Prosthetic Valve Disease
Mario J. Garcia
Historical Perspective
For many patients with valvular heart disease, prosthetic valve replacement is the only effective therapy. Hufnagel successfully implanted the first prosthetic valve in 1952 (1) in the descending aorta of a patient with aortic regurgitation. The first in situ aortic valve replacement was performed by Harken (2) in 1953 and the first mitral valve by Starr (3) in 1960. These bare-strut ball valves led to the development of the Starr-Edwards ball-and-cage valve. Tilting-disc valves were developed in the late 1960s and represented a significant advance by demonstrating a far superior hemodynamic performance over the ball-and-cage valves. Double-leaflet hingeless tilting valves were developed in the early 1970s, and since then have become the most commonly used mechanical valves. The first tissue valve was an aortic homograft inserted by Ross in 1962 (4). Stented porcine valve xenografts were first implanted in 1965 by Carpentier (5), which led to the development of the Hancock and the Carpentier-Edwards valves. Stentless porcine valves were later introduced, and these have gained increasing popularity over the last decade. Percutaneously deployed aortic prosthetic valves and mitral valve repair devices have been recently implanted with success and are poised to revolutionize the management of valvular heart disease.
Normal Prosthetic Valve Anatomy and Function
Examples of common models of prosthetic valves are shown in Figure 25.1. Most mechanical and tissue valve prostheses have distinct components: a sewing ring, supporting struts, and an occluder device. Sewing rings are constructed from metallic alloys or plastics and are covered with a cloth through which sutures are passed to implant the valve. The size of the prosthesis is determined by the diameter of the suture ring. The supporting struts hold the occluders in place. The occluders may be composed of plastic, metal, or biologic tissue. Each type of prosthesis has specific features that may permit their recognition by means of auscultation, two-dimensional and Doppler echocardiography, and/or cinefluoroscopy. Occasionally, invasively obtained catheter gradients are needed to determine prosthetic valve dysfunction. Retrograde catheter crossing of tissue prostheses and ball-and-cage prosthesis may be performed safely to determine transvalvular gradients. Tilting-disc prostheses should not be crossed with these catheters because of the risk of catheter entrapment (6).
Stented tissue valves and mechanical prostheses have smaller effective orifices than normal native valves of the same size. This reduction in effective orifice is caused by the profile of the suture ring and the supporting struts. For this reason, most tissue and mechanical prostheses in a semilunar position will generate a systolic flow murmur. Diastolic murmurs in atrioventricular prosthetic valves are uncommonly heard unless there is prosthetic stenosis or high forward flow due to severe valvular regurgitation (7). The normal auscultatory findings of prosthetic valves may be altered by factors such as chest wall thickness, emphysema, stroke volume, and heart rate.
Some prosthetic valves may be identified from plain chest radiography (Fig. 25.2). Suture rings are often radiopaque, as are prosthetic cages and some tissue-valve-supporting stents. Tilting discs may or may not be seen in a plain film depending on the angle of the prosthesis, the construction material, and the x-ray penetration. Cinefluoroscopy may be useful for evaluating the mobility of the occluder of mechanical valves in patients with suspected obstruction. Prosthetic valve suture rings, cages, discs, and stents may produce echocardiographic shielding or lead to an inability to visualize structures or flow beyond the location of the ultrasound-reflecting surface. This limits the ability to detect transvalvular or paravalvular regurgitation, thrombi, or vegetations of infective endocarditis in mitral prostheses by transthoracic echocardiography (8). In those cases, transesophageal echocardiography offers superior visualization.
The effective orifice of the prosthesis and the cardiac output determine the magnitude of the transvalvular gradients. Transvalvular gradients are most commonly estimated
from continuous-wave Doppler velocities by means of the modified Bernoulli equation (ΔP = 4V2). In most instances, Doppler-derived and catheter-derived gradients are comparable. However, Doppler measurement often overestimates the gradients of small mechanical valves in the aortic position (9). This discrepancy between catheter-derived and Doppler gradients is the result of pressure recovery upstream from the prosthesis. All mechanical and most biologic stented valves have a certain amount of intravalvular regurgitation.
from continuous-wave Doppler velocities by means of the modified Bernoulli equation (ΔP = 4V2). In most instances, Doppler-derived and catheter-derived gradients are comparable. However, Doppler measurement often overestimates the gradients of small mechanical valves in the aortic position (9). This discrepancy between catheter-derived and Doppler gradients is the result of pressure recovery upstream from the prosthesis. All mechanical and most biologic stented valves have a certain amount of intravalvular regurgitation.
Mechanical Valves
The first mechanical valves had a ball-and-cage design with a metallic alloy ring attached to a cage in which either a metallic or Silastic ball moves from a closed position occluding the ring to an open position in the outer end of the cage. Flow across the prosthesis is directed circumferentially around the ball, assuming a lateral angle. The Starr-Edwards ball-and-cage prosthesis has excellent durability, in many instances exceeding 30 years (10). A major disadvantage of ball-and-cage valves is their suboptimal hemodynamic profile. The mean transvalvular gradient for Starr-Edwards ball-and-cage prostheses in the mitral position is 4.6 + 2.4 mm Hg and the estimated effective orifice is 1.4 to 2.9 cm2 (11,12,13,14,15). In the aortic position, mean gradient is 23 + 8 mm Hg and estimated effective orifice is 1.2 to 1.6 cm2 (16,17). On auscultation, the ball-and-cage valves produce a loud opening click followed by multiple clicks of variable intensity produced by the ball bouncing in the cage and a flow murmur in the aortic position. A less intense click occurs with the closing of the valve. Radiographically, the support ring and cage can be detected in plain films. In certain models of ball-and-cage prostheses the ball is metallic or barium-impregnated, allowing its visualization. Two-dimensional echocardiography can demonstrate the highly reflective echoes produced by the metallic struts and the less intense reflective echoes produced by the proximal surface of the ball. In the disc-and-cage valves, such as the Kay-Suzuki valve, a flat disc replaces the ball occluder, providing a lower profile. These valves, however, have been discontinued due to the gradual erosion and often fatal embolization of the disc.
Tilting-disc valves consist of a metallic ring attached to a single or to two flat or concave discs that pivot from the occluded position to an open position at an angle of 60 to 85 degrees. The Björk-Shiley valve has been manufactured in six different models, four of which are available in the United States. The original models had a flat disc made of Delrin or pyrolytic carbon; this was later replaced with a convexoconcave disc. Fracture of the welded struts and embolization of the disc led to the withdrawal of these valves from the market. The Omniscience tilting-disc valve (18) has a free-floating concave-convex disc in titanium housing. This valve, no longer in use, has considerably higher risk of thrombosis, probably due to the smaller built-in volume of regurgitation (19). The Medtronic-Hall valve has a one-piece housing manufactured from titanium and a rotatable Teflon sewing ring and is still in use. A small orifice in the center permits regurgitant flow to “wash” potentially thrombogenic material from the disc. Currently available single tilting-disc valves have excellent hemodynamics and low risk of mechanical failure. The St. Jude Medical (20) and the Carbomedics (21) valves are bileaflet tilting-disc valves. The
leaflets of the St. Jude Medical prosthesis are manufactured from pyrolytic carbon impregnated with tungsten for radiopacity. These semicircular leaflets open to an 85-degree angle, leaving two larger lateral orifices and a narrow rectangular slit between them. The flow across bileaflet tilting-disc valves is symmetric, providing excellent hemodynamic performance (22). The rates of mechanical failure and thromboembolism are very low. The Carbomedics bileaflet valve has similar characteristics and hemodynamic performance. Their mean transvalvular gradient is significantly lower than equally sized Starr-Edwards ball-and-cage valves, from 6 to 15 mm Hg in the aortic and from 2 to 7 mm Hg in the mitral position. Durability at 15 years has been shown to be excellent for this valve (23). The estimated effective orifices range from 2.1 to 3.9 cm2 for mitral and 1.3 to 2.5 cm2 for aortic prostheses. The auscultatory findings of tilting-disc prostheses include a loud single or double closing click and a soft midsystolic ejection murmur in the aortic or a low-frequency diastolic rumble in the mitral position. Tilting-disc prostheses also produce a softer opening click. These valves have different radiographic appearance based on the number and position of the struts and the tilting discs. In some valves, such as the Björk-Shiley and Wada-Cutter valves, the discs may be radiolucent. The two half-discs of bileaflet valves can be observed from a tangential plane (Fig. 25.3). The motion of the valve ring and the opening angle of the discs can be evaluated under fluoroscopic guidance. Color Doppler may help to identify the type of prosthesis. The Medtronic-Hall tilting-disc valve has a characteristic regurgitant flow jet through its central orifice. The St. Jude’s Medical valve has two central divergent and multiple peripheral convergent regurgitant jets that may be visualized depending on the imaging angle (Fig. 25.4).
leaflets of the St. Jude Medical prosthesis are manufactured from pyrolytic carbon impregnated with tungsten for radiopacity. These semicircular leaflets open to an 85-degree angle, leaving two larger lateral orifices and a narrow rectangular slit between them. The flow across bileaflet tilting-disc valves is symmetric, providing excellent hemodynamic performance (22). The rates of mechanical failure and thromboembolism are very low. The Carbomedics bileaflet valve has similar characteristics and hemodynamic performance. Their mean transvalvular gradient is significantly lower than equally sized Starr-Edwards ball-and-cage valves, from 6 to 15 mm Hg in the aortic and from 2 to 7 mm Hg in the mitral position. Durability at 15 years has been shown to be excellent for this valve (23). The estimated effective orifices range from 2.1 to 3.9 cm2 for mitral and 1.3 to 2.5 cm2 for aortic prostheses. The auscultatory findings of tilting-disc prostheses include a loud single or double closing click and a soft midsystolic ejection murmur in the aortic or a low-frequency diastolic rumble in the mitral position. Tilting-disc prostheses also produce a softer opening click. These valves have different radiographic appearance based on the number and position of the struts and the tilting discs. In some valves, such as the Björk-Shiley and Wada-Cutter valves, the discs may be radiolucent. The two half-discs of bileaflet valves can be observed from a tangential plane (Fig. 25.3). The motion of the valve ring and the opening angle of the discs can be evaluated under fluoroscopic guidance. Color Doppler may help to identify the type of prosthesis. The Medtronic-Hall tilting-disc valve has a characteristic regurgitant flow jet through its central orifice. The St. Jude’s Medical valve has two central divergent and multiple peripheral convergent regurgitant jets that may be visualized depending on the imaging angle (Fig. 25.4).
![]() FIGURE 25.2. Radiographic appearance in the anteroposterior projection of mechanical valves in the aortic (A), mitral (M), and tricuspid (T) positions. |
Tissue Valves
The biologic nonhuman tissue valves currently available are manufactured from either porcine valvular tissue or bovine pericardium mounted in supporting stents or preserved in situ in a segment of the porcine ascending aorta. The Carpentier-Edwards porcine valve (24) consists of a cloth-covered silicone-rubber sewing ring attached to three cloth-covered steel alloy flexible stents (25). The Hancock porcine valve has very similar characteristics, but the stents are manufactured of polypropylene. Bioprosthetic valves are structurally similar to human semilunar valves. Despite their human anatomic resemblance, these valves have suboptimal hemodynamic performance in
comparison to tilting-disc mechanical prostheses of the same diameter (26,27). This limitation results primarily from the reduction in their profile caused by the interposed stents and is more significant in valves smaller than 23 mm. The bioprosthetic leaflets are also stiffer than normal native human semilunar valves, exhibiting an incomplete opening at low flow rates. The durability of porcine bioprosthetic valves has improved significantly with newer changes in design and preservation techniques. Actuarial probability of freedom from structural valve degeneration at 5, 10, and 15 years has been reported at 99%, 79% and 57%, respectively (28). Durability is reduced in mitral prostheses because of the greater closing pressure in this position in younger patients and patients with increased calcium metabolism (29,30). Bovine, and more recently equine (31), pericardial bioprostheses were designed to improve the hemodynamic profile of the porcine valves. The Carpentier-Edwards bovine pericardial valve (32) resembles structurally the porcine tissue valves but appears to have superior hemodynamic performance, demonstrating lower transvalvular gradients during exercise (33). Intermediate follow-up of the Hancock II porcine bioprostheses suggests that their durability will be superior to that of their predecessors. Freedom from reoperation has been reported to be 100%, 97%, and 89% after aortic, mitral, and combined valve replacement, respectively, after 7 years of follow-up (34). Bioprosthetic valves are available in diameters of 19 to 31 mm. The effective orifice area for bioprostheses ranges from 1.4 to 2.5 cm2 in the mitral and 0.9 to 1.8 cm2 in the aortic position. The auscultatory findings of bioprosthetic valves include a systolic ejection murmur in the aortic position. Frequently, a systolic murmur is also heard in patients with mitral bioprostheses, caused by turbulent flow in the left ventricular outflow track. The bioprosthetic valve sewing ring is radiopaque in x-ray films. In some models wire-framed stents may also be seen. The three equidistant stents are the hallmark of bioprostheses by two-dimensional echocardiography. The three leaflets can also be imaged, although they are less echogenic than the native aortic valve. Normal bioprostheses may show mild intravalvular regurgitation by color Doppler (35).
comparison to tilting-disc mechanical prostheses of the same diameter (26,27). This limitation results primarily from the reduction in their profile caused by the interposed stents and is more significant in valves smaller than 23 mm. The bioprosthetic leaflets are also stiffer than normal native human semilunar valves, exhibiting an incomplete opening at low flow rates. The durability of porcine bioprosthetic valves has improved significantly with newer changes in design and preservation techniques. Actuarial probability of freedom from structural valve degeneration at 5, 10, and 15 years has been reported at 99%, 79% and 57%, respectively (28). Durability is reduced in mitral prostheses because of the greater closing pressure in this position in younger patients and patients with increased calcium metabolism (29,30). Bovine, and more recently equine (31), pericardial bioprostheses were designed to improve the hemodynamic profile of the porcine valves. The Carpentier-Edwards bovine pericardial valve (32) resembles structurally the porcine tissue valves but appears to have superior hemodynamic performance, demonstrating lower transvalvular gradients during exercise (33). Intermediate follow-up of the Hancock II porcine bioprostheses suggests that their durability will be superior to that of their predecessors. Freedom from reoperation has been reported to be 100%, 97%, and 89% after aortic, mitral, and combined valve replacement, respectively, after 7 years of follow-up (34). Bioprosthetic valves are available in diameters of 19 to 31 mm. The effective orifice area for bioprostheses ranges from 1.4 to 2.5 cm2 in the mitral and 0.9 to 1.8 cm2 in the aortic position. The auscultatory findings of bioprosthetic valves include a systolic ejection murmur in the aortic position. Frequently, a systolic murmur is also heard in patients with mitral bioprostheses, caused by turbulent flow in the left ventricular outflow track. The bioprosthetic valve sewing ring is radiopaque in x-ray films. In some models wire-framed stents may also be seen. The three equidistant stents are the hallmark of bioprostheses by two-dimensional echocardiography. The three leaflets can also be imaged, although they are less echogenic than the native aortic valve. Normal bioprostheses may show mild intravalvular regurgitation by color Doppler (35).
Stentless heterograft and human homograft prostheses consist of a tubular segment of a porcine/human proximal ascending aorta containing its in situ aortic valve, which is sutured proximally to the aortic annulus and distally to the mid ascending aorta. Short-term and intermediate-term follow-up of stentless valves have demonstrated better hemodynamics with smaller resting and exercise gradients and greater regression of left ventricular (LV) hypertrophy when compared to stented tissue valves of similar size (36,37). Nevertheless this has not been shown to translate into improved clinical outcomes (38). Stentless aortic root bioprosthesis can be used safely to replace the aortic root for aortic valve and aortic root pathology (39). Freedom from structural valve degeneration has been reported to be about 85% at 9 years (40). Human aortic valves are harvested from cadaveric hearts and cryopreserved (41). Similar to stentless heterografts, these are supported by a short segment of the donor’s aortic root, in which the recipient’s coronary arteries are reimplanted. The homograft endothelial cells and fibroblasts are antigenic (42) and are gradually destroyed by immunologic reactions. Freedom from structural valve degeneration at 10, 15, and 20 years has been reported to be 81%, 62%, and 31%, respectively (43). The flow characteristics, auscultatory findings, and radiographic appearance of stentless valves are similar to those of native aortic valves. Homografts have a reduced rate of reinfection, as low as 95% after 20 years, when used to replace valves with endocarditis (44,45). Pulmonary allografts consist of healthy pulmonary valves transplanted to the aortic position to substitute a diseased aortic valve while a cadaveric homograft is then placed in the pulmonic position. Late follow-up has shown no evidence of primary tissue degeneration and normal cusp cellularity. This evidence suggests that the cusps not only survive permanently, but also can grow with the patient, making the operation ideal for children. Freedom from reoperation and actuarial patient survival has been reported at 85% and 80%, respectively, after 20 years. However, aortic insufficiency, degeneration of the implanted homograft in the pulmonary position, and stenosis of the pulmonary trunk anastomotic site are not infrequent causes of reoperation in these patients.
Selection of Prosthetic Valve Type
The selection of a prosthetic valve should always be individualized to each patient based on age, anatomic considerations, lifestyle, expected longevity, and relative risks of anticoagulation. The in-hospital mortality and complication rates after valve replacement are similar with mechanical and bioprostheses (46) and thus should not be considered determining factors in the selection of a valve. Several retrospective and prospective studies have shown that patient survival is comparable at long-term follow-up. Structural failure requiring reoperation is more common with prosthetic valves, whereas thromboembolic and bleeding complications are more common with mechanical valves. Children requiring valve replacement benefit most from mechanical prostheses (47). Tilting-disc mechanical valves provide better hemodynamic performance than bioprostheses particularly at smaller size, thus prolonging the need for reoperation. Structural valve deterioration of bioprosthetic valves also occurs more rapidly in children and young adults (46,48). Therefore, as a general rule, mechanical valves are preferred in younger patients, whereas bioprosthetic valves are recommended in older patients in whom the risk of anticoagulation is higher. Both mechanical and bioprosthetic valves have a disadvantage in women of childbearing age. Pregnant women with mechanical valves require rigorous control of their anticoagulation and are at higher risk for thromboembolism and for fetal hemorrhage and embryopathy (49,50). Bioprosthetic valves may obviate the need for anticoagulation (49,51), but they have the disadvantage of their limited durability. Obviously, bioprosthetic valves should be used in patients at higher risk for anticoagulation-related hemorrhage, including those with intestinal angiodysplasia, familial polyposis, inflammatory bowel disease, liver disease, and hereditary blood dyscrasias.
Pathophysiology of Prosthetic Valves
Complications related to prosthetic heart valves can occur as early as in the immediate postoperative period. Their relative frequency and timing vary with each type of prosthesis and determine the manner in which patients with prosthetic valves should be clinically followed.
Thromboembolic Complications
Thromboembolism represents one of the most important causes of morbidity and mortality in patients with prosthetic valves. The incidence of thromboembolic events is significantly high in non-anticoagulated patients with mechanical prosthetic valves, ranging from 7% to 34% per year (52,53,54). Involved mechanisms include platelet activation by the synthetic surfaces, flow stagnation, increased shear stress, and activation of intrinsic clotting factors by the prosthetic material or the damaged endothelium (55). Thromboembolic complications are more common in older patients, patients in atrial fibrillation, and patients with left ventricular dysfunction (56). The risk of thromboembolism is higher for both mechanical and bioprostheses in the early postoperative period, declining after the first 3 months for bioprosthetic valves. Oral anticoagulation with warfarin significantly reduces the risk of thromboembolism in patients with mechanical heart valves. Improved prosthetic design and anticoagulation reduced the embolic rates to 2.5% to 4% per patient-year in anticoagulated patients with Starr-Edwards valves implanted after 1970 (57). The incidence of thromboembolic complications with Björk-Shiley tilting-disk valves is about 4% per year in the mitral and 2.5% per year in the aortic position, and the incidence is 1% to 3% average with Medtronic-Hall valves in anticoagulated patients (58). Bileaflet mechanical valves have a risk of about 2.5% per year in the mitral and 1% per year in the aortic position (59,60,61). Regardless of their type of mechanical prosthesis, the incidence of thromboembolism is less than 1% in those patients that maintain therapeutic anticoagulation (62). Unfortunately, chronic anticoagulation therapy increases the long-term hemorrhagic complications (Table 25.1). Pooled data from 46 studies including more than 13,000 patients with mechanical heart valves studied for more than 50,000 patient-years showed an incidence of major bleeding complications of about 1.4 per 100 patient-years (63). Hemorrhagic complications occur more frequently in patients with an International Normalized Ratio (INR) above therapeutic range (64) (Fig. 25.5). The intensity of anticoagulation should vary according to the type of the mechanical valve and the presence of other predisposing factors (Fig. 25.6). Antiplatelet therapy may be used in combination with warfarin in patients with mechanical heart valves. Low-dose aspirin (100 mg/day) in patients with a target INR of 3 to 4.5 has been shown to reduce the annualized risk of death and major systemic thromboembolic events from 11.7% to 4.2% (65). The additive benefit of aspirin is greater in patients with previous embolic events and those with coronary artery disease, but it is less clear in others in whom the increased risk of bleeding exceeds a low risk of thromboembolism.
TABLE 25.1 Incidence of adverse events (thromboembolism, major bleeding, and stroke) in patients with mechanical valves receiving chronic anticoagulation | |||||||||||||||||||||||||||||||||||||||||||||
---|---|---|---|---|---|---|---|---|---|---|---|---|---|---|---|---|---|---|---|---|---|---|---|---|---|---|---|---|---|---|---|---|---|---|---|---|---|---|---|---|---|---|---|---|---|
|
Because pregnancy causes relative hypercoagulability (66), rigorous anticoagulation is required throughout gestation in patients with mechanical valve prostheses. Anticoagulant therapy is associated, however, with higher fetal morbidity and mortality, especially if the drugs are administered during the first trimester (67). Warfarin has teratogenic effects in the embryo and may result in fetal death when the embryo is exposed between the sixth and ninth weeks of gestation. Pregnant women with mechanical valves that receive warfarin during
this period carry a risk of embryopathy as high as 30% and a risk of spontaneous abortion of 25% to 30% (68). Therapeutic abortion should be considered if advanced gestation is discovered accidentally while receiving this drug. Anticoagulation should be maintained during pregnancy with either continuous intravenous or subcutaneous heparin administered twice daily starting at 17,500 to 20,000 units and adjusted to a target activated partial thromboplastin time (aPTT) greater than two times control 6 hours after administration (69). Subtherapeutic doses of heparin are associated with a significant risk of thromboembolism. The risk of major hemorrhagic complications in pregnant patients treated with heparin is about 2% (70). Substituting heparin from week 6 until week 12 of gestation eliminates the risk of embryopathy in pregnant women with mechanical valves (50). Warfarin should be substituted again by heparin from after week 38 until delivery because warfarin crosses the placenta and may cause fetal intracranial hemorrhage in the peripartum period (71). Heparin should be discontinued before elective induction of labor. Low-molecular-weight heparins have been shown to be safe and effective for the prevention and treatment of deep venous thrombosis in pregnant women (72), but they have been associated with a 12% risk of thromboembolic complications in pregnant women with mechanical valves (73).
this period carry a risk of embryopathy as high as 30% and a risk of spontaneous abortion of 25% to 30% (68). Therapeutic abortion should be considered if advanced gestation is discovered accidentally while receiving this drug. Anticoagulation should be maintained during pregnancy with either continuous intravenous or subcutaneous heparin administered twice daily starting at 17,500 to 20,000 units and adjusted to a target activated partial thromboplastin time (aPTT) greater than two times control 6 hours after administration (69). Subtherapeutic doses of heparin are associated with a significant risk of thromboembolism. The risk of major hemorrhagic complications in pregnant patients treated with heparin is about 2% (70). Substituting heparin from week 6 until week 12 of gestation eliminates the risk of embryopathy in pregnant women with mechanical valves (50). Warfarin should be substituted again by heparin from after week 38 until delivery because warfarin crosses the placenta and may cause fetal intracranial hemorrhage in the peripartum period (71). Heparin should be discontinued before elective induction of labor. Low-molecular-weight heparins have been shown to be safe and effective for the prevention and treatment of deep venous thrombosis in pregnant women (72), but they have been associated with a 12% risk of thromboembolic complications in pregnant women with mechanical valves (73).
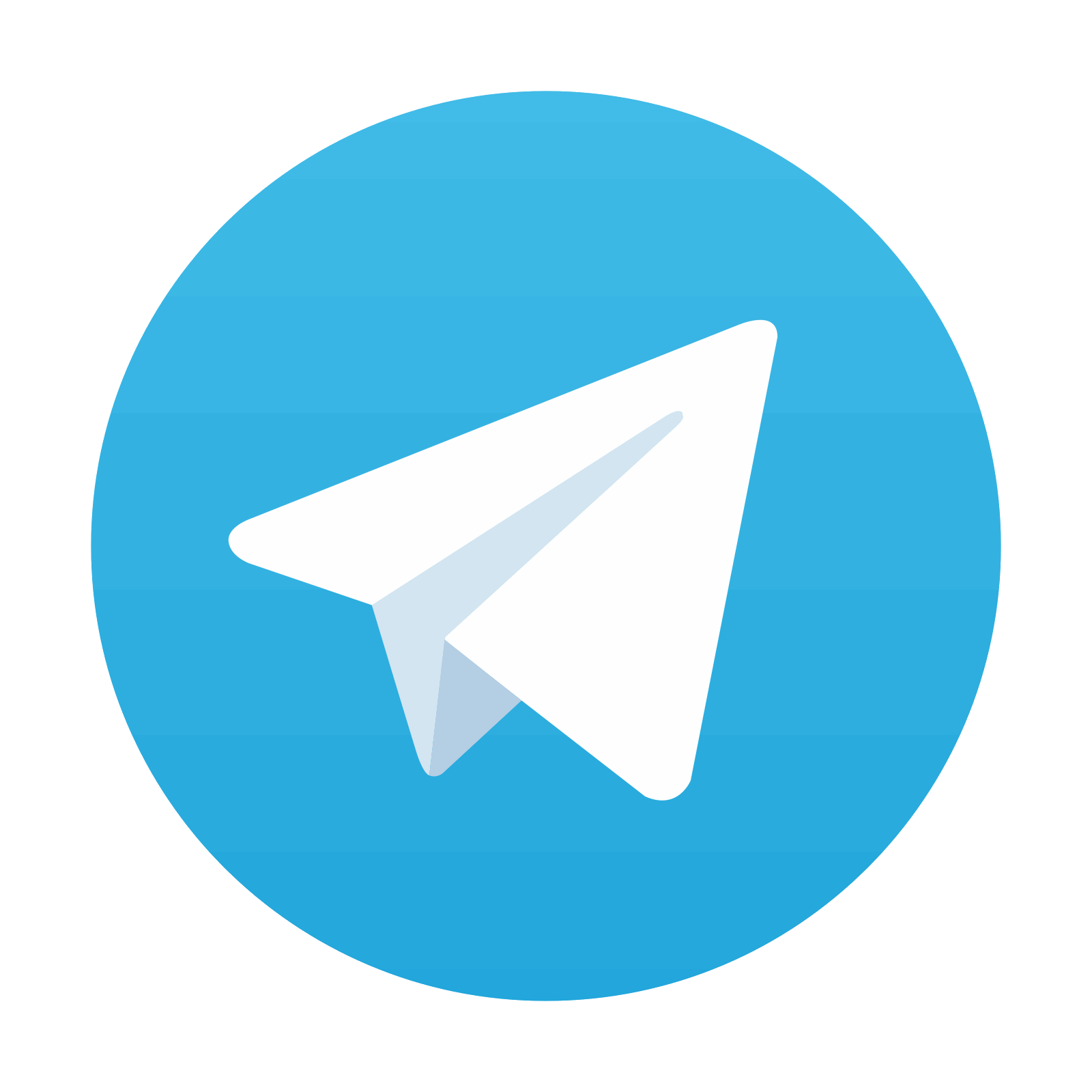
Stay updated, free articles. Join our Telegram channel

Full access? Get Clinical Tree
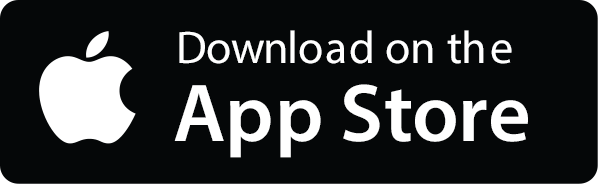
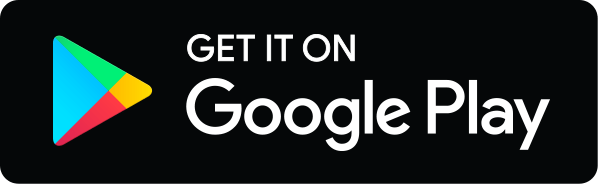