in mice or humans abrogates protective immune responses to fungal particles and intact C. albicans yeasts, and these deficiencies are greater than deletions in any single CLR receptor (Rogers et al. 2005; Gross et al. 2006; LeibundGut-Landmann et al. 2007). Some CLR activate noncanonical NFκB subunits through the Raf1 kinase (Gringhuis et al. 2007, 2009b), and the signaling pathways for other CLR are unknown at the time of writing this chapter. CLR initiate immunity to fungi through stimulating phagocytosis, respiratory burst, and the release of cytokines that polarize adaptive responses toward protective Th1/Th17 immunity. Here we will cover the transmembrane and soluble CLRs that are known to initiate immunity against fungi.
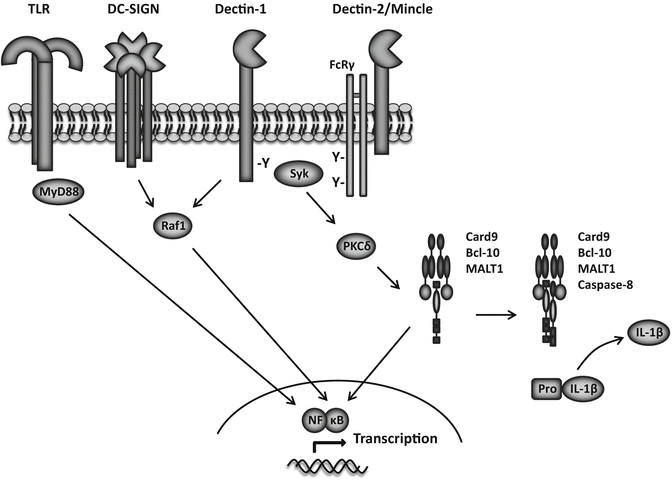
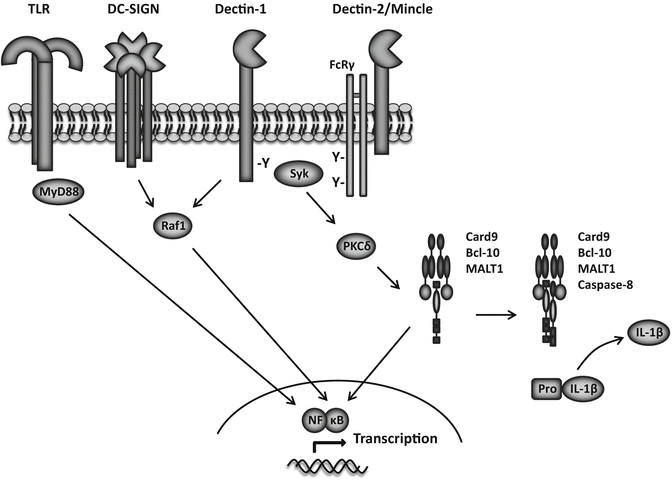
Fig. 4.2.
PRR signal transduction pathways. Transmembrane PRRs involved in antifungal immunity and their intracellular signaling pathways. Dectin-1, Dectin-2, and Mincle induce intracellular signaling via immunoreceptor tyrosine (Y)-based activation motifs, which recruit and activate Syk kinase either directly or indirectly through the FcRγ adaptor chain. Syk signals through protein kinase C (PKC)δ to activate the Card9–Bcl-10–Malt1 complex, inducing gene transcription and the production of various inflammatory mediators. When caspase-8 is included in this complex, it can cleave pro-IL-1 β into is biologically active form. Dectin-1 additionally signals through MAPK and NFAT; DC-SIGN and Dectin-1 can signal via the Raf-1 kinase pathway, which modulates the activity of other signaling pathways and gene transcription. TLR primarily signal through MyD88, but can also utilize TRIF
1. Dectin-1
Dendritic cell-associated C-type lectin receptor-1 (Dectin-1) was initially identified in a subtractive cloning screen as a dendritic cell-associated receptor with unknown function (Ariizumi et al. 2000b). Later, zymosan screening of a macrophage cDNA expression library identified Dectin-1 as the first known pattern recognition receptor for β-glucan (Brown and Gordon 2001). The gene encoding for Dectin-1, CLEC7a, is found within the natural killer cell (NKC) gene complex within a cluster of genes encoding for several PRRs that is now known as the Dectin-1 cluster (Huysamen and Brown 2009). Dectin-1 contains an immunoreceptor tyrosine-based activation motif (ITAM)-like domain (also called a Hemi-ITAM) in its cytoplasmic tail that mediates cellular activation through the phosphorylation of a key tyrosine residue that serves as a docking site for the signal transduction kinase Syk. Dectin-1 lacks calcium-binding motifs and thus does not require calcium for ligand recognition.
Dectin-1 selectively and specifically binds β-1,3-glucans, which are structural carbohydrates in the fungal cell wall (Gow et al. 2012); interestingly there seems to be an unidentified endogenous ligand present on T cells (Ariizumi et al. 2000b). β-glucan-containing particles such as zymosan and curdlan are potent Dectin-1 activators often used to probe Dectin-1 function in vitro, and the activity of these ligands can be competitively inhibited by the soluble β-glucans glucan phosphate and laminarin. Dectin-1 utilizes the signal transduction kinase Syk to activate MAPK, NFAT, and through the PKCδ-CARD9-Bcl10-MALT1 axis, NFκB (Gross et al. 2006; Goodridge et al. 2007; LeibundGut-Landmann et al. 2007; Sancho and Reis e Sousa 2012; Strasser et al. 2012). Furthermore, Dectin-1 can activate noncanonical NFκB subunits independently of Syk through the serine and threonine kinase Raf-1 (Gringhuis et al. 2009b), though it is unclear how Raf-1 interacts with the receptor itself. Interestingly, Dectin-1 signaling is only accomplished upon clustering into a “phagocytic synapse” from which the regulatory tyrosine phosphatases CD45 and CD148 are excluded (Goodridge et al. 2011).
Dectin-1 binds a range of fungal pathogens, and its importance to antifungal defense is evidenced by susceptibilities to A. fumigatus, C. albicans, and P carinii infections in Dectin-1-deficient mice. Dectin-1 is expressed primarily on cells of myeloid lineage, specifically macrophages, dendritic cells, monocytes, and neutrophils, although it is also found on an innate-like subset of T cells, γδ T cells (Martin et al. 2009). Dectin-1 engagement on myeloid cells initiates phagocytosis, respiratory burst, and release of inflammatory mediators including eicosanoids and cytokines/chemokines such as TNF-α, IL-1β, IL-6, IL-23, CCL2, CXCL1, and CCL3. These effects are mediated by the Syk/CARD9 signaling pathway in all cell types except for macrophages, in which Dectin-1 elicits phagocytosis independently of Syk (Herre et al. 2004). Indeed, in macrophages, Syk/CARD9 is only required for respiratory burst, and this response is restricted to a subset of cells that are primed to use this kinase (Underhill et al. 2005). Interestingly, myeloid usage of Syk/CARD9 is cell-type-specific, with only bone marrow-derived dendritic cells, peritoneal macrophages, alveolar macrophages, and GM-CSF or interferon (IFN)-γ primed bone marrow-derived macrophages being able to produce robust TNF-α following Dectin-1 stimulation (Goodridge et al. 2009a). Additionally, dendritic cells stimulated by Dectin-1 activate naïve CD4 + T cells and direct their polarization towards protective Th1/Th17 effector cells. Th17 immunity is crucial to antifungal defenses at mucosal sites of infection such as the oral mucosa (Conti et al. 2009); furthermore, during pulmonary infection with A. fumigatus, Dectin-1 suppresses IL-12 and IFN-γ production, favoring Th17 differentiation (Rivera et al. 2011). Interestingly, Dectin-1-stimulated dendritic cells instruct a percentage of regulatory T cells (Treg) to become IL-17 secreting effector cells (Osorio et al. 2008).
Th17 effector cells require IL-1β, IL-6, and IL-23 for polarization and maintenance; however, IL-1β is initially produced as a pro-peptide that requires cleavage to mature to its bioactive form. The inflammasome is a complex of cytoplasmic proteins responsible for the cleavage of pro-IL-1β, and Dectin-1 stimulation leads to the activation of the inflammasome and IL-1β production. Dectin-1-derived signals are translated to NLRs such as NLRP3 or NLR4C by an unknown mechanism that activates caspase-1 and initiates IL-1β processing; additionally, a direct link to IL-1β processing from Dectin-1 through a noncanonical inflammasome involving caspase-8 has also been described (Gringhuis et al. 2012).
The importance of Dectin-1 in antifungal immune defense is evidenced by human polymorphisms that lead to susceptibility to fungal infection. One polymorphism in Dectin-1, Y238X, generates an early stop codon, resulting in a truncated nonfunctional receptor. This mutation has been identified in patients with recurrent mucosal candidiasis, and cells from these patients have poor cytokine responses to C. albicans and β-glucan (Ferwerda et al. 2009). Interestingly, individuals with CARD9 polymorphisms have a more severe phenotype than that of Dectin-1 (Glocker et al. 2009). CARD9 deficiency renders humans susceptible to infection with Candida and results in complete Th17 deficiency and altered Th1 responses (LeibundGut-Landmann et al. 2007; Glocker et al. 2009). Reduced Th17 responses also correlate with susceptibility to mucocutaneous infections in Dectin-1-deficient humans (Ferwerda et al. 2009). Indeed, various defects in Th17 immunity, including mutations in STAT1, STAT3, IL-17, and IL17RA have been linked to susceptibility to mucocutaneous infections, especially chronic mucocutaneous candidiasis (CMC) (Ma et al. 2008; Milner et al. 2008; Liu et al. 2011; Puel et al. 2011; van de Veerdonk et al. 2011b). Furthermore, other diseases characterized by susceptibility to CMC, such as autoimmune polyendocrine syndrome 1, are also associated with alterations in Th17 immunity (Kisand et al. 2010; Puel et al. 2010). Recently, a polymorphism in Dectin-1 has also been linked to a severe form of ulcerative colitis, implicating a crucial role for this receptor in modulating fungal responses in the microbiome (Iliev et al. 2012).
2. Dectin-2
Dectin-2 was originally identified as an overexpressed transcript in a murine myeloid leukemia model (Fernandes et al. 1999), and was also identified as a dendritic cell-specific lectin in the subtractive cloning screen that identified Dectin-1 (Ariizumi et al. 2000a). Since the generation of an anti-Dectin-2 antibody, it has also been found on tissue macrophages and peripheral blood monocytes (Taylor et al. 2005). Dectin-2 is selective and specific for α-mannans, such as those found in the fungal cell wall (McGreal et al. 2006; Robinson et al. 2009). Dectin-2 is structurally similar to Dectin-1; however, its cytoplasmic tail is truncated and thus lacks an ITAM domain. Instead, Dectin-2 couples with the ITAM-containing FcRγ to activate Syk and, subsequently, the PKCδ-CARD9-Bcl10-MALT1 axis (Sato et al. 2006). The Dectin-2 CRD contains an EPN (Glu-Pro-Asn) motif that often indicates mannose specificity and is also found in the CLRs DC-SIGN, MBL, and MR. The gene encoding for Dectin-2 (CLECf4n) is also found within the NKC gene complex, in a cluster of genes adjacent to the Dectin-1 cluster. Genes encoded in the Dectin-2 cluster include another important fungal CLR, Mincle, as well as PRRs involved in cell turnover and homeostasis, such as BDCA-1, DCIR, and DCAR. Each of these receptors in the Dectin-2 cluster couple to FcRγ to initiate signaling, except for DCIR, whose cytoplasmic tail contains an inhibitory immunoreceptor (ITIM) signaling motif (Graham and Brown 2009).
Like Dectin-1, Dectin-2 also binds zymosan particles with high affinity; interestingly, however, when it comes to C. albicans Dectin-2 is thought to preferentially bind the hyphal morphology whereas Dectin-1 preferentially binds yeasts, although there is plenty of evidence to suggest that Dectin-1 will bind hyphae and Dectin-2 yeasts (Gantner et al. 2005; Sato et al. 2006; Saijo and Iwakura 2011). In fact, cytokine production in response to C. albicans hyphae is only mildly abrogated in macrophages from Dectin-2-deficient mice, indicating that other receptors are clearly involved. Dectin-2 is known to bind a variety of fungal species, including C. albicans, unencapsulated C. neoformans, H. capsulatum, P. brasiliensis, and the dermatophyte species Microsporum and Trichophyton (Sancho and Reis e Sousa 2012). Ligation of Dectin-2 results in yeast phagocytosis and release of inflammatory cytokines, including TNF-α, IL-1β, IL-6, IL-12, and IL-23 (Robinson et al. 2009; Saijo et al. 2010). Dectin-2 signaling drives antifungal Th17 responses, and Dectin-2-deficient mice are impaired in Th17 immunity and are thus susceptible to C. albicans infection; however, Dectin-2 appears to collaborate with Dectin-1 to modulate Th1 immunity, rather than directly drive Th1 immunity on its own (Robinson et al. 2009; Saijo et al. 2010). Interestingly, Dectin-2 can also drive Th2 responses during allergy (Barrett et al. 2011). No human Dectin-2 polymorphisms have been identified to date.
3. DC-SIGN
The dendritic cell-specific intercellular adhesion molecule-3-grabbing non-integrin receptor (DC-SIGN, CD209) in the human genome is found within a cluster of genes that encode for three related members of a “DC receptor” class, so named for their homology to DC-SIGN. These other members include CD23, a characteristic surface molecule of B cells, and SLectin, which is found on liver endothelium (Geijtenbeek et al. 2000). The murine DC-SIGN locus contains eight paralogues of CD209 (SIGNR1 to SIGNR8), though SIGNR6 is a pseudogene. SIGNR3 appears to be the closest relative to human DC-SIGN, as it is the only isoform that recognizes terminal mannose; however, SIGNR1 may also bind fungi (Powlesland et al. 2006). DC-SIGN is found on dendritic cells of the skin and mucosa and some subsets of tissue macrophages. Structurally, DC-SIGN contains a characteristic C-type lectin CRD with a mannan-binding EPN motif, transmembrane stalk, and intracellular tyrosine implicated in signaling. It functionally exists in the membrane as a tetramer, which lends a great amount of flexibility to ligand binding, and organizes itself into nanoclusters in the membrane (Cambi et al. 2004; Manzo et al. 2012). DC-SIGN binds mannose and fucose linkages of diverse microbial origin, including fungi, bacteria, and viruses. Though DC-SIGN contains an intracellular tyrosine it has not been directly associated with signaling; however, it does mediate phagocytosis of fungi, and plays a modulatory role in cytokine production. Like Dectin-1, DC-SIGN can activate noncanonical NFκB through Raf-1 (Gringhuis et al. 2007). Interestingly, DC-SIGN utilizes Raf-1 to collaborate with various TLRs to modulate cytokine signaling. Raf-1 activity results in acetylation of the p65 subunit of NFκB, but only after NFκB is previously activated by TLR3, TLR4, or TLR5. This NFκB acetylation leads to increased NFκB binding affinity at cytokine genes, including those coding for IL-6, IL-10, and IL-12. Thus, while Raf-1 activation by DC-SIGN is independent of TLRs, TLR signaling must first activate NFκB for Raf-1 to have its modulatory effects (Gringhuis et al. 2007). Furthermore, depending on whether mannose or fucose is presented, Raf-1 may be excluded from the signaling complex, resulting in differential cytokine expression. For example, Raf-1 is excluded upon recognition of Helicobacter pylori, a fucose-containing bacteria (Gringhuis et al. 2009a).
4. Mannose Receptor
Receptors with fungal mannose-binding capability have been described since the early 1980s, and, as such, the mannose receptor (MR, CD206, Clec13D) was the first characterized fungus-binding receptor (Jouin et al. 1981; Berton and Gordon 1983). MR is the prototypical member of the MR family of lectins that includes Dec205, Endo180, and phospholipase A2 receptor (PLA2R), which are all collagen-binding endocytic receptors involved in the clearance of endogenous ligands. MR is expressed on macrophages, inflammatory dendritic cells, and some non-myeloid cells such as liver endothelium. MR recognizes terminal mannose residues of α-mannan from a variety of microbial sources as well as putative endogenous ligands (Linehan et al. 2001). The MR family of receptors has a unique structure compared to other CLRs, containing multiple CRD domains. MR itself has eight CRDs that bind carbohydrate moieties with varying specificity, although five of these (CRD4-8) are adequate to reproduce the binding affinity of the full-length molecule (Taylor et al. 1990, 1992). MR does not contain any known consensus sequence for signal transduction, and no signaling pathways have been identified for it; therefore, MR probably exists as a modulatory receptor that facilitates the activities of canonical PRRs (Martinez-Pomares 2012). The MR has been implicated in the recognition of several fungi in vitro, yet in mice this receptor appears to only be required for protective immunity to infections with C. neoformans (Lee et al. 2003; Dan et al. 2008). Although its signal transduction mechanisms are unknown, MR activation is known to promote the development of Th17 responses against C. albicans; however, MR knockout mice are not susceptible to infection with C. albicans or P. carinii (Lee et al. 2003; Swain et al. 2003; van de Veerdonk et al. 2009).
5. Mincle
The macrophage-inducible C-type lectin, Mincle (Clec4e, Clecsf9), is located in the Dectin-2 cluster of the NKC gene complex and is structurally similar to Dectin-2. Mincle couples to Syk via the FcRγ to activate the PKCδ-CARD9-Bcl10-MALT1 axis, although Mincle does not induce phagocytosis, only cytokine production, in response to fungal ligands (Wells et al. 2008). Mincle is constitutively expressed at a low level on myeloid cells, and can be induced by LPS or cytokines such as IFN-γ. Although the FcRγ is not necessary for surface expression of Mincle, upregulation in response to LPS stimulation is abrogated in FcRγ-deficient mice (Yamasaki et al. 2008). Interestingly, the expression pattern of Mincle in response to C. albicans is similar to that of TLR2 (Wells et al. 2008). Mincle recognizes α-mannan in the cell wall of C. albicans, F. pedrosoi , and Malassezia sp., is a receptor for mycobacterial cord factor, and plays a major homeostatic role in sensing necrosis, namely through recognition of the endogenous ligand SAP130 (Brown 2008; Bugarcic et al. 2008; Wells et al. 2008; Yamasaki et al. 2009). Using an NFAT-GFP reporter cell line, Mincle was found to specifically recognize several strains of Malassezia sp., and Mincle-deficient mice challenged with Malassezia produced less TNF-α, IL-10, CXCL1, and CXCL2 than wild-type mice (Yamasaki et al. 2009). Mincle is also necessary for proinflammatory TNFα responses to F. pedrosoi, particularly during TLR co-stimulation (see below) (Sousa Mda et al. 2011). Mincle-deficient mice are more susceptible to infection with C. albicans; however, there are no differences in survival between wild-type and Mincle-deficient mice when given a lethal dose of C. albicans, suggesting that Mincle plays a regulatory role for the receptor during Candida infection that can be compensated for (Wells et al. 2008). Although there is no data as yet for Mincle-mediated polarization of adaptive responses to fungi, it is known that Mincle is essential for driving Th17 responses to mycobacterial factors (Schoenen et al. 2010).
6. Collectins
Collectins are a family of soluble, secreted C-type lectins. Structurally, they contain the characteristic C-type lectin CRD, which is linked to a collagen-like arm, and individual subunits combine to form a functional multimeric protein (see Table 4.1). In mice, this class is composed of surfactant proteins A and D (SP-A, SP-D) and two mannose-binding lectins (MBL1 and MBL2), whereas in humans there are two SP-A isoforms and only one MBL. Mannose and terminal mannan are the primary ligands that SP-A, SP-D, and MBL bind, and together these receptors have been found to bind nearly every known human fungal pathogen, including A. fumigatus, B. dermatitidis, C. albicans, C. neoformans, H. capsulatum , and P. carinii (Brummer and Stevens 2010). Although lung surfactants are required for respiration by reducing surface tension at the air–liquid interface, their immune role is to opsonize yeast cells or conidia, causing aggregation of the fungal particles and resulting in enhanced phagocytosis and oxidative burst by macrophages. Surfactant-opsonized zymosan particles are taken up by macrophages as aggregates, an effect that can be reversed by EDTA (Faro-Trindade et al. 2012). Uptake of surfactant-opsonized particles has been suggested to occur via interaction with opsonic receptors such as C1q (Eggleton et al. 2000), and cytokine responses are thought to occur in collaboration with other PRRs or a putative SP-A receptor (Gardai et al. 2003; Kuroki et al. 2007). However, recent studies have suggested that the only major function of surfactant in fungal recognition is particle aggregation (Faro-Trindade et al. 2012). SP-A and SP-D are produced by type II alveolar cells and Clara cells in the airways; MBL is produced in the liver and released into the plasma for distribution. MBL is an opsonic factor in the lectin pathway of complement activation. MBL recognizes antibody–antigen complexes and interacts with proteases such as C1r or MBL-associated serine proteases (MASPs), which then activate the lectin pathway of complement. Interestingly, low MBL levels have been associated with susceptibility to mycoses in humans (Mullighan et al. 2002; Granell et al. 2006). SP-D-deficient mice are susceptible to pulmonary A. fumigatus infection, which can be reversed by administration of exogenous SP-D; however, SP-A-deficient mice are more resistant to A. fumigatus, a result that is characterized by higher IL-4 to IFN-γ ratios (Madan et al. 2010).
Table 4.1.
C-type lectin receptors in antifungal Immunity
Receptor | Selected ligands | Signal transduction pathways | Major cell types expressing the receptor | Structure | Selected references |
---|---|---|---|---|---|
Dectin-1 | β-1,3-Glucan | Syk/CARD9 | Dendritic cells | ![]() | Brown (2011) |
Aspergillus | Raf-1 | Macrophages | Brown et al. (2003) | ||
Candida | MAPK | Monocytes | LeibundGut-Landmann et al. (2007) | ||
Coccidioides | NFAT | Neutrophils | Taylor et al. (2007) | ||
Cryptococcus (spores) | |||||
Pneumocystis | |||||
Dectin-2 | α-Mannan | Syk/CARD9 (via FcRγ) | Dendritic cells | ![]() | Sancho and Reis (2012) |
Candida | Macrophages | McGreal et al. (2006) | |||
Cryptococcus (acapsular) | Inflammatory monocytes | Robinson et al. (2009) | |||
Histoplasma | Saijo et al. (2010) | ||||
Paracoccidioides | |||||
Trichophyton | |||||
Microsporum | |||||
DC-SIGN | α-Mannan | Raf-1 | Dendritic cells | ![]() | Gringhuis et al. (2007) |
Aspergillus | Macrophages | van den Berg et al. (2012) | |||
Candida | |||||
Paracoccidioides | |||||
MR | α-Mannan | Unknown | Macrophages | ![]() | van de Veerdonk et al. (2009) |
Candida | Inflammatory dendritic cells | Martinez-Pomares (2012) | |||
Cryptococcus | Liver epithelium | McGreal et al. (2005) | |||
Pneumocystis | |||||
Mincle | α-Mannan | Syk/CARD9 (via FcRγ) | Macrophages | ![]() | Brown (2008) |
Candida | Wells et al. (2008) | ||||
Malassezia | |||||
SP-A SP-D MBL | α-Mannan Aspergillus Blastomyces Candida Cryptococcus Histoplasma Pneumocystis | Complement (C1q) Modulates other PRR | Secreted by lung epithelium (SP-A, SP-D) Secreted by liver epithelium (MBL) | ![]() ![]() ![]() | Kuroki et al. (2007) Brummer and Stevens (2010) |
C. NOD-Like Receptors
Nucleotide oligomerization domain (NOD)-like receptors (NLRs) are a family of more than 20 cytoplasmic proteins that regulate inflammatory and apoptotic processes, including the processing and activation of pro-IL-1β and pro-IL-18 in a multiprotein complex termed the “inflammasome” (Franchi et al. 2012). Both of these cytokines are essential for protective antifungal immunity, particularly for driving the development of protective Th1 and Th17 responses (van de Veerdonk et al. 2011a). Two NLRs (NLRP3 and NLRC4) and their inflammasome components, including the adaptor molecule ASC and the protease caspase-1, are implicated in mediating IL-1β and IL-18 responses to fungi (Netea et al. 2003; Vonk et al. 2006; Gross et al. 2009; Hise et al. 2009; Said-Sadier et al. 2010; van de Veerdonk et al. 2011a). Both NLRs are required for controlling mucosal infections with Candida, but only NLRP3 is involved in preventing dissemination (Hise et al. 2009; Tomalka et al. 2011). CLRs and TLRs can induce pro-IL-1β in response to fungi, but how the NLRs actually sense fungal pathogens is still unclear. Activation of the NLRP3 inflammasome in response to A. fumigatus and C. albicans requires Syk kinase, as well as respiratory burst and potassium efflux, which is suggestive of a direct involvement of CLRs (Gross et al. 2009; Hise et al. 2009; Said-Sadier et al. 2010). One inflammasome pathway, mediated by caspase-8 cleavage, is directly stimulated via Dectin-1 through Syk and does not require ASC or NLRs; instead caspase-8 is integrated into the CARD9-Bcl10-MALT1 complex via binding to MALT1 (Gringhuis et al. 2012) (Fig. 4.2).
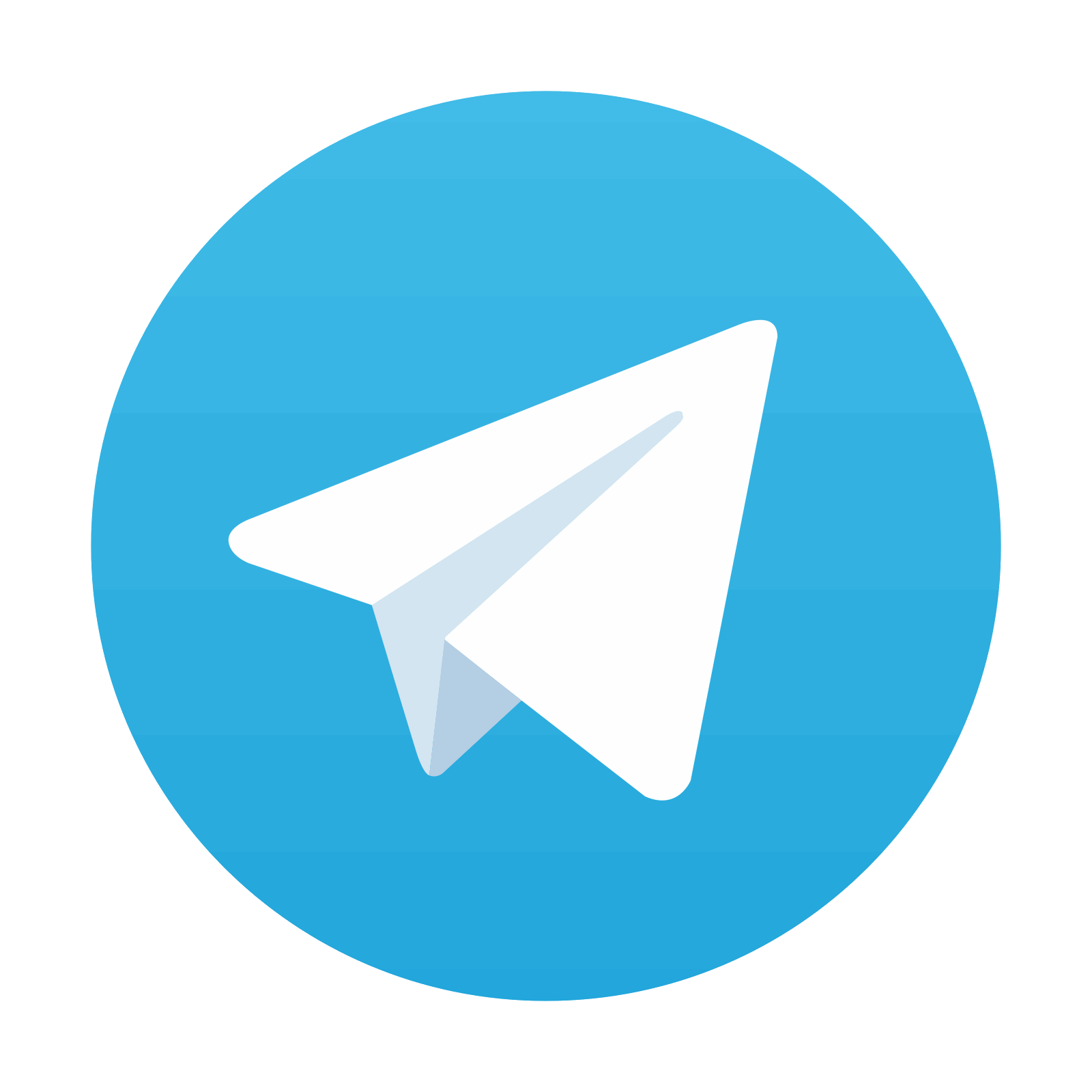
Stay updated, free articles. Join our Telegram channel

Full access? Get Clinical Tree
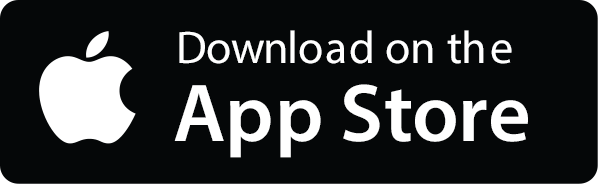
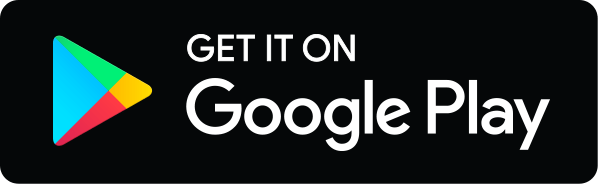