Table 42.1 Dana Point Clinical Classification of Pulmonary Hypertension (2008)1 | |||||||||||||||||||||||||||||||||||||||||||||||||||||||||||||||||||||||||||||||||||||||||||||||||||||||||
---|---|---|---|---|---|---|---|---|---|---|---|---|---|---|---|---|---|---|---|---|---|---|---|---|---|---|---|---|---|---|---|---|---|---|---|---|---|---|---|---|---|---|---|---|---|---|---|---|---|---|---|---|---|---|---|---|---|---|---|---|---|---|---|---|---|---|---|---|---|---|---|---|---|---|---|---|---|---|---|---|---|---|---|---|---|---|---|---|---|---|---|---|---|---|---|---|---|---|---|---|---|---|---|---|---|
|
Table 42.2 Prevalence of PH Groups | ||||||||||||||||
---|---|---|---|---|---|---|---|---|---|---|---|---|---|---|---|---|
|
![]() Figure 42.1 Three major mechanistic pathways are known to be perturbed in patients with PAH. (1) The NO pathway: NO is created in endothelial cells by type III NO synthase (eNOS), which in turn induces guanylate cyclase (GC) to convert guanosine triphosphate (GTP) to cGMP, a second messenger that constitutively maintains pulmonary artery smooth muscle cell (PASMC) relaxation and inhibition of PASMC proliferation. (2) The endothelin (ET) pathway: Big-ET (or pro-ET) is converted in endothelial cells to ET-1 (21 amino acids) by endothelin-converting enzyme (ECE). ET-1 binds to PASMC ETA and ETB receptors, which ultimately leads to PASMC contraction, proliferation, and hypertrophy. ET-1 also binds to endothelial cell ETB receptors. (3) The prostacyclin pathway: The production of PGI2 (prostacyclin) is catalyzed by prostacyclin synthase (PS) in endothelial cells. In PASMCs, PGI2 stimulates adenylate cyclase (AC), thus increasing production of cAMP from ATP, another second messenger that maintains PASMC relaxation and inhibition of PASMC proliferation. Importantly, the pathways interact as illustrated, modulating the effect of any single pathway. They also are impacted by transmitters and stimuli that act at cell membrane receptors (Rec). Examples of these include but are not limited to thrombin, bradykinin, arginine vasopressin (AVP), vessel-wall shear stress, angiotensin II (Ang II), cytokines, and reactive oxygen species (ROS). In addition, the effect of a transmitter depends on its specific site of action (such as PASMC ETA or ETB receptors versus endothelial cell ETB receptor). The large white arrows depict aberrations observed in these pathways among patients with PAH. The orange boxes represent agents with reported clinically beneficial effects in patients with PAH. PDE5-inh indicates PDE-5 inhibitor, e.g., sildenafil; ETRA, endothelin receptor antagonist, e.g., bosentan (dual), ambrisentan, and sitaxsentan (receptor A selective). Prostanoids, e.g., epoprostenol, treprostinil, and iloprost, supplement exogenously deficient levels of PGI2. Red stop signs signify an inhibitory effect of the depicted agents. Dotted arrows depict pathways with known and unknown intervening steps that are not shown.8 (Reproduced with permission from: McLaughlin VV, McGoon MD. Pulmonary arterial hypertension. Circulation 2006;114:1417-1431.) |
![]() Figure 42.2 Diagnostic approach for suspected PAH. Pivotal tests are essential to establishing the definitive diagnosis of PAH.19 (Reproduced with permission from: McLaughlin VV, Archer SL, Badesch DB, et al. ACCF/AHA 2009 expert consensus document on pulmonary hypertension. A report of the American College of Cardiology Foundation Task Force on Expert Consensus Documents and the American Heart Association developed in collaboration with the American College of Chest Physicians; American Thoracic Society, Inc.; and the Pulmonary Hypertension Association. Circulation 2009;119:2250-2294.) |
generally obtained early in the evaluation of patients with suspected PH. The tricuspid regurgitant jet is used to estimate the RVSP, and pressures >40 mmHg are consistent with PH. However, TTE can both under- and overestimate pulmonary artery pressures, as compared to the gold standard of RHC. In addition to the pressure measurements, TTE is useful in identifying elevated right-sided pressures by showing evidence of right atrial or ventricular enlargement, flattening of the interventricular septum, or an underfilled LV.
Ventilation/perfusion (V/Q) scan: the test of choice for the initial evaluation of chronic, surgically accessible thromboembolic disease. A PE protocol CT is excellent for identifying acute PE; however, is not sensitive for chronic thromboembolic disease. If indicated, pulmonary angiography can often be scheduled immediately following RHC if chronic thromboembolic disease is highly suspected.
Pulmonary function tests (PFTs): may show only mild restrictive disease and a mildly decreased diffusing capacity for carbon monoxide (DLCO); scleroderma patients may have a more marked decrease in DLCO.
Polysomnogram: should be performed if a patient endorses symptoms of sleep disordered breathing.
Blood tests: given the association between PH and both connective tissue disorders and chronic liver disease, antinuclear antibody (ANA) tests and liver function tests (LFTs) are routinely performed. HIV testing should be performed if a patient endorses risk factors. A complete blood count is helpful in identifying anemia as a cause of high-output heart failure. Thyroid function studies should be performed in all patients.
Six minute hall walk (6MWT): commonly used to objectively assess functional capacity; this test is often performed at baseline and repeated periodically to assess response to treatment; it is frequently used as an endpoint in clinical PAH trials.
with CCBs. Acute vasodilator testing should be avoided in patients with significantly elevated left heart filling pressures or low cardiac output. Veno-occlusive disease and pulmonary capillary hemangiomatosis should be considered in patients who experience pulmonary edema during vasodilator testing.22
Table 42.3 PAH-Specific Therapies | ||||||||||||||||||||||||||||||||||||||||||||||||||||||||||||||||||||||||||||||||||||||||||||||||||||||||||||||||||
---|---|---|---|---|---|---|---|---|---|---|---|---|---|---|---|---|---|---|---|---|---|---|---|---|---|---|---|---|---|---|---|---|---|---|---|---|---|---|---|---|---|---|---|---|---|---|---|---|---|---|---|---|---|---|---|---|---|---|---|---|---|---|---|---|---|---|---|---|---|---|---|---|---|---|---|---|---|---|---|---|---|---|---|---|---|---|---|---|---|---|---|---|---|---|---|---|---|---|---|---|---|---|---|---|---|---|---|---|---|---|---|---|---|---|
|
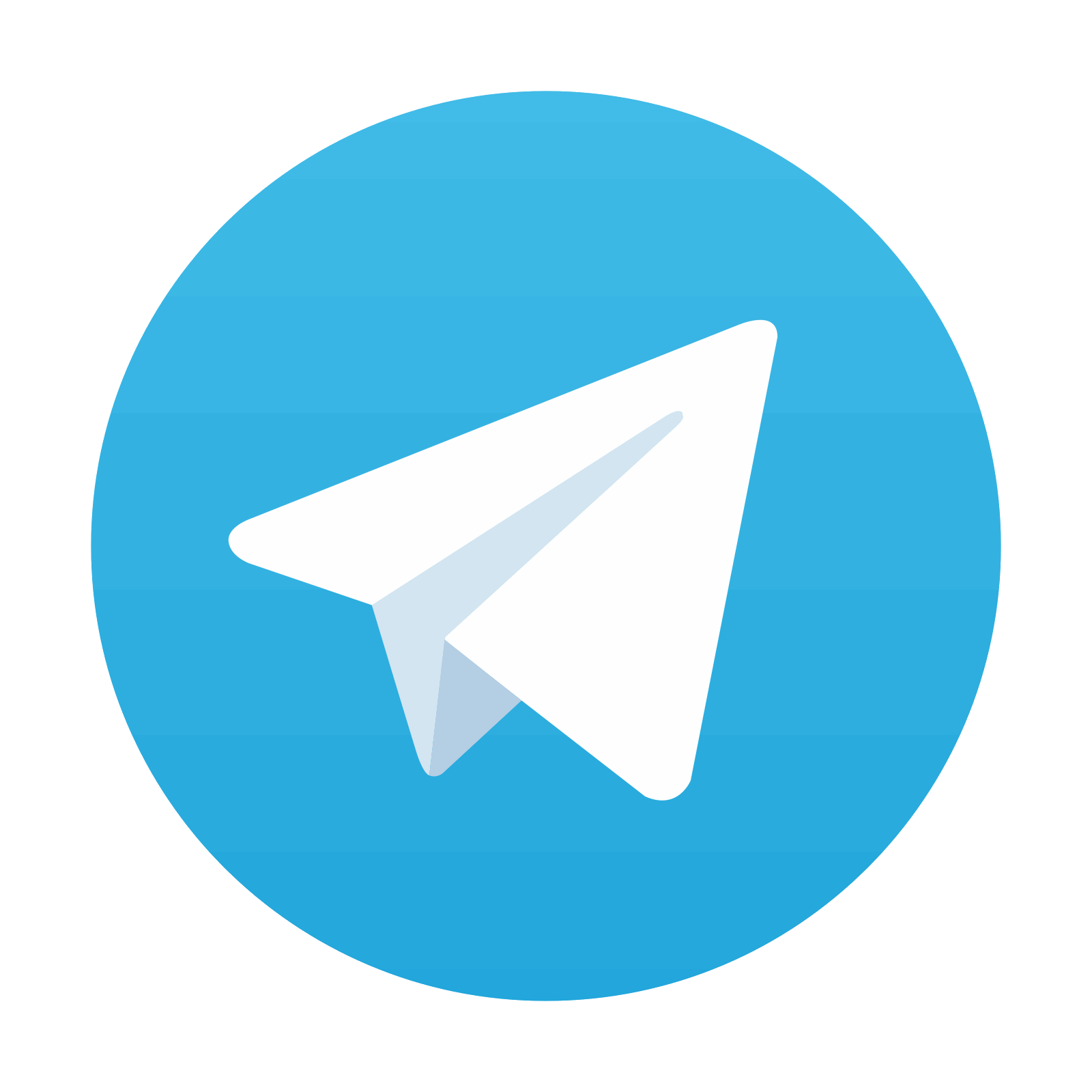
Stay updated, free articles. Join our Telegram channel

Full access? Get Clinical Tree
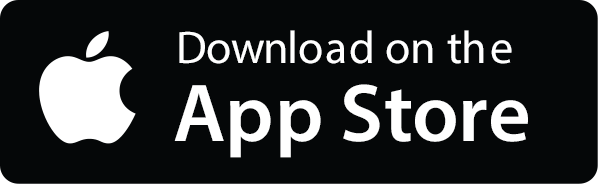
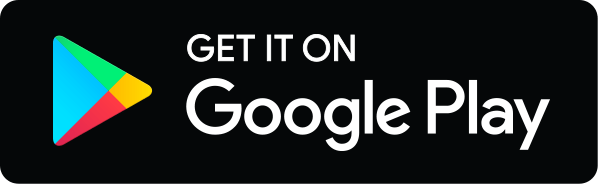
