Fig. 13.1.
Angioinvasive aspergillosis in a 35-year-old man with acute lymphatic leukemia. CT scan shows bilateral peribronchovascular nodular lung lesions with surrounding halo of ground-glass attenuation representing adjacent hemorrhage
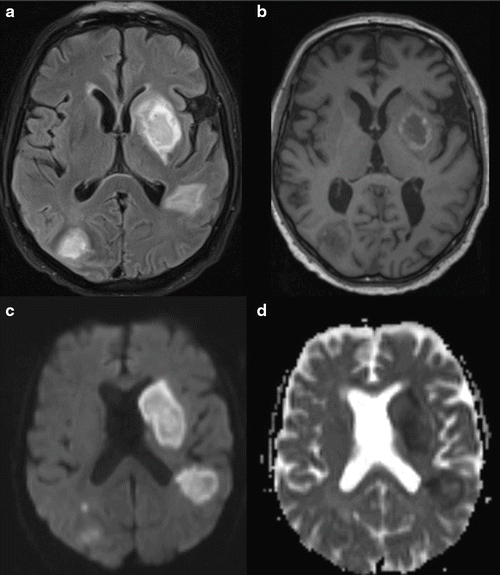
Fig. 13.2.
Invasive aspergillosis of the brain in a 70-year-old male patient with lung carcinoma and chemotherapy. (a) Axial T2 tirm MR image shows bihemispheric hyperintense lesions. (b) Unenhanced axial T1 weighted MR image shows bihemispheric hypointense lesions with a rim of high signal intensity representing blood products. (c) Diffusion-weighted images shows high signal intensity in all lesions. (d) Apparent diffusion coefficient (ADC) map shows low signal intensity in the center of the lesions, a finding indicative of restricted diffusion
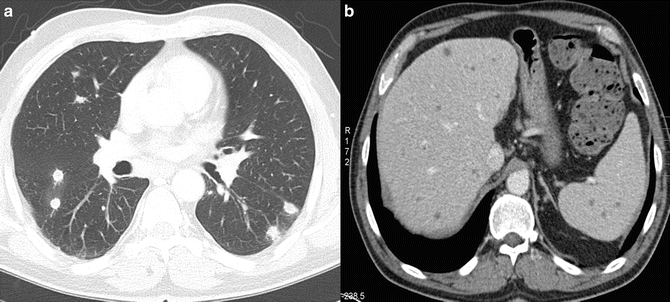
Fig. 13.3.
Systemic candidiasis in a 40-year-old male patient with chronic myeloic leukemia. (a) Axial CT scan shows multiple bilateral intrapulmonary nodules smaller than 1 cm. (b) Axial contrast-enhanced CT shows countless hypoattenuating lesions in the liver and the spleen representing Candida microabscesses
V. Classical Laboratory Diagnosis
A. Pre-analytical Considerations in Diagnosing Fungal Infections
As with other microbial infections, the diagnosis of fungal infections depends upon a combination of clinical observation and the application of various laboratory techniques. Superficial fungal infections are often associated with suspicious lesions, which guide clinical diagnosis. In contrast, invasive fungal infections rarely show specific signs. As a consequence, the selection, collection, possible storage, and transportation of diagnostic material are of utmost importance in order to establish the diagnosis. During transportation, fungi must remain viable in order to be recovered on culture, and specimens should not be allowed to desiccate. Transportation to the laboratory should occur at room temperature. Specimens should be delivered within 2 h after sampling but should not arrive at the laboratory later than 24 h after collection. Specimen collections from the central nervous system should be processed as soon as possible. When immediate processing is not possible, the specimen should be held at ambient temperature (CLSI 2012).
To establish or confirm diagnosis, it is essential that the laboratory is provided with an adequate specimen. For the diagnosis of invasive fungal infections, specimens originating from sterile locations are preferred. Blood cultures should be performed whenever deep fungal infections are suspected. All Candida species or other organisms such as Histoplasma spp. have been shown to be reliably detected by the current available blood culture systems. For aspergillosis and mucormycosis, blood cultures are of limited utility. However, disseminated infections caused by Fusarium spp. and Scedosporium spp. are associated with the recovery of the pathogen in blood cultures in approximately 50% of all cases (Arendrup et al. 2012).
In case of suspected meningitis, 3–5 ml of cerebrospinal fluid (CSF) should be collected in sterile, screw-capped tubes. Immediate transport of all collected fluid to the laboratory at room temperature is mandatory (CLSI 2012). CSF is best for detection of Cryptococcus meningitis (Denning et al. 2003), but is less sensitive for the detection of central nervous system aspergillosis or candidiasis (Arendrup et al. 2012).
Tissue biopsies are of high diagnostic value. However, for some fungi (e.g. Mucorales), homogenization of the biopsy material reduces the culture yield and is it preferable to inoculate culture plates with slices of minimally manipulated tissue (Arendrup et al. 2012).
The confirmation of many fungal infections is based on samples obtained from lower respiratory tract specimens. Sputum specimens are acceptable, but increasing the number of sputum samples examined increases the sensitivity of detection, with three samples providing optimum yield in invasive aspergillosis. Bronchoalveolar lavage (BAL) fluid provides a more representative sample from the lower respiratory tract and allows CT scan abnormalities to be directly sampled. The presence of Candida spp. in BAL fluid, similarly, does not correlate with invasive lung infection and should be interpreted as of low significance (Meersseman et al. 2009; Arendrup et al. 2012).
B. Currently Available Diagnostic Methods
Currently available laboratory methods for diagnosing invasive fungal infections include microscopic detection, isolation of the fungus, serologic detection of antibodies and antigen, and histopathologic evidence of invasion (Alexander 2002). For definite diagnosis of proven invasive fungal infections, histological and cultural evidence from biopsies, resection material, or other specimens obtained from normally sterile body sites is required (De Pauw et al. 2008). However, it is not always possible to fulfill these criteria and a combination of different methods is necessary to detect and identify the fungal organism causing the disease.
1. Histopathology
Histopathology is the cornerstone for diagnosis and identification of fungal pathogens. However, as with culture methods, it may not be possible to obtain a tissue sample from critically ill patients. In addition, identifying the specific pathogen based solely on morphological characteristics can be difficult or impossible because several different organisms may have similar histopathological characteristics, e.g., Fusarium spp. and other filamentous fungi are indistinguishable from Aspergillus in tissue biopsies (Alexander and Pfaller 2006). Because Aspergillus is far more commonly encountered than the other pathogens mentioned, a pathologist often may describe an organism as Aspergillus or Aspergillus-like on the basis of morphological features alone. This can hinder diagnosis and may entail inappropriate therapy (Chandrasekar 2010). Molecular tools can aid in the identification of fungal pathogens from histopathology samples (see Sect. 6).
2. Microscopy
Direct microscopy is most useful in the diagnosis of superficial and subcutaneous fungal infections. Recognition of fungal elements can provide a reliable and rapid indication of the mycosis involved. Various methods can be used: unstained wet-mount preparations can be examined by light-field, dark-field, or phase contrast illumination (Richardson and Warnock 2003).
The most common direct microscopic procedure relies on the use of 10–20% potassium hydroxide (KOH; Fig. 13.4), which degrades the proteinaceous components of specimens while leaving the fungal cell wall intact, thus allowing their visualization (Lease and Alexander 2011).
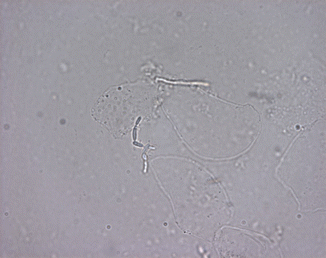
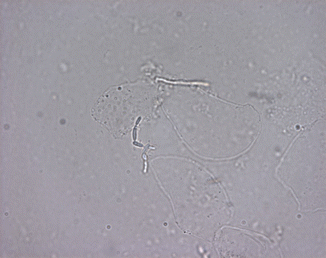
Fig. 13.4
KOH preparation demonstrating pseudohyphae of Candida spp.
The visibility of fungi within clinical specimens can be further enhanced by the addition of calcofluor white or blankophores (Willinger 2006). These are fluorophores, which are members of a group of compounds known as fluorescent brighteners, optical brighteners, or “whitening agents” and bind to β-1-3 and β-1-4 polysaccharides, such as found in cellulose and chitin. When excited with ultraviolet or violet radiation, these substances will fluoresce with an intense bluish/white color (Harrington and Hageage 2003). The high intensity of the elicited fluorescence allows rapid and reliable microscopic screening (Fig. 13.5). Optical brightener methods have been shown to be more sensitive than KOH wet mount.
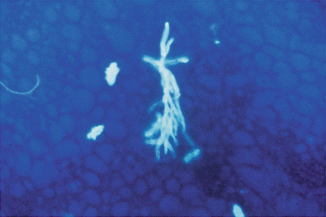
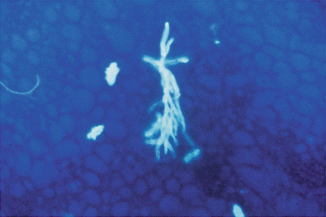
Fig. 13.5
Microscopy with an optical brightener showing dichotomously branched hyphae indicative of Aspergillus
This has also been shown in a study of respiratory samples (mostly BAL) from transplant recipients and neutropenic patients, indicating a sensitivity of 88% and a specificity of 99% for the detection of Aspergillus-like elements by blankophor in comparison with a 76% sensitivity for culture (Andreas et al. 2000; Vyzantiadis et al. 2012). The procedure is also suitable for disclosing fungi in Gram-stained microscopical mounts. Filamentous fungi like aspergilli, which stain poorly by the Gram procedure, may be unveiled on Gram-stained microscopic mounts after removal of immersion oil by subsequent blankophor staining (Ruchel and Schaffrinski 1999). In addition, optical brightener methods have been shown to be more sensitive than KOH wet mount in a number of specimen types (Chander et al. 1993). Furthermore, calcofluor or blankophor can be combined with 10–20% KOH, although it is better to pre-soften the tissue with KOH before adding the fluorescent stain (Vyzantiadis et al. 2012).
Because optical brighteners provide a rapid and sensitive method for the detection of most fungi, their use is encouraged for respiratory samples, pus, tissue samples, and fluids from sterile sites when a fluorescence microscope is available.
Similarly, lactophenol cotton blue is easy to handle and often used for the detection and identification of fungi (Fig. 13.6). Other stains are frequently used in direct microscopy, such as the India ink wet mount, which is useful for visualization of encapsulated fungi, particularly Cryptococcus neoformans. Although a negative direct examination cannot rule out fungal disease, visualization of fungal elements in specimens can often secure initial information helpful in the selection of empirical antifungal therapy (Lease and Alexander 2011).
Fig. 13.6.
Staining of Lichtheimia corymbifera using lactophenol cotton blue
Because yeast and molds can stain variably with the Gram stain, a more specific fungal stain is recommended (CLSI 2012). Microscopy may help to discern whether an infection is caused by yeast or molds (Table 13.1). The presence of pseudohyphae and optionally blastoconidia indicates the presence of yeast. Molds are most commonly seen as hyaline hyphomycetes, generally characterized by parallel cell walls, septation (cross-wall formation in hyphae), lack of pigmentation, and progressive dichotomous branching as in Aspergillus, Fusarium, or Scedosporium species (Vyzantiadis et al. 2012). Aspergillus septate hyphae have a diameter of 3–6 μm and each branch is approximately equal in width to the originating stem. Branching at 45° angles is characteristic. Because many other filamentous fungi present with a similar appearance in clinical samples on direct microscopy, one can usually only say that the infection is due to one of the agents of hyalohyphomycosis, although the balance of probability is that most infections will be due to Aspergillus (Patterson 2003).
Table 13.1.
Characteristic morphology of fungi in clinical specimens by direct microscopic examination (selection of certain fungi)
Fungal group and representatives | Microscopic morphology in clinical specimens |
---|---|
Yeasts | |
Candida spp. (except C. glabrata) | Single budding of moderately variable size |
2.5–7.5 μm in size | |
Pseudohyphae and true hyphae may be present in certain species | |
C. glabrata | Blastoconidia of uniform size (2.5–4 μm) |
No production of hyphae or pseudohyphae | |
Cryptococcus neoformans | Spherical budding yeasts of variable size |
2–15 μm in diameter | |
Capsule may be present or absent | |
No hyphae or pseudohyphae | |
Pneumocystis jirovecii | Intracystic bodies that resemble parentheses facing one another are pathognomonic |
4–6 μm in size | |
Seen on histological stains | |
Cysts often found in clusters | |
No budding | |
Best visualized in histological sections using special stains | |
Trophozoites seen on staining with giemsa or immunofluorescent stains | |
Hyaline hyphomycetes | |
Aspergillus | Hyaline, septate, dichotomously branching hyphae |
Acremonium | Uniform width (3–6 μm) |
Fusarium | Aspergillus may produce conidial heads in specimens from cavitary pulmonary lesions |
Paecilomyces | Conidiation may be visualized on histopathological examination of some of the non-Aspergillus hyphomycetes |
Scedosporium | |
Scopulariopsis | |
Trichoderma | |
Mucorales | |
Cunninghamella | Broad, thin-walled, non-septated hyphae |
Lichtheimia (formerly Absidia) | 6–25 μm wide |
Mucor | Nonparallel sides and random branches |
Rhizomucor | |
Rhizopus | |
Saksenaea | |
Dematiaceous fungi | |
Alternaria | Pigmented (brown, tan, or black) |
Bipolaris | Septate hyphae |
Curvularia | 2–6 μm wide |
Cladophialophora | |
Dimorphic fungi | |
Blastomyces dermatitidis | Large, spherical, thick-walled, budding yeast cells |
8–15 (max. 40) μm diameter | |
Typically broad-based junction between mother and daughter cells | |
Cells may appear multinucleate | |
Coccidioides immitis | Spherical, thick-walled spherules |
Vary in size (20–30 μm) | |
Mature spherules contain endospores | |
Released endospores may be mistaken for yeast | |
Hyphae and arthroconidia may be found in cavitary lesions | |
Histoplasma capsulatum | Small budding yeasts |
2–5 μm in size | |
Paracoccidoides brasiliensis | Large, multiple-budding yeasts |
Variable size | |
2–30 μm in diameter | |
Large cells are surrounded by smaller buds around the periphery (“mariner’s wheel appearance”) |
In addition, it is important to look for septate and non-septate hyphae, thus allowing to distinguish between Aspergillus spp. and members of the Mucorales. Mucoraceous molds have large (3–25 μm in diameter, average 12 μm) ribbon-like, multinucleated hyphal cells with nonparallel walls and infrequent septa. The branching is not dichotomous, irregular, and sometimes at right angles. Hyphae can appear distorted with swollen cells, or compressed, twisted, and folded (Vyzantiadis et al. 2012). Another group of molds causing tissue invasion with a distinctive appearance are the agents of phaeohyphomycosis, such as Alternaria and Curvularia. These fungi have melanin in their cell walls and appear as pigmented, septate hyphae. These are the agents of phaeohyphomycosis (Revankar 2007). The detection of fungal hyphae and/or arthrospores in skin, nail, or hair samples may indicate the presence of dermatophytes but give no special hint as to the species involved.
3. Antigen and Antibody Detection
Except for cryptococcosis, where antigen testing can reliably prove infection, serological tests mainly provide supplemental information for the diagnosis of invasive fungal infections. Antibody tests are often used in the diagnosis of endemic mycoses, which may be difficult to detect by traditional methods. In some cases, antibody tests are a supplemental test in the diagnosis of invasive candidosis, but have two major drawbacks: detection of anti-Candida antibodies fails to discriminate between disseminated and superficial infections, and antibodies are often present in colonized but uninfected patients. In order to diagnose invasive candidosis, a rise in antibody titers has to be observed in serial serum samples. However, because immunocompromised patients do not reliably produce antibodies it is rendered nearly impossible to diagnose invasive candidosis in these patients solely by antibody detection (Willinger 2006). Aspergillus antibodies are only infrequently detectable in immunocompromised patients, but are often helpful in patients with aspergilloma or allergic bronchopulmonary aspergillosis and in patients with cystic fibrosis (Kappe and Rimek 2010). Another approach to the diagnosis of fungal infections is the detection of antigens in blood or other body fluids, whether it is used as a single test or in combination with antibody detection.
(a)
Invasive Candidosis
Mannan is a Candida cell wall constituent and can be detected in the serum of patients with invasive candidosis (IC), but it is quickly degraded and cleared from the bloodstream. Furthermore, antimannan antibodies are found in patients either colonized or infected by Candida spp. However, it has been shown in patients developing IC that mannan and antimannan antibody titers complement each other (Peman and Zaragoza 2012). In fact, when these tests are used together, their sensitivity and specificity for diagnosing IC improves significantly (Marchetti et al. 2012). In practice, the two more commonly used tests are Platelia Candida Ag® and Platelia Candida Ab® assays (Bio-Rad Laboratories, Marnes-la-Coquette, France). It has been shown that detection of both antigen and antibody is superior to evaluation of each one separately as it presents a sensitivity of 83% and a specificity of 86% in IC; consequently, its use might be helpful in these patients (Mikulska et al. 2010). Nevertheless, the results of mannan antigen and antibody detection varies depending on the Candida species implicated; the tests perform best for C. albicans, C. glabrata, and C. tropicalis and worst for C. parapsilosis and C. krusei. Another important benefit of this combined antigen–antibody detection is the ability to make an earlier diagnosis than with blood cultures, in some cases. Serial determinations may be necessary. These assays can help to detect the infection early because they can be positive 6 days, on average, prior to blood cultures. The method also shows very high negative predictive value (>85%) and can be used to rule out infection (Cuenca-Estrella et al. 2012). However convincing this may be, many experts feel that these assays do not have sufficient sensitivity or specificity to influence their clinical decision-making (Kullberg et al. 2011).
Another option is the Cand-Tec latex agglutination test (Ramco Laboratories, Houston, TX, USA), which detects an antigen, not specifically defined. Though the sensitivity and specificity of this test vary among reports (Yeo and Wong 2002), it is still widely used. However, false-positive reactions due to the rheumatoid factor and renal insufficiency (Ruchel 1993) have been observed and it is generally recommended to use better-defined assays.
An indirect immunofluorescence assay to detect Candida albicans germ tube-specific antibodies (CAGTA) is commercially available as C. albicans IFA IgG® (Vircell Laboratories, Granada, Spain) and has shown an overall sensitivity of 77–89% and a specificity of 91–100% thus being useful in the diagnosis of IC in critically and noncritically ill patients (Quindos et al. 2004). In addition, Pemáan et al. (2011) found a high rate of positive results when using this test. The presence of this biomarker was the only protective factor independently associated with mortality, and positivity was not modified by either colonization or previous antifungal treatment. However, there are no recommendations to use this biomarker yet.
(b)
Cryptococcosis
The detection of cryptococcal capsular polysaccharide is one of the most valuable rapid serodiagnostic tests for fungi performed on a routine basis. The cryptococcal antigen can be detected either by the latex agglutination test or by enzyme-linked immunosorbent assay (ELISA). The test has gained widespread appeal and is suited to prove disseminated cryptococcosis (De Pauw et al. 2008). False-positive reactions have been reported in patients with disseminated trichosporonosis, Capnocytophaga canimorsus septicemia, malignancy, and positive rheumatoid factor. Another assay format is the Premier Cryptococcal Antigen enzyme immunoassay (Meridian Diagnostics, Inc., Cincinnati, OH, USA) that utilizes a polyclonal capture system and a monoclonal detection system. The Premier EIA was reported to be as sensitive as the latex agglutination system for the detection of capsular polysaccharide in serum and cerebrospinal fluid. In addition, it does not react with rheumatoid factor and gives fewer false-positive results (Yeo and Wong 2002).
(c)
Invasive Aspergillosis
The most commonly used, commercially available antigen test for Aspergillus detection is the double-sandwich ELISA test Platelia Aspergillus® (Bio-Rad Laboratories, Marnes, France), which is validated for use in serum and bronchoalveolar lavage (BAL) fluid and has been evaluated in oncohematologic patients and in receptors of hematopoietic precursors with prolonged neutropenia (Table 13.2). This test has also been evaluated in non-hematological ICU patients and patients suffering from chronic obstructive pulmonary disease (COPD) (Meersseman et al. 2008; Guinea et al. 2010; Hage et al. 2011; He et al. 2011; He et al. 2012). The Platelia Aspergillus® detects galactomannan (GM), which represents a heteropolysaccharide cell wall component of the Aspergillus cell wall and is released by Aspergillus species during hyphal growth. To optimize its diagnostic value in patients at risk of infection, it is recommended to perform two GM determinations a week, mainly in oncohematologic patients and in hematopoietic stem cell transplant recipients with prolonged neutropenia (Peman and Zaragoza 2012). Circulating GM may be detected at a median of 5–8 days before clinical signs and symptoms of IA become evident. Furthermore, its concentration corresponds to the fungal tissue burden, and may therefore be used to monitor the patient’s response to antifungal treatment (Maertens et al. 2009).
Table 13.2.
Characteristics of the galactomannan antigenemia test
Parameter | Description |
---|---|
Specimen | Serum, BAL |
Criteria for positivity | Two consecutive serum specimens with GMI ≥0.5 |
Always repeat the test before implementing therapy for IA | |
Population | Prolonged neutropenia, allogeneic SCT, ICU patients, COPD patients |
Pfeiffer et al. (2006) presented a meta-analysis of the diagnostic value of GM detection with the Platelia Aspergillus® assay, showing that for proven and probable cases of IA, the pooled (adult and pediatric) sensitivity was only 61%, whereas the overall specificity was 93%. In general, the negative predictive value (NPV) and the specificity are excellent (>95%), suggesting that the assay can be used to rule out the diagnosis of IA. However, false positive and negative results of GM have been described in certain patient groups (Table 13.3) by various authors (Wheat and Walsh 2008; Hage et al. 2011; Peman and Zaragoza 2012). Solely testing for antigenemia does not replace other tests for IA. To maximize sensitivity, testing should precede empiric antifungal therapy and positive results should be confirmed on a new specimen (Wheat 2003). Generally lower sensitivity rates have been obtained from pediatric patients than adult patients in studies evaluating the utility of GM antigenemia test in early diagnosis of IA (Oz and Kiraz 2011). When using this test it has to be considered that results may be falsely positive or negative and have to be correlated with clinical and laboratory findings.
Table 13.3.
Causes for false-positivity or cross-reactivity in the galactomannan antigenemia test
Patient groups with false-positive results | False-positivity caused by galactomannan contamination | Cross-reactivity caused by similar cell wall galactomannan |
---|---|---|
Infants with intestinal colonization by Bifidobacterium spp. | Piperacillin-tazobactam | Penicillium spp. |
Amoxicillin-clavulanate | Alternaria spp. | |
Chronic graft- versus-host disease | Other beta-lactam antibiotics | Paecilomyces spp. |
Plasmalyte (sodium gluconate) | Cryptococcus spp. | |
People following diets rich in soy protein and other foods | Other intravenous hydration or nutrition fluids containing sodium gluconate | Histoplasma capsulatum |
Geotrichum | ||
Echinocandins | Neosartoria | |
Chronic graft- versus-host disease | Lichtheimia ramosa | |
Diets rich in soy protein and other foods |
Antigen has been detected in body fluids other than sera. The detection of antigen in BAL fluid was described in various studies, indicating the presence of pulmonary aspergillosis. A meta-analysis evaluating the accuracy of BAL galactomannan determined the sensitivity to be 90% and specificity 94% (Guo et al. 2010). A positive result increased the probability of having IA by about sixfold, whereas a negative result decreased the probability to 1%. Whereas the sensitivity remained unchanged, at about 85%, the specificity increased from 89% to 94% by using a cut-off of 1.0 instead of 0.5. BAL was judged to be more useful than serum, by comparison with results of another meta-analysis (Pfeiffer et al. 2006).
Hage et al. (2011) state when using BAL, positive results caused by airway colonization may complicate the use of the test for diagnosis. As shown, results may be false-positive in patients colonized with Aspergillus, Penicillium, and Paecilomyces (Clancy et al. 2007; Husain et al. 2007). When combined results of those studies were analyzed, sensitivity was unchanged but specificity increased using a cut-off of 1.0 (Muller et al. 2002). Results in colonized specimens were at least 1.0 in 8 out of 12 cases (Husain et al. 2008). However, BAL galactomannan was negative in hematology patients who were colonized with Aspergillus species (Bergeron et al. 2010). As colonization is undesirable in solid organ transplant or hematology patients at high risk of IA, results attributed to colonization should not be disregarded, but rather prompt additional investigation to exclude invasive disease or to assess the effectiveness of antifungal prophylaxis or therapy, and follow-up evaluation for subsequent invasive disease (Hage et al. 2011). All in all, GM detection in BAL fluid seems to be useful in establishing or excluding the diagnosis of IA in ICU patients (Hage et al. 2011).
In addition, the test might allow the detection of GM in other specimens such as cerebrospinal fluid, urine, and homogenates/extracts of tissue specimens. Few data exist on the performance of the galactomannan assay for homogenized tissue specimens, nevertheless the data are promising (Lackner and Lass-Flörl 2013).
Monitoring for antigen clearance or rebound may provide useful information for assessing the effectiveness of therapy. Declining levels in patients responding to therapy and rising concentrations in those with fatal outcomes have been observed by several authors (Bretagne et al. 1997; Maertens et al. 2001). Thus, failure of antigenemia to decline may suggest treatment failure and support consideration of modifying the therapy.
Studies on patients who have undergone solid organ transplantation report sensitivity ranging from 67% to 100% and specificity from 40% to 98% (Clancy et al. 2007; Husain et al. 2007; Pasqualotto et al. 2010). Detection of antigen in BAL was more sensitive than detection of antigen in serum or isolation of Aspergillus from the BAL or lung biopsy, as highlighted in an evaluation of proven cases in hematology patients (Maertens et al. 2010). In that study, the sensitivity was 100% for BAL antigen, 55% for serum antigen, 62% for culture, and 74% for microscopy. In organ transplant recipients, sensitivity was 82% for antigen detection compared to 73% for culture and/or microscopy (Husain et al. 2008).
Recently Thornton et al. (2012) described a new promising diagnostic method for the detection of Aspergillus in patients suffering from hematological malignancies. The technology is based on the detection of Aspergillus-specific JF5 by MabJF5 monoclonal antibodies. The JF5 is an extracellular glycoprotein that is exclusively secreted during active growth of the fungus and represents a surrogate marker of Aspergillus infection. Evaluating exclusively the activity of Aspergillus rather than the antigen presents a major advantage compared with the GM test and, at the same time, limits the risk of cross-reactivity or a false-positive result due to contaminated materials because the JF5 test only detects germinating conidia that represent a potential infectious risk. The technique has been incorporated into an immuno-chromatographic lateral-flow device (‘point of care’ diagnostic tool), which is easy to use. The test aims to detect invasive pulmonary aspergillosis using blood or/and serum samples from patients in only 15 min. However, further studies are needed to prove the test’s performance in a clinical setting (Lackner and Lass-Flörl 2013).
4. (1,3)-β-d-Glucan as a Marker for Invasive Fungal Infection
(1,3)-β-d-Glucan (BDG) is a component of the cell walls of many fungal organisms; its presence can be detected in sera when observing activation of the factor G of the horseshoe crab coagulation cascade (Bellanger et al. 2011). The presence of BDG in serum has been shown to be a reliable marker of invasive fungal infection (Karageorgopoulos et al. 2011), and it has recently been added to the EORTC/MSG guidelines as a biological criterion for invasive fungal diseases other than zygomycosis and cryptococcosis (De Pauw et al. 2008). It has also been proposed as a noninvasive marker for pneumocystosis (Bellanger et al. 2011). However, one has to be aware that this test does not detect cryptococcosis and fungal colonization or superficial infections.
The contribution of BDG to IFI screening has mainly been investigated for patients with hematological malignancies. More recently, studies have reported the contribution of the BDG assay for detecting invasive candidiasis in patients in surgical intensive care units (Mohr et al. 2011; Ostrosky-Zeichner et al. 2011).
Karageorgopoulos et al. (2011) recently presented a meta-analysis including 2979 patients (594 with proven or probable IFIs) showing a pooled sensitivity of 76.8% and a specificity of 85.3%. However, marked statistical heterogeneity was noted. BDG has good diagnostic accuracy for distinguishing proven or probable IFIs from no IFIs and can be useful in clinical practice, if implemented in the proper setting and interpreted after consideration of its limitations. In addition, the detection of BDG precedes the appearance of symptoms, radiologic signs, and the empirical antifungal treatment in some patients (Peman and Zaragoza 2012).
5. Culturing Techniques
Culture remains one of the key methods for diagnosing fungal infection. Though often slow, sometimes insensitive, and sometimes confusing with respect to contamination, culture may yield the specific etiological agent and may allow susceptibility testing to be performed. Proper collection and transportation of the specimen is essential. In particular, sterile materials are important for diagnosis of IFI.
Blood cultures (BC) are the first-line test and currently considered the “gold standard” in the event of any suspected case of systemic mycosis (Ostrosky-Zeichner 2012). Several commercial blood culture systems are available.
Lysis centrifugation has been considered the “gold standard” for recovering pathogenic yeasts, as well as thermally dimorphic fungi from blood (Willinger 2006). In particular, dimorphic molds can be most reliably and rapidly recovered in the lysis centrifugation system. Lysis centrifugation involves incorporation of a tube containing an anticoagulant and a lytic agent and exploits the possibility that white blood cell lysis releases viable fungal cells. However, it is time consuming and prone to contamination (Berenguer et al. 1993). When older BC systems are used as comparators, lysis centrifugation procedures show higher efficacy, whereas current automated systems have improved the sensitivity of blood cultures for detecting microorganisms (Cuenca-Estrella et al. 2012). In comparison to current automated blood culture systems, in recent studies lysis centrifugation does not appear more sensitive for the majority of invasive fungi (Morrell et al. 1996; Arendrup et al. 2010).
Of the automated systems, there are two that are widely used: the Bactec System (BD Diagnostic System, Sparks, MD) and the BacT/Alert System (bioMérieux, Marcy l’Etoile, France). Studies have documented that these systems match the performance of lysis centrifugation methods for the detection of yeast. The Bactec system proposes a specifically formulated medium for the isolation of fungi, called Mycosis IC/F medium. This medium is a brain–heart broth enriched with sucrose, chloramphenicol, and tobramycin to inhibit bacterial growth, and a lysis agent, saponin. The incubation period recommended by the manufacturers for Bactec Mycosis IC/F and BacT/Alert FA vials is 14 and 5 days, respectively. In various studies, the vast majority of the Candida species were detected in 5 days (Meyer et al. 2004; Ericson et al. 2012). The main reason for 14 days of incubation for Bactec Mycosis IC/F vials is to detect the growth of filamentous fungi, which may take longer.
In 2012, recommendations concerning diagnostic procedures for detection of Candida diseases were published by the ESCMID Fungal Infection Study Group (Cuenca-Estrella et al. 2012). Concerning candidemia, the number of BC recommended in a single session is 3 (2–4), with a total volume varying according to the age of the patient: 40–60 ml for adults, 2–4 ml for children under 2 kg, 6 ml between 2 and 12 kg, and 20 ml between 12 and 36 kg. The timing for obtaining the BC is one right after the other from different sites, and venipuncture remains the technique of choice. A BC set comprises 60 ml blood for adults obtained in a single session within a 30-min period and divided into 10-ml aliquots among three aerobic and three anaerobic bottles. The frequency recommended is daily when candidemia is suspected, and the incubation period must be at least 5 days.
When these recommendations have been followed, the sensitivity of BC to detect Candida is 50–75% although lower sensitivity rates in neutropenic patients and those undergoing antifungal treatment have been reported (Arendrup et al. 2008, 2011). It should be noted that fungal pathology in direct Gram stain from positive BC flasks can provide a reasonably reliable basis for discrimination between C. albicans and non- albicans species (Harrington et al. 2007).
Despite the advances in blood culture technology, the recovery of fungi from blood remains an insensitive marker for IFI. Filamentous fungi will be detected to a much lesser extent than yeasts. For IA, BCs represent a limited diagnostic tool because Aspergillus fungaemia is rarely encountered, even in the setting of disseminated disease (Duthie and Denning 1995; Denning 1998; Girmenia et al. 2001). Mainly, A. terreus has been described as being detected by blood cultures, other Aspergillus species very rarely. However, recently it has been described (Rosa et al. 2011) that BACTEC automated systems may allow detection of A. fumigatus, A. flavus, and A. terreus. In this study, BACTEC Plus Aerobic/F vials seemed to be advantageous for Aspergillus detection in blood samples collected from patients under antifungal treatment.
Culture is highly sensitive (98%) in patients with Cryptococcus meningitis (Denning et al. 2003). However, in central nervous system aspergillosis or candidiasis, cerebrospinal fluid (CSF) cultures are less sensitive (Arendrup et al. 2012). For Candida meningitis, 80% of CSF cultures have been described as positive (Sanchez-Portocarrero et al. 2000). All yeasts and molds obtained from sterile sites, including blood and continuous ambulatory peritoneal dialysis (CAPD) fluids, and intravenous-line tips should be identified to species level. This is also valid for bronchoscopically gained specimens. Treatment of clinically obvious or severe cases should not be delayed for culture results, although treatment may need to be altered according to the species. The presence or absence of fungal elements on microscopy is not always predictive of positive culture results, and if a clinician is faced with unexpectedly negative results, investigations should be repeated while alternative diagnoses are considered (Moriarty et al. 2012).
However, the interpretation of results obtained from a culture of the respiratory tract specimens is difficult because of the possible transient colonization by yeasts, on the one hand, and the ubiquitous nature of airborne conidia and the risk of accidental contamination with molds, on the other hand. It has been observed that the cultivation of Aspergillus spp. from normally sterile sites and the presence of Aspergillus in respiratory samples from immunocompromised children at risk of IA are highly indicative of infection (Muller et al. 2002). Detection of Aspergillus species in BAL fluid is highly suspicious for invasive disease in febrile granulocytopenic patients with new pulmonal infiltrates. However, the absence of hyphal elements or a negative culture does not exclude the diagnosis.
6. Identification of Cultured Fungi
Yeasts are identified by their assimilation pattern and their microscopic morphology, and molds by their macroscopic and microscopic morphology. Due to the slow growth of some species/isolates this can take days to weeks. As for Candida spp., the use of chromogenic media and test kits allows rapid identification and can accelerate the otherwise slow identification of yeasts. Identification of the most common yeasts can be performed immediately when visible growth is observed (Fig. 13.7). Chromagar Candida (Becton Dickinson, Franklin Lakes, NJ, USA) and Candida ID Agar (bioMérieux, Marcy l’Etoile, France) have been shown to allow easier differentiation of Candida species in mixed yeast populations than the traditional Sabouraud Glucose agar. Moreover, more rapid identification of Candida species can be achieved on these media than with conventional test kits. These media reduce the need for subculture and further biochemical tests and considerably simplify and shorten the identification procedure, mainly for C. albicans, C. tropicalis, and C. krusei (Willinger and Manafi 1999; Fricker-Hidalgo et al. 2001; Willinger et al. 2001). However, C. glabrata the second most common Candida species cannot be identified reliably on chromogenic media.
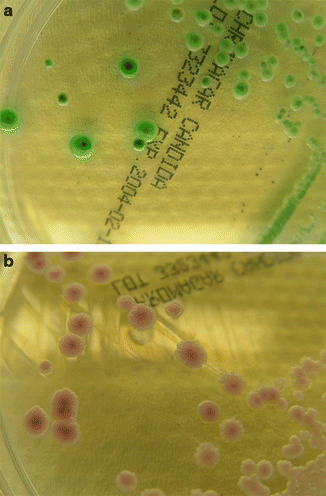
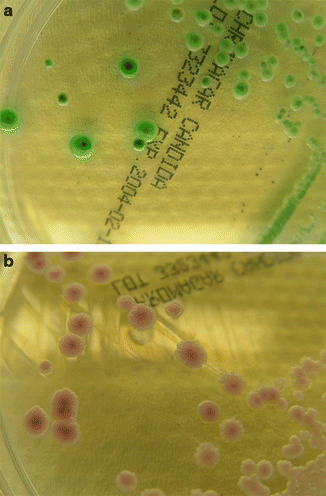
Fig. 13.7.
Pigmentation of various Candida species on ChromagarCandida® : (a) C. albicans and (b) C. parapsilosis)
Identifying filamentous fungi can be much more cumbersome. Generally, macroscopic and microscopic morphology is the key to identification (de Hoog et al. 2009). Macroscopic examination of the colonies can reveal important characteristics concerning color, texture, exudates, pigments, specific structures, growth rate and growth zones, and aerial (the hyphae projecting above the medium surface) and submerged or vegetative mycelium (the portion that penetrates the medium in order to secure the colony and absorb nutrients). The color of the reverse of the colony must be recorded along with any pigment that diffuses into the medium. In addition, microscopic elements have to be evaluated for identification. The production of characteristic conidia and hyphae enables the identification of a large number of molds, at least to the genus level (Vyzantiadis et al. 2012). Standard references including identification algorithms are available (de Hoog et al. 2009) but, despite this, morphology-based identification of molds requires a high degree of experience.
Commercially available biochemical test systems identify most of the commonly isolated species of yeast accurately but may result in no identification or misidentification of more unusual isolates (Freydiere et al. 2001). Furthermore, samples for these tests must be incubated for 1–3 days before results are obtained. Identification of molds may also be cumbersome and tedious because it is mainly based on morphology. As an alternative to the conventional identification schemes, proteomic profiling by mass spectral analysis was recently evaluated for use in species differentiation of a variety of microorganisms. Matrix-assisted laser desorption ionization–time-of-flight mass spectrometry (MALDI-TOF) is emerging as a rapid and accurate tool for identifying pathogens, not only for bacteria but also for molds and yeast species. This technology generates characteristic mass spectral fingerprints that are unique signatures for each microorganism and are thus ideal for an accurate microbial identification at the genus and species levels and have the potential to be used for strain typing and identification (Stevenson et al. 2010; Croxatto et al. 2012). Furthermore, molecular tools can aid in the identification of cultured fungi (see below)
7. Mass-Spectrometry-Based Identification of Fungal Pathogens
This technique probably presents the major paradigm change of the past decades in the way in which species are determined in microbiology because it allows the identification of microorganisms within a few minutes. Currently, there are four commercial systems available: the MALDI Biotyper (Bruker Daltonics, Bremen, Germany), the AXIMA@SARAMIS database (AnagnosTec, Potsdam, Germany and Shimadzu, Duisburg, Germany), and the Andromas (Andromas, Paris, France) and VITEK MS systems (bioMérieux, Marcy l’Etoile, France). Among fungi, ascomycetous and basidiomycetous yeasts including Candida, Pichia, and Cryptococcus genera are most easily processed and analyzed (Bader 2013).
Recent studies showed that far more than 90% of clinical Candida isolates from different species could be correctly identified by MALDI-TOF MS (Marklein et al. 2009; van Veen et al. 2010). Furthermore, closely related yeast species that cannot be discriminated with common biochemical methods, such as Candida ortho-/meta-/parapsilosis, Candida glabrata/bracarensis/nivariensis, Candida albicans/dubliniensis, Candida haemulonii group I and II complexes, or the phenotypically similar species Candida palmioleophila, Candida famata, and Candida guilliermondii, can be resolved without difficulty by MALDI-TOF MS (Bader 2013).Stay updated, free articles. Join our Telegram channel
![]()
Full access? Get Clinical Tree
![]()
![]()
