Pulmonary Embolism: Introduction
Approximately 100,000 patients in the United States die each year directly as a consequence of acute pulmonary embolism (PE), with another 100,000 deaths occurring in patients with concomitant disease in whom PE contributes significantly to their demise.1,2 Three-month mortality in unselected patients with acute PE is as high as 15%.3 Although a number of patients die of comorbidities that predispose them to the thromboembolic event, a substantial number of patients die from PE within 1 hour of presentation, often before the diagnosis can be confirmed and therapy initiated, or because the diagnosis was overlooked.4 Autopsy studies have repeatedly documented the high frequency with which PE has gone unsuspected and undetected.5 Despite advances in diagnostic imaging tests and therapeutic interventions, PE remains underdiagnosed and prophylaxis continues to be dramatically underused.
Over the past decade, a number of valuable insights into the natural history of venous thrombosis and PE have enhanced our diagnostic and therapeutic approaches. One such insight is the awareness that patients hospitalized for medical problems face a thromboembolic risk similar to that of their surgical counterparts. Another is an understanding of the substantial thromboembolic recurrence risk among patients with idiopathic or unprovoked venous thrombosis.6 Yet another insight is the awareness that the presence of right ventricular (RV) dysfunction in the setting of PE may be associated with an increased risk of adverse consequences, including subsequent cardiovascular collapse and death.7 Anticoagulation with heparin as a “bridge” to warfarin is still considered the standard treatment for PE. The spectrum of anticoagulant drugs has been expanded recently. Low-molecular-weight heparins (LMWHs) have been shown to be effective and safe for both treatment and for prevention of venous thromboembolism (VTE), particularly in hospitalized medical patients. Fondaparinux, a new pentasaccharide, is very effective in a fixed low dose in preventing VTE after orthopedic and abdominal surgery and has been demonstrated in clinical trials to be as effective as LMWH and unfractionated heparin (UFH) for the initial treatment of patients with deep venous thrombosis (DVT) and PE. A new generation of oral direct thrombin inhibitors and factor Xa inhibitors appears to be on the horizon.
Risk Factors and Pathogenesis of Venous Thromboembolism
In 1856, Virchow proposed his triad of factors leading to intravascular coagulation, including stasis, vessel wall injury, and hypercoagulability. Risk factors for DVT are based on these processes (Table 72–1). The overwhelming majority of emboli originate from the deep veins of the lower extremities, although any venous bed can be involved. Although thrombi may form at any point along the vein wall, most originate in valve pockets. The veins of the calf are the most common site of origin, with subsequent extension of the clot prior to embolization.8 Eventually, the thrombus may expand to fill the vessel entirely, with both retrograde and proximal extension. If embolization does not occur, the thrombosis can partially or completely resolve via three mechanisms: recanalization, organization, and lysis. Postthrombotic syndrome occurs in 20% to 50% of patients and involves chronic pain, swelling, edema, and skin changes, which reduce quality of life and incur significant health care costs.9
Acquired factors |
Age older than 40 y |
History of venous thromboembolism |
Prior major surgical procedure |
Trauma |
Hip fracture |
Immobilization or paralysis |
Venous stasis |
Varicose veins |
Congestive heart failure |
Myocardial infarction |
Obesity |
Pregnancy or postpartum period |
Oral contraceptive therapy |
Cerebrovascular accident |
Malignancy |
Severe thrombocythemia |
Paroxysmal nocturnal hemoglobinuria |
Antiphospholipid antibody syndrome (including lupus anticoagulant) |
Inherited factors |
Antithrombin III deficiency |
Factor V Leiden (activated protein C resistance) |
Prothrombin gene (G20210A) defect |
Protein C deficiency |
Protein S deficiency |
Dysfibrinogenemia |
Disorders of plasminogen |
Hyperhomocysteinemia |
Frequently, more than one risk factor for venous thrombosis is present; knowledge of these risk factors provides the rationale for both prophylaxis and clinical suspicion. Comorbidities enhance the risk of VTE. In the DVT FREE prospective registry of 5451 patients with ultrasound-confirmed DVT, the most common comorbidities were arterial hypertension (50%), surgery within 3 months (38%), immobility within 30 days (34%), cancer (32%), and obesity (27%).10
Although studies to date have yielded inconsistent results, long automobile or airplane trips appear to be risk factors for VTE. The proportion of subjects who develop acute PE during or after airplane travel appears to be associated with other thrombotic risk factors, such as the presence of the factor V Leiden mutation or the use of oral contraceptive agents, and correlates with the flight distance. The risk of PE significantly increases with a flight distance of greater than 3107 miles (5000 km) or a duration longer than 8 hours.11
Obesity merits further investigation because recent studies implicate obesity as a risk factor for VTE, particularly in the United States, where obesity represents a major health issue.12,13 The Nurses’ Health Study explored risk factors for PE in women and found that a body mass index of 29 kg/m2 or greater was an independent risk factor.14 The Framingham Study has confirmed that obesity is a risk factor for PE, particularly in women.15 In addition to increased venous stasis, obesity may also increase the risk for VTE as a consequence of elevated plasma levels of certain clotting factors, such as fibrinogen, factor VII, and plasminogen activator inhibitor-1, and as a result of platelet activation caused by enhanced lipid peroxidation.16-19
An abundance of literature documents that the risk of VTE increases with age, with a relative risk for those 70 years of age approximately 25-fold greater than the risk for those 20 to 29 years of age.20 Age also appears to increase mortality because of PE, and PE is suspected less commonly before death in elderly patients.4,21
Prior VTE substantially increases the risk of subsequent events. Surgical patients with a previous history of VTE who do not receive prophylaxis develop postoperative DVT in more than 50% of cases.22 Surgery itself significantly enhances the risk. Patients who are undergoing general surgery without additional risk factors develop DVT in nearly 20% of cases if neither pharmacologic nor mechanical prophylaxis is applied.23 Patients who are undergoing lower extremity orthopedic procedures such as total hip or total knee replacement develop DVT in more than 50% of cases.24 Prophylactic anticoagulation may be initiated either before surgery or shortly thereafter to prevent the development of intraoperative and early postoperative thrombosis. These orthopedic settings have been comprehensively investigated, prompted by the increasing use of LMWH. Spinal surgery, pelvic surgery, and neurosurgery place patients at a particularly high risk for VTE.
Trauma, particularly of the lower extremities and pelvis, heightens the risk of DVT. PE has been identified at autopsy in as many as 60% of patients with lower extremity fractures, and mortality has been attributed to PE in as many as 50% of patients dying after hip fracture.25,26 The incidence of VTE increases with time after the traumatic event. Autopsy-confirmed PE in patients surviving for less than 24 hours after trauma has been demonstrated in 3.3%, increasing to 5.5% in patients surviving up to 7 days. PEs occurred in 18.6% of patients surviving for a longer period.27
Upper extremity DVT has become more important because of an increasing use of pacemakers; implantable defibrillators; and long-term, indwelling, central venous catheters.28 Symptomatic PE may originate from upper extremity thrombi, although this appears much less common than embolization from lower extremity DVT. Upper extremity DVT poses the risk of superior vena cava syndrome and loss of vascular access.29 Effort-related, upper extremity axillosubclavian thrombosis (Paget-von Schroetter syndrome) may occur spontaneously or be associated with an underlying thrombophilic tendency, and may result in significant, long-term functional impairment.30
Epidemiologic analyses, as well as autopsy data, suggest that patients with cardiac disease are predisposed to VTE.4,31 Although myocardial infarction (MI) without anticoagulation is associated with a significant incidence of DVT, more recent therapeutic strategies for acute coronary syndromes have had a beneficial impact.32 Large, placebo-controlled acute MI trials indicate that the use of thrombolytic therapy reduces the incidence of VTE.33
Cancer clearly augments the risk of VTE, although the precise pathogenesis of thromboembolism in cancer is not well understood. Numerous mechanisms, including intrinsic tumor procoagulant activity and extrinsic factors such as chemotherapeutic agents and indwelling access catheters, contribute to this process. The thrombophilic tendency associated with cancer is often amplified by clinical factors such as patient weakness and immobility. An analysis based on data from PIOPED (Prospective Investigation of Pulmonary Embolism Diagnosis) found that of 399 patients with PE, 73 (18.3%) had cancer.4 Pancreatic, lung, gastric, genitourinary tract, and breast malignancies are associated with a particularly high risk of DVT and PE. About half of all cancer patients and approximately 90% of those with metastases exhibit abnormalities of one or more coagulation parameters.34,35 After the administration of various chemotherapeutic agents, changes in the levels of coagulation factors, suppression of anticoagulant and fibrinolytic activity, and direct endothelial damage have been documented clinically and experimentally.36 Hormonal therapy, particularly tamoxifen in breast cancer adjuvant therapy, is also associated with an increased risk of thromboembolism, particularly when combined with chemotherapy.37 Neutropenia and sepsis, which often accompany chemotherapeutic regimens, often necessitate hospitalization and bedrest, which contribute further to the risk of VTE. A subsequent malignancy has been reported to occur within 2 to 3 years in approximately 5% to 10% of patients presenting with idiopathic venous thrombosis.38 Although an aggressive search for cancer does not appear to be warranted in patients presenting with idiopathic DVT, recent data suggests that a limited approach (abdominal/pelvic computed tomography [CT], mammography, sputum cytology) may be cost effective.39
Pregnancy and the postpartum period are the most common settings in which women younger than age 40 years acquire thromboembolic disease. Venous thrombosis develops in these settings three to six times more often than in age-matched women not on oral contraceptives.40 Although DVT appears to be more common in the third trimester and postpartum than before delivery, the risk is considerable throughout pregnancy.41 Cesarean section further augments the risk. Oral contraceptives are associated with an increased relative risk of VTE, although the absolute risk (~one to three cases per 10,000 woman-years) remains small.40 Third-generation agents (agents containing desogestrel or gestodene as the progestogen component) appear to cause acquired resistance to activated protein C and double the risk of VTE.42,43 Oral contraceptive use should be avoided by women with protein C, protein S, and antithrombin III deficiency, as well as those who are homozygous carriers of the factor V Leiden mutation. Results from a clinical trial evaluating hormone replacement therapy indicated that such therapy increased the incidence of VTE in women 45 to 64 years of age. The best available evidence suggests a two- to four-fold increased relative risk of VTE among oral hormone replacement therapy users compared with nonusers.40 The risk of VTE also appears to be highest during the first year of exposure to hormone replacement.44 It has not been clearly established that previous use increases the risk.45 Although such therapy is associated with quality-of-life benefits in women who require postmenopausal symptom control, physicians must balance this benefit against the risk of VTE, cardiovascular disease, and breast cancer before prescribing hormone replacement therapy. Whether routine screening should be performed before the initiation of oral contraceptive agents or hormone replacement therapy remains controversial.46 Given the low absolute risk, especially among users of oral contraceptive agents, the general consensus is that such an approach would not be cost effective.47
Antiphospholipid antibodies represent a diverse family of immunoglobulins that includes anticardiolipin antibodies and the lupus anticoagulant and that are targeted against anionic surfaces such as β2-glycoprotein I and prothrombin. Antiphospholipid antibodies, notably the lupus anticoagulant and high-titre anticardiolipin antibodies, are associated with both arterial and venous thrombi.48
Inherited thrombophilias result in variable degrees of VTE risk.49,50 Antithrombin III deficiency was first described in 1965 and was the first inherited trait associated with thrombophilia. Functional and quantitative abnormalities of protein C and protein S were subsequently described. The factor V Leiden mutation, a single base mutation (substitution of A for G at position 506), is a far more common genetic polymorphism associated with activated protein C resistance. It is present in approximately 4% to 6% of European populations and is less common among those of Asian, Indian, or African descent.51,52 The relative risk of a first idiopathic DVT among men heterozygous for the mutation is three- to seven-fold higher than that of those not affected.52 This genetic mutation is also a risk factor for recurrent pregnancy loss, probably because of placental thrombosis.53 Oral contraceptive use in patients with heterozygous factor V Leiden mutation is associated with a 10-fold higher risk of VTE.54
Another less frequent thrombophilic mutation has been identified in the 3 untranslated region of the prothrombin gene (substitution of A for G at position 20210).55 This mutant allele is present in 2% to 4% of the general population and causes increased levels of prothrombin. This prothrombin gene defect increases the risk of DVT by a factor of 2.7 to 3.8.55,56 It appears that carriers of both factor V Leiden and the prothrombin G20210A defect have an increased risk of recurrent DVT after a first episode and are candidates for lifelong anticoagulation.57
Homocysteine has potential thrombogenic effects, including injury to vascular endothelium and antagonism of the synthesis and function of nitric oxide.58 Coexisting hyperhomocysteinemia has been shown to increase the risk for thrombosis in patients with factor V Leiden.59 However, the thermolabile methylenetetrahydrofolate reductase gene variant is not independently associated with thrombosis, emphasizing that the precise role of homocysteine in venous thrombosis is unclear. Thus, interactions between the genetic factors (defects in enzymes) that control homocysteine metabolism and nutritional factors (folate, vitamin B6, and vitamin B12 deficiencies) that affect homocysteine metabolism warrant additional investigation with regard to VTE.60
Recently, elevated levels of clotting factors VII, VIII, IX, XI, and XII have been associated with an increased risk for venous thrombosis. In particular, elevated factor VIIIc levels have been demonstrated to be a strong and independent risk factor for both acute and recurrent venous thrombosis.61,62
Pathophysiology of Acute Pulmonary Embolism
The effect of PE on oxygenation and hemodynamics depends on the extent of obstruction of the pulmonary vascular bed and the severity of underlying cardiopulmonary disease.7,63-65 Hypoxemia develops in the preponderance of patients with PE and has been attributed to various mechanisms, including intrapulmonary or intracardiac right-to-left shunting, elevated alveolar dead space, ventilation–perfusion (V/Q) inequality, and decreases in the mixed venous O2 level, thereby magnifying the effect of the normal venous admixture. The two latter mechanisms are proposed to account for the majority of hypoxemia and hypocarbia associated with acute embolism. Shunt may occur as a consequence of atelectasis related to loss of surfactant, alveolar hemorrhage, or bronchoconstriction related to regional areas of hypocarbia. Hypoxemia leads to an increase in sympathetic tone with systemic vasoconstriction and may actually increase venous return with augmentation of stroke volume, at least initially, if there is no significant underlying cardiac or pulmonary pathology already present.
The hemodynamic effects of embolism are related to three factors: the degree of reduction of the cross-sectional area of the pulmonary vascular bed, the preexisting status of the cardiopulmonary system, and the physiologic consequences of both hypoxic and neurohumorally mediated vasoconstriction.7,63-65 Obstruction of the pulmonary vascular bed by embolism acutely increases the workload on the RV, a chamber ill equipped to deal with high-pressure load. In patients without preexisting cardiopulmonary disease, obstruction of less than 20% of the pulmonary vascular bed results in a number of compensatory events that minimize adverse hemodynamic consequences. Recruitment and distension of pulmonary vessels occur, resulting in a normal or near-normal pulmonary artery pressure and pulmonary vascular resistance; cardiac output is maintained by increases in the RV stroke volume and increases in the heart rate. As the degree of pulmonary vascular obstruction exceeds 30% to 40%, increases in pulmonary artery pressure and modest increases in right atrial pressure occur. The Frank-Starling mechanism maintains RV stroke work and cardiac output. When the degree of pulmonary artery obstruction exceeds 50% to 60%, compensatory mechanisms are overcome, cardiac output begins to decrease, and right atrial pressure increases dramatically. With acute obstruction beyond this amount, the right heart dilates, RV wall tension increases, RV ischemia may develop, the cardiac output decreases, and systemic hypotension develops. In patients without prior cardiopulmonary disease, the maximal mean pulmonary artery pressure capable of being generated by the RV appears to be 40 mm Hg. The correlation between the extent of pulmonary vascular obstruction and the pulmonary vascular resistance appears to be hyperbolic, reflecting at its lower end the expansible nature of the pulmonary vascular bed, and at its upper end, the precipitous decline in cardiac output that may occur as the RV fails.66,67
The hemodynamic response to acute PE in patients with preexisting cardiopulmonary disease may be considerably different.64 Patients with prior cardiopulmonary disease demonstrate degrees of pulmonary hypertension that are disproportionate to the degree of pulmonary vascular obstruction. As a result, severe pulmonary hypertension may develop in response to a relatively small reduction in pulmonary artery cross-sectional area. Thus, evidence of RV hypertrophy (rather than RV dilatation) associated with a mean pulmonary artery pressure in excess of 40 mm Hg should suggest an element of chronic pulmonary hypertension resulting from a potentially diverse group of etiologic possibilities (eg, chronic thromboembolic pulmonary hypertension [CTEPH], left ventricular [LV] failure, valvular disease, right-to-left cardiac shunts).
Diagnosis of Deep Venous Thrombosis and Pulmonary Embolism
Erythema, warmth, pain, swelling, and tenderness are not specific for DVT but suggest the need for further evaluation. PE must always be considered when unexplained dyspnea is present. Pleuritic chest pain and hemoptysis are also common in patients with PE. Coughing may be present, and although it is sometimes caused by PE, it more commonly occurs with bronchitis or pneumonia. Anxiety and lightheadedness are symptoms that may be caused by PE but may also be caused by a number of other entities that result in hypoxemia or hypotension. Severe dyspnea and syncope are the principal symptoms that may suggest massive, life-threatening PE.68,69 Tachypnea and tachycardia are the most common signs of PE, but they are also nonspecific. A pleural rub or accentuated pulmonic component of the second heart sound may suggest PE but can also be explained by other disorders. With embolism of sufficient magnitude to cause RV dysfunction, a murmur of tricuspid regurgitation, systemic hypotension, or jugular venous distension might be present.
A major advance in the diagnostic approach to both venous thrombosis and PE has been a transition from a technique-oriented approach to one that uses Bayesian analysis. In doing so, the pretest probability of the disease, calculated independently of a particular test result through either empiric means or through a standardized prediction rule, is calculated. This pretest probability aids in the selection and interpretation of further diagnostic tests to create a posttest probability of the disease. This posttest probability can then be used as a basis for clinical decision making. For PE, three such scores have been developed and validated. Wells and coworkers70 prospectively tested a rapid seven-item bedside assessment to estimate the clinical pretest probability for PE. An alternative scoring system, the Geneva score, involved seven variables and required gas exchange and radiographic information.71 Recently, a revised Geneva score requiring eight clinical variables without gas exchange or radiographic information was validated and published.72 Although such scoring systems have not proven to be more accurate than implicit assessment, they do provide a means of standardization that compensates for variability in physician experience and judgment.
PE may mimic a large spectrum of diseases. The most common differential diagnoses are pneumonia, musculoskeletal pain, pneumothorax, costochondritis, congestive heart failure, chronic lung disease, asthma, acute MI, aortic dissection, pericarditis, and anxiety states.
The plasma d-dimer is a specific derivative of cross-linked fibrin. Measurement of circulating plasma d-dimer has been comprehensively evaluated as a diagnostic test for acute VTE.73 A normal enzyme-linked immunosorbent assay (ELISA) result is highly sensitive in excluding PE and DVT. When an ELISA d-dimer level is below an established cutoff level, the sensitivity and negative predictive value for VTE are 98% or above.73 In a prospective analysis, 76 of 79 (96%) patients with high-probability V/Q scans had elevated d-dimer levels.74 Thus, increased levels of cross-linked fibrin degradation products are an indirect but suggestive marker of intravascular thrombosis, indicating endogenous fibrinolysis. An increased d-dimer level is nonspecific for PE and may be seen with advancing age and in patients with various conditions, including infections and other inflammatory states, cancer, MI, the postoperative state, and the second and third trimesters of pregnancy.
It is important to recognize that the usefulness of d-dimer testing in inpatients is limited as a result of comorbidities in this population that elevate d-dimer levels.
Although hypoxemia is common in acute PE, some patients, particularly young individuals without underlying cardiopulmonary disease, may have a normal PaO2 (arterial oxygen partial pressure). In a retrospective study of hospitalized patients with PE, the PaO2 was greater than 80 mm Hg in 29% of patients who were younger than 40 years compared with 3% in the older group.68 However, the alveolar–arterial (A–a) difference was elevated in all patients. An important tenet should be that unexplained hypoxemia, particularly in the setting of risk factors for DVT, suggests the possibility of PE.
Electrocardiography (ECG) findings in acute PE are generally nonspecific and include T-wave changes, ST-segment abnormalities, incomplete or complete right bundle-branch block, right axis deviation in the extremity leads, and clockwise rotation of the QRS vector in the precordial leads. The changes that do occur are likely caused by right-heart dilatation. Approximately 20% of patients with PE have no ECG changes. Therefore, ECG cannot be relied upon to rule in or rule out PE, although ECG proof of a clear alternative diagnosis, such as MI, is useful when PE is among the possible diagnoses. The “classic” S1 Q3 T3 pattern described by McGinn and White75 in 1935 in seven patients with acute cor pulmonale secondary to PE was subsequently demonstrated to be present in approximately 10% of PE cases.76 In patients without underlying cardiac or pulmonary disease from the UPET (Urokinase Pulmonary Embolism Trial), ECG abnormalities were documented in 87% of patients with proven PE.77 These findings were not specific for PE, however. In this clinical trial, 26% of patients with massive or submassive PE and 32% of those with massive PE had manifestations of acute cor pulmonale, such as the S1 Q3 T3 pattern, right bundle-branch block, a P-wave pulmonale, or right axis deviation. The low frequency of specific ECG changes associated with PE was confirmed in the PIOPED study.68
Despite its lack of diagnostic accuracy, ECG may be helpful in predicting adverse clinical outcomes in patients with PE. It was recently suggested that a T-wave inversion in V2 or V3 is the most frequent ECG sign of massive PE.78 In another PE study, both the pseudoinfarction pattern (Qr in V1) (Fig. 72–1) and T-wave inversion in V2 were closely related to the presence of RV dysfunction and were independent predictors of adverse clinical outcome.79 In a recent trial, an abnormal ECG at presentation proved to be an independent predictor of an adverse outcome, although no individual abnormality appeared capable of predicting such an outcome after being adjusted for the patients’ clinical symptoms and findings on admission and for the presence of preexisting cardiac or pulmonary disease.80
Figure 72–1.
Twelve-lead electrocardiogram from a 54-year-old man with massive pulmonary embolism and cardiogenic shock. Several signs of right ventricular strain are present, including sinus tachycardia with a heart rate of 100 beats/min; right axis deviation; SI SII SIII; clockwise rotation of QRS vector in the precordial leads; Qr in V1; and T-wave inversion in V2.
The chest radiograph is abnormal in the majority of patients with PE, but the findings are nonspecific and often subtle. Atelectasis, cardiomegaly, pulmonary infiltrates, small pleural effusions, and mild elevation of a hemidiaphragm may be present.68,81 Classic radiographic evidence of pulmonary infarction (Hampton hump) or decreased vascularity (Westermark sign) are suggestive but uncommon. A normal chest radiograph in the presence of significant dyspnea and hypoxemia without evidence of bronchospasm or anatomic cardiac shunt is strongly suggestive of PE. In most situations, however, the chest radiograph cannot be used to definitively diagnose or exclude PE. Although exclusion of other processes such as pneumonia, congestive heart failure, pneumothorax, or rib fracture (which may cause symptoms similar to acute PE) is important, it is important to recognize that PE often coexists with other underlying lung diseases.
Contrast-enhanced CT of the chest has become the most useful imaging test in patients with clinically suspected acute PE.
First-generation scanners have poor resolution in the segmental pulmonary arteries and a limited sensitivity for subsegmental clots. However, they appear to predict a benign clinical course over the ensuing 3 months.82
Second-generation scanners involve continuous movement of the patient through the scanner with concurrent scanning by a constantly rotating gantry and detector system.83-89 A helix of projecting data is obtained. Continuous volume acquisitions can be obtained during a single breath. Rapid scans can be obtained, facilitating imaging in critically ill patients.
The latest generation of multidetector-row CT scanners (Figs. 72–2 and 72–3) permits image acquisition of the entire chest with 1-mm or submillimeter resolution with a breathhold of less than 10 seconds.90,91
Figure 72–2.
Contrast-enhanced 16-slice computed tomography scan in a 72-year-old man with extensive, acute central pulmonary embolism showing a “saddle embolus” (arrows) extending into both central pulmonary arteries. Colored volume-rendering technique seen from an anterocranial (A) and anterior (B) perspective allows intuitive visualization of location and extent of embolism.
Figure 72–3.
Contrast-enhanced 16-slice computed tomography (coronal reconstruction) in a 63-year-old man with multiple segmental pulmonary emboli (arrows). As an incidental finding, the examination also revealed a focal lung lesion in the left upper lobe, which was later confirmed to be stage I small cell lung cancer.
Limitations of chest CT in early clinical studies included poor visualization of horizontally oriented vessels in the right middle lobe and lingula because of volume averaging.84 The peripheral areas of the upper and lower lobes may be inadequately scanned, and the presence of intersegmental lymph nodes may result in false-positive study results. Multiplanar reconstructions in coronal, sagittal, or oblique planes aid in distinguishing lymph nodes from emboli. CT is capable of revealing emboli in the main, lobar, or segmental pulmonary arteries with more than 90% sensitivity and specificity.84-88 However, for subsegmental emboli, the sensitivity and specificity are lower. The incidence of isolated subsegmental emboli with first- and second-generation scanners appears to be approximately 6% to 30%.89 When CT is being used for PE diagnosis, it should be incorporated into an overall diagnostic strategy that includes pretest probability, d-dimer testing, and perhaps ultrasound examination of the deep veins.90,91 An additional advantage is evaluating a patient for the entire spectrum of VTE in one imaging session by scanning the legs and pelvis as well as the lungs.
Outcome studies have demonstrated that embolism can be safely excluded using a clinical assessment tool, d-dimer testing, and CT except in patients who present with a high clinical likelihood of embolism.92-95 In the recently published PIOPED II trial, the sensitivity of CT was 83%, a finding somewhat at odds with published outcome data.95 PIOPED II did confirm the usefulness of clinical assessment in that the negative predictive value of a normal CT scan was 96% in patients with a low probability of embolism and 89% for those with an intermediate probability, but only 60% in those with a high-probability scan finding.
Normal results on CT scanning, therefore, appear capable of excluding embolism in embolic suspects with low or intermediate probabilities of the disease. In embolic suspects with a high probability of embolism and negative CT angiogram results, additional diagnostic testing should be considered. As demonstrated in the initial PIOPED trial, a negative V/Q scan or contrast pulmonary angiogram would achieve this end.96 Sequential, noninvasive, lower extremity examinations in patients with adequate cardiopulmonary reserve, although not confirming that embolism did not occur, would render the probability of recurrence unlikely. Alternatively, additional testing should be considered in patients with a low clinical probability of embolism and a CT scan that is suggestive but not clearly diagnostic of embolism as the positive predictive value of CT scanning under this circumstance in PIOPED II was only 58%.95
Another important advantage of chest CT is the concomitant ability to define nonvascular and vascular structures such as airway, parenchymal, and pleural abnormalities; lymphadenopathy; and cardiac and pericardial disease. This is very important for rapid detection of alternative diagnoses (eg, aortic dissection, pneumonia, pericardial tamponade) in patients with acute “chest syndromes” in the emergency setting. Intravenous (IV) contrast agents may precipitate renal failure in patients with renal insufficiency. Patients with a history of allergy to contrast agents should receive preprocedure treatment with steroids administered at least 6 hours before the procedure if possible. H1 and H2 histamine blockers may be used in addition to corticosteroids, but opinion is divided.
V/Q scanning has been the pivotal diagnostic test for suspected PE for many years. Although clinical indications for the study remain (contrast allergy, renal insufficiency, pregnancy), chest CT has now virtually replaced lung scanning.
V/Q scanning is nondiagnostic in up to 70% of patients with suspected PE. Normal and high-probability scans are considered diagnostic. A normal perfusion scan excludes the diagnosis of PE with enough certainty that further diagnostic testing is unnecessary.
In the PIOPED study, the usefulness of lung scanning combined with clinical assessment of patients with suspected PE was prospectively evaluated.96 Patients with PE had scans that were of high, intermediate, or low probability, but so did most individuals without PE. Although the specificity of high-probability scans was 97%, the sensitivity was only 41%. Of interest, 33% of patients with intermediate-probability scans and 12% of those with low-probability scans were diagnosed definitively with PE by pulmonary arteriography. Forty percent of low-probability scans in the presence of high clinical suspicion were followed by documentation of PE at angiography. When the clinical suspicion of PE was considered very high, the positive predictive value of high-probability scans for PE was 96%. In patients with nondiagnostic lung scans, further diagnostic testing for PE should be undertaken.
Standard contrast pulmonary arteriography (Fig. 72–4) is the established “gold standard” imaging test for the diagnosis of PE. The risk of subsequent VTE in patients with a negative test result and without anticoagulation is less than 2%. Two referee readers from the PIOPED study agreed on the presence or absence of subsegmental emboli in only 66% of cases.97 In another study, using selective pulmonary arteriography, there was excellent agreement on main, lobar, and segmental emboli but only 13% agreement on subsegmental emboli.98
Figure 72–4.
Selective conventional angiography of the right pulmonary artery in a 67-year-old woman with acute onset of dyspnea and chest pain, an elevated d-dimer enzyme-linked immunosorbent assay level, and an inconclusive ventilation–perfusion scan. The right upper lobe artery is obstructed with visualization of the tail of the embolus in the proximal right upper lobe artery (arrows).
Gadolinium-enhanced magnetic resonance (MR) angiography is also being used to evaluate clinically suspected PE.99-101 Recent study have demonstrated that when MR is performed under optimal conditions, it appears to be highly sensitive and specific even for segmental PE compared with pulmonary angiography.100-102 MR has several attractive advantages over chest CT, including excellent sensitivity and specificity for the diagnosis of DVT and no requirement of ionizing radiation or iodinated contrast agents. Furthermore, MR technology also allows assessment of RV and RV size and function—potentially important for the risk stratification of PE patients. Use of the technique has been hampered by the cost, required scanning time in relationship to CT, and availability of scanners.
Transthoracic echocardiography (Fig. 72-5A-C) has emerged as a potentially important tool for risk assessment and treatment guidance in patients with acute PE. The presence of RV dysfunction on a baseline echocardiogram in normotensive patients appears to represent an independent predictor of an adverse outcome or early death.3,103-107 Patients with severe RV dysfunction may show regional wall motion abnormalities of the RV known as the McConnell sign, which is evidence of severe hypokinesis of the RV free wall combined with preserved systolic contraction of the RV apex.108
Approximately 40% of normotensive patients with symptomatic PE have echocardiographic evidence of RV dysfunction, and it remains controversial whether all such patients, the majority of whom will do well with conventional therapy, should be subjected to the risks of thrombolytic therapy.
Echocardiography is also useful to diagnose patent foramen ovale in patients with suspected paradoxical embolism, directly visualizing thrombi in the main pulmonary artery (Fig. 72–5D), right heart chambers, and vena cava.109,110
Figure 72–5.
Chest computed tomography and echocardiographic findings in a 55-year-old man with submassive pulmonary embolism. A. Chest computed tomography scan demonstrating multiple segmental emboli, including a central embolus in main pulmonary artery and extending into right pulmonary artery (R-PA). B. Transthoracic apical four-chamber view with severe right ventricular (RV) dilatation. C. Transthoracic parasternal short-axis view showing flattening of interventricular septum (“D-shaped” left ventricle [LV], arrowheads). D. Transthoracic short-axis view demonstrating the central clot in the main and R-PAs. Ao, aorta; L-PA, left pulmonary artery; PA, pulmonary artery; RA, right atrium; right pulmonary artery. From Garg RK, Bednarz J, Spencer KT, Lang RM. Acute pulmonary embolism. Circulation. 2000;102:2441, with permission.
A number of diagnostic techniques can be used to evaluate patients with suspected DVT. Compression ultrasonography (duplex ultrasonography) is the most common technique used in the United States and in many other areas of the world. Impedance plethysmography is used at some centers, and a number of important clinical trials have been performed using this now-outdated technique. Magnetic resonance imaging (MRI) appears to have some important advantages, but it has not generally been used as a first-line test because of cost and lack of availability. CT venography used alone or in conjunction with CT angiography appears to have a sensitivity and specificity equivalent to that of duplex ultrasonography but exposes the patient to additional radiation. Contrast venography remains the gold standard, but it is performed infrequently in view of the accuracy of ultrasonography. Each diagnostic technique has advantages and limitations. Although diagnostic algorithms may be suggested for suspected DVT, these are institution specific, depending on resources and available expertise with certain techniques.
Compression ultrasonography with venous imaging is a portable, accurate, and widely available diagnostic technique for DVT.111 The primary criterion to diagnose DVT by ultrasonography is the noncompressibility of the vein. Combined with a Doppler reading, this technique is referred to as duplex ultrasonography. Ultrasound technology has been further improved by the development of color duplex instrumentation that displays Doppler frequency shifts as color superimposed on the grayscale image. The color duplex images display both mean blood-flow velocity, expressed as a change in hue or saturation, and direction of blood flow, displayed as red or blue. Ultrasound imaging techniques can also identify or suggest the presence of pathology other than DVT such as Baker cysts, hematomas, lymphadenopathy, arterial aneurysms, superficial thrombophlebitis, and abscesses.112 The sensitivity and specificity of ultrasonography for symptomatic proximal DVT has been well above 90% in most recent clinical trials.113,114 There are limitations, including insensitivity for asymptomatic DVT (<50%), operator dependence, the inability to accurately distinguish acute from chronic DVT in symptomatic patients, and insensitivity for calf vein thrombosis.115-117 Compared with other technologies, ultrasonography is relatively inexpensive and is the preferred diagnostic modality for symptomatic suspected proximal DVT.
Ultrasonography is considered diagnostic for PE if it confirms DVT in patients with PE symptoms. However, approximately 50% of patients with CT-confirmed embolism have no imaging evidence of lower extremity venous thrombosis.118 Thus, embolism cannot safely be ruled out in patients with suspected PE on the basis of a negative lower extremity duplex ultrasonography. In patients with suspected embolism and a negative multidetector-row CT examination of the chest, the addition of duplex ultrasonography appears to only minimally increase diagnostic yield.
Contrast venography is a costly and invasive procedure that may result in superficial phlebitis or hypersensitivity reactions, but it is generally safe and accurate. Although contrast venography is the gold standard for DVT diagnosis, it is now rarely performed except in clinical trials because of its higher sensitivity in detecting asymptomatic thrombi than duplex ultrasonography.119 DVT is usually diagnosed with compression or Doppler ultrasonography, which is widely available, noninvasive, and accurate. Alternative diagnostic approaches are contrast CT and MRI.
Venography is performed whenever noninvasive testing is nondiagnostic or impossible to perform or during interventional procedures such as catheter-directed thrombolysis, catheter embolectomy, percutaneous angioplasty, or insertion of an inferior vena cava (IVC) filter.
Impedance plethysmography has been used in patients who presented with suspected acute DVT but is rarely obtained in the era of ultrasonography.
MRI has clear advantages as a diagnostic test for suspected DVT and appears to be an accurate, noninvasive alternative to venography.120,121 A major feature of this technique is excellent resolution of the IVC and pelvic veins.99,100 Preliminary experience with MRI suggests that it is at least as accurate as contrast venography or ultrasound imaging and more sensitive than ultrasonography for pelvic vein and calf-limited thrombosis.121-123 Simultaneous bilateral lower extremity imaging can be accomplished, and MRI appears to accurately distinguish acute from chronic DVT. This technique is also useful in differentiating other entities such as cellulitis or a Baker cyst from acute DVT. As with many other diagnostic techniques, its usefulness depends to a certain degree on the experience of the reader.
Diagnostic Strategy
The overall diagnostic approach depends on the hemodynamic presentation of the patient. Although rapid diagnosis and therapeutic intervention are required in patients with shock and suspected massive PE, there is sufficient time to obtain imaging tests in hemodynamically stable patients with suspected PE.
In patients with hypotension or cardiogenic shock associated with suspected massive PE, rapid initiation of therapy is potentially lifesaving. The definition of massive PE should be based on hemodynamic considerations rather than purely anatomic considerations.7 Irrespective of the degree of vascular obstruction, patients with PE who present with shock have a mortality rate that approaches 30%, but those with cardiopulmonary arrest have a mortality rate of approximately 70%. Time-consuming imaging tests often can be avoided when emergency bedside echocardiography is available. In patients with suspected massive PE and evidence of severe acute RV dysfunction on the echocardiogram, thrombolysis or embolectomy may be rapidly initiated. A caveat to this general recommendation exists in patients presenting with decompensated right-heart failure caused by nonembolic forms of pulmonary hypertension. Clues to the chronic nature of the RV dysfunction include a history of chronic rather than acute dyspnea, the presence of RV hypertrophy rather than simple dilatation, or estimated pulmonary artery systolic pressures by echocardiography that are greater than approximately 70 mm Hg.
PE cannot be excluded without objective testing. The history, physical examination, and diagnostic studies (eg, chest radiography, ECG, arterial blood gas analysis) can raise or lower the clinical suspicion of embolism but are incapable of excluding or confirming it unless a clearly identifiable condition (eg, pneumothorax) is identified to account for the patient’s complaints. Noninvasive strategies have been investigated and algorithms constructed that are capable of confirming or excluding the diagnosis of embolism under most circumstances.
In all emergency department patients and other outpatients, the clinical pretest probability for PE should be calculated by implicit assessment or, preferably, through a standardized technique.70-72 Unless the pretest probability is high, a highly sensitive plasma d-dimer assay such as an ELISA should be obtained. PE can be excluded by a highly sensitive d-dimer result below the assay-specific cutoff level except in patients with a high pretest clinical probability. In patients with elevated d-dimer levels or a high clinical probability of embolism, spiral chest CT should be obtained (Fig. 72–6). In patients with significant impairment of renal function, pregnancy, or allergy to contrast agents, lower extremity duplex study or V/Q perfusion scanning may be preferred as the primary chest imaging test. A V/Q scan can also be performed in patients with a nondiagnostic or negative chest CT when the clinical suspicion of PE persists. A normal V/Q is capable of excluding the diagnosis, but a high-probability scan is capable of confirming the diagnosis in patients with a high or intermediate probability of disease. If a high clinical suspicion for PE persists, pulmonary angiography can be performed. This strategy is safe and requires pulmonary angiography in fewer than 10% of patients.125
Figure 72–6.
Diagnostic strategy for patients with suspected pulmonary embolism (PE) without shock. In this strategy, chest computed tomography (CT) is used as the principal imaging test. *Further testing should be considered if the test is inconclusive or negative, with a persistent suspicion of PE. ELISA, enzyme-linked immunosorbent assay.
The approach to a hospitalized patient with suspected embolism is different. At the present time, the safe exclusion of embolism in inpatients using a clinical prediction rule and d
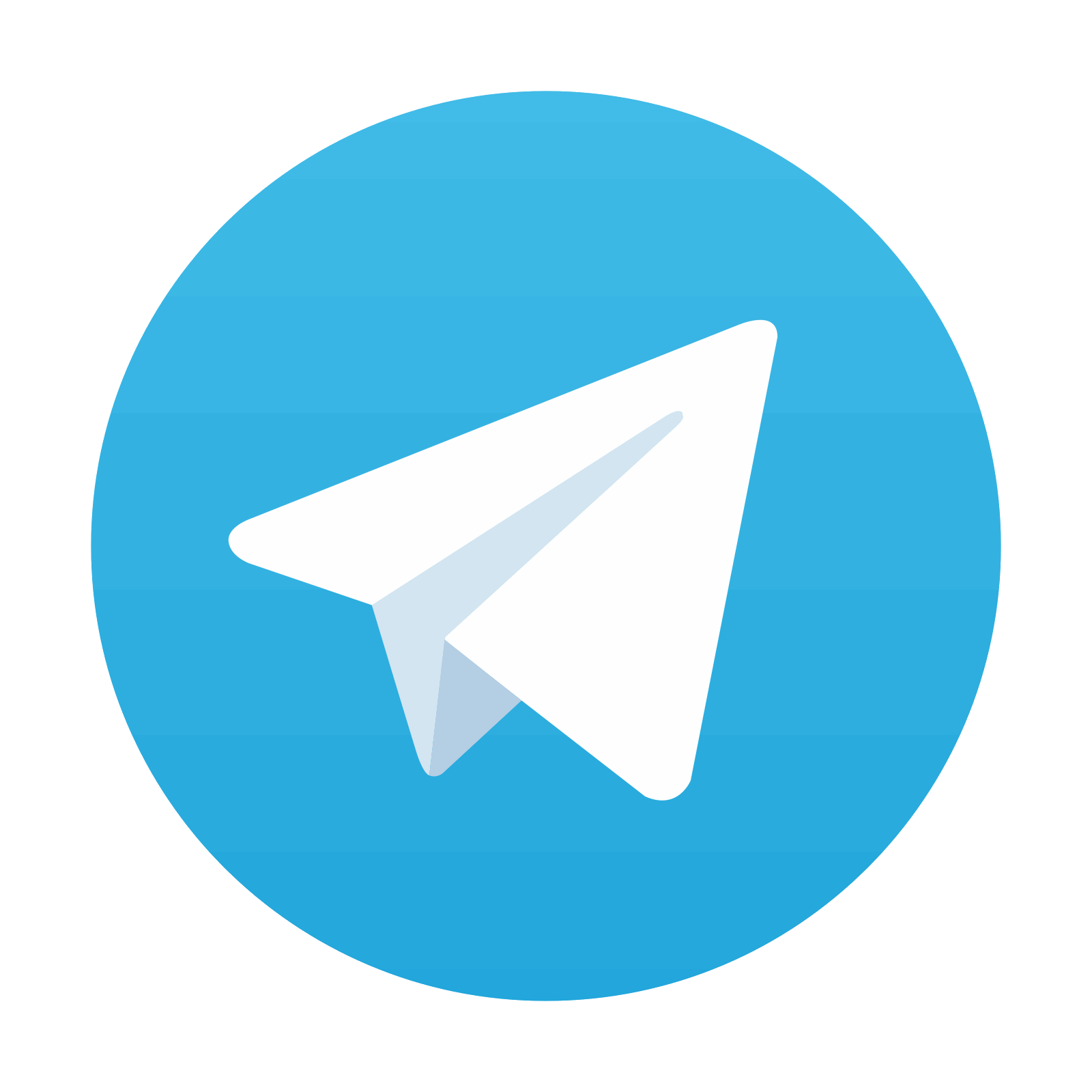
Stay updated, free articles. Join our Telegram channel

Full access? Get Clinical Tree
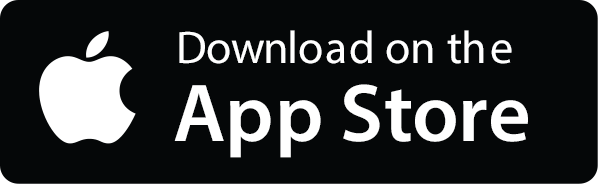
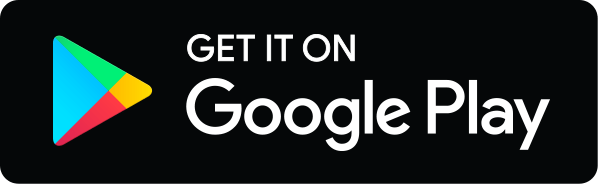
