Chapter 23 Principles and Techniques of Cardiac Catheter Mapping
Significance of the Local Electrogram
The intracardiac EGM is a graphical representation of localized cardiac electrical activity occurring in the region of the recording electrodes. From a purely signal-processing perspective, the EGM is determined as the instantaneous difference between signals recorded from two electrodes, at least one being intracardiac, and the second either also intracardiac (bipolar configuration) or positioned more remotely (unipolar configuration), for example, in an intravascular position, or connected to the “Wilson” central terminal or system ground. The “local” myocardial region contributing to the EGM is relative, related to the distance between recording electrodes as well as the specific recording configuration. More closely spaced electrodes are generally insensitive to far-field components and improve the fidelity of the near-field signal but at the cost of signal amplitude; wider-spaced electrodes, however, will detect signal from a larger region and record a greater amplitude, but they may dilute the local signal detail with detected far-field elements (Figures 23-1 and 23-2).
Utility of the Unipolar Electrogram
The minimally filtered unipolar EGM manifests an initial positive deflection when a propagating signal approaches the exploring electrode and then a rapid negative deflection as the signal moves away from it, which results in an rS, or RS, morphology. When the exploring electrode is positioned at or beyond a nonconductive boundary (e.g., within scar), such that the signal can only move toward the recording electrode but then cannot continue to propagate past it, the unipolar EGM assumes an essentially upright R, or Rs, morphology. As the exploring electrode is moved within “range” or close to an arrhythmogenic focus, the amplitude and duration of the initial positive deflection decreases, and the negative deflection predominates. When the exploring pole is positioned at an arrhythmogenic focus (e.g., origin of focal atrial tachycardia [AT]), or close to a nonconductive boundary such that the signal can only propagate away from the electrode (e.g., VT exit site or accessory pathway insertion), a QS morphology EGM results with a rapid initial downstroke (Figure 23-3, A; Figure 23-4). The maximum negative slope (intrinsic deflection) of the unipolar EGM coincides with depolarization of tissue directly beneath the recording electrode.1 As such, the unipolar EGM may provide information on proximity to an arrhythmia focus because of this sensitivity to far-field signals, and so may be iteratively evaluated to navigate toward and identify an arrhythmogenic focus or site of early activation. Although the unipolar EGM encodes whether the wavefront propagation is toward or away from the exploring electrode, it cannot further distinguish the specific direction of wavefront propagation.
While applicable for mapping focal tachycardias, unipolar recordings are somewhat limited in evaluating macro–re-entry when the circuit is entirely contained within a single chamber, as wavefronts arriving from all sites in the circuit would be expected to produce R waves.2
Utility of the Bipolar Electrogram
Bipolar EGMs are obtained from two exploring electrodes in relative proximity, each recording in unipolar configuration (see Figure 23-3, B). The lower-frequency components of the unipolar signals are contributed by essentially the same far-field signal and are canceled when their difference is obtained. However, the instantaneous local signals under each electrode differ, primarily in local activation time, voltage and frequency response, and propagation characteristics, generating a bipolar EGM with one or more rapid deflections (Figures 23-4 and 23-5).
Utility of Simultaneous Unipolar and Bipolar Recordings in Focal Tachycardias
As stated, the amplitude and duration of the R wave of the unipolar EGM decrease with proximity to a tachycardia focus, and the intrinsic deflection of the unipolar EGM correlates well with local activation under the exploring electrode. A QS morphology of the unipolar EGM results when recording at the site of depolarization of a tachycardia origin. However, the timing of the maximal negative slope in the minimally filtered unipolar EGM is often difficult to gauge visually, and QS waves with lesser dV/dt may be seen a distance from a tachycardia focus.2 The peak of the first deflection of the bipolar EGM also correlates well with local activation, and the prematurity of the bipolar EGM with respect to the surface ECG tachycardia beat may be used to identify a presumed tachycardia focus.3 When the onset of the surface ECG wave is difficult to identify, assessing prematurity of the bipolar EGM may become unreliable, confounding the determination of proximity to a tachycardia focus. Instead, the unipolar EGM may be used to identify a tachycardia focus by identifying sites at which a QS morphology in the unipolar EGM coincides with the peak of the first deflection of the simultaneous bipolar EGM, as this obviates reliance on the surface ECG (Figure 23-6). The earliest sites obtained with bipolar mapping are associated with the shortest interval between the onset of the unipolar signal and the first peak of the bipolar signal, with successful ablation sites exhibiting intervals under 15 ms.2
Factors Affecting the Local Electrogram
Anisotropic Conduction and Electrocardiogram Morphology
Anisotropic conduction refers to preferential longitudinal conduction that is observed in adult cardiac myocytes.4–6 In mature muscle cells, activation wavefronts may propagate across intercellular junctions both longitudinally and transversely. Because of the elongated configurations of ventricular myocytes, however, wavefronts propagating transversely encounter comparatively more intercellular junctions and, thus, travel more slowly than wavefronts moving an equal distance longitudinally. Therefore, normal myocardial conduction is described as uniformly anisotropic, with advancing wavefronts that are smooth in all directions but slower transversely than longitudinally, resulting in teardrop-shaped isochrones (Figure 23-7, A).
The “normal” ventricular EGM has been characterized during sinus rhythm in people with normal left ventricular function and without known structural heart disease. Normal bipolar EGMs obtained using multipolar catheters with 10-mm spacing are typically greater than 3 mV and of less than 70 ms duration without splitting, fractionation, or late components, though with somewhat lower amplitude and longer duration at the cardiac base.7
However, normal hearts do not exhibit “normal” EGMs and “normal” conduction patterns at all sites. Anisotropic conduction can become nonuniform in the absence of overt structural heart disease. Such anisotropy may be functional, related to anatomic and histologic barriers, borders, or alterations and varying orientations of overlapping myofibers. These histo-anatomic variances may predispose to local differences in signal characteristics, which, when detected in bipolar configuration, are then extracted and preserved, producing EGMs with multiple rapid deflections similar to those observed in patients with known structural heart disease.8
With advancing age, myocardial disease (e.g., myocardial infarction [MI]) and iatrogenic alterations in cellular architecture (e.g., prior ablation, maze procedure, external beam radiation), and associated deposition of connective tissue primarily along the longitudinal axis of cardiac fibers, lateral impulse propagation encounters greater resistance and becomes extremely slow and irregular (see Figure 23-7, B).4–6 This remodeling of cellular interconnections and electrical uncoupling produces heterogeneous regions of anisotropic slow conduction with reduced voltage and frequency response to excitatory stimuli, resulting in low-amplitude, fractionated EGMs with prolonged duration (Figure 23-8).
Catheter Mapping: Integral Components
Use of Electrode Catheters
The recording of local activation signals during tachycardia is obtained through both stationary and roving electrode catheters. Standard diagnostic multipolar electrode catheters are introduced percutaneously, and the obtained signals—unipolar or bipolar EGMs—are displayed in real time on a digital recording system. Stationary electrode catheters may be positioned in any cardiac chamber and are typically maintained at a fixed site for the duration of the diagnostic evaluation. For safety and convenience, sites accessible transvenously via the right cardiac chambers are chosen, usually the high-lateral right atrium (approximating the site of sinus endocardial breakthrough), His bundle region (approximating the site of atrioventricular node [AVN] conduction), within the coronary sinus (CS) (approximating a posterior location and a right-left axis of activation of both the left atrium and the left ventricle), and the right ventricle (Figure 23-11). This “standard” catheter positioning approximates the normal conduction system axis and creates a skeleton of recording sites that defines the sequence and timing of activation from all four cardiac chambers, creating a fixed framework within which the tachycardia may be assessed. Other recording sites may be sampled as well to further enhance the diagnostic framework on the basis of the suspected arrhythmia.
Cardiac Anatomy and Implications for Mapping
Knowledge of recording sites is critical to guide mapping as EGMs are interpretable only when paired with location. Decisions regarding how to proceed with mapping, that is, where to map next or in which direction the sought target is expected, cannot be made without knowing the current catheter positions. Therefore, competent catheter mapping requires basic awareness of fluoroscopic anatomy for proper EGM interpretation. Conversely, fluoroscopy may belie the true location of catheters, given its inability to detect depth, but EGM evaluation may confirm catheter location, as specific anatomic sites are associated with distinguishing EGM patterns or morphologies such that the local anatomic region may be inferred from the recorded signals (Figure 23-12). Sites with distinctive bipolar EGM patterns include the valve annuli, atrial appendage, crista terminalis, His bundle, CS, and the junctions between the cardiac chambers and the vascular tree (Figure 23-13).
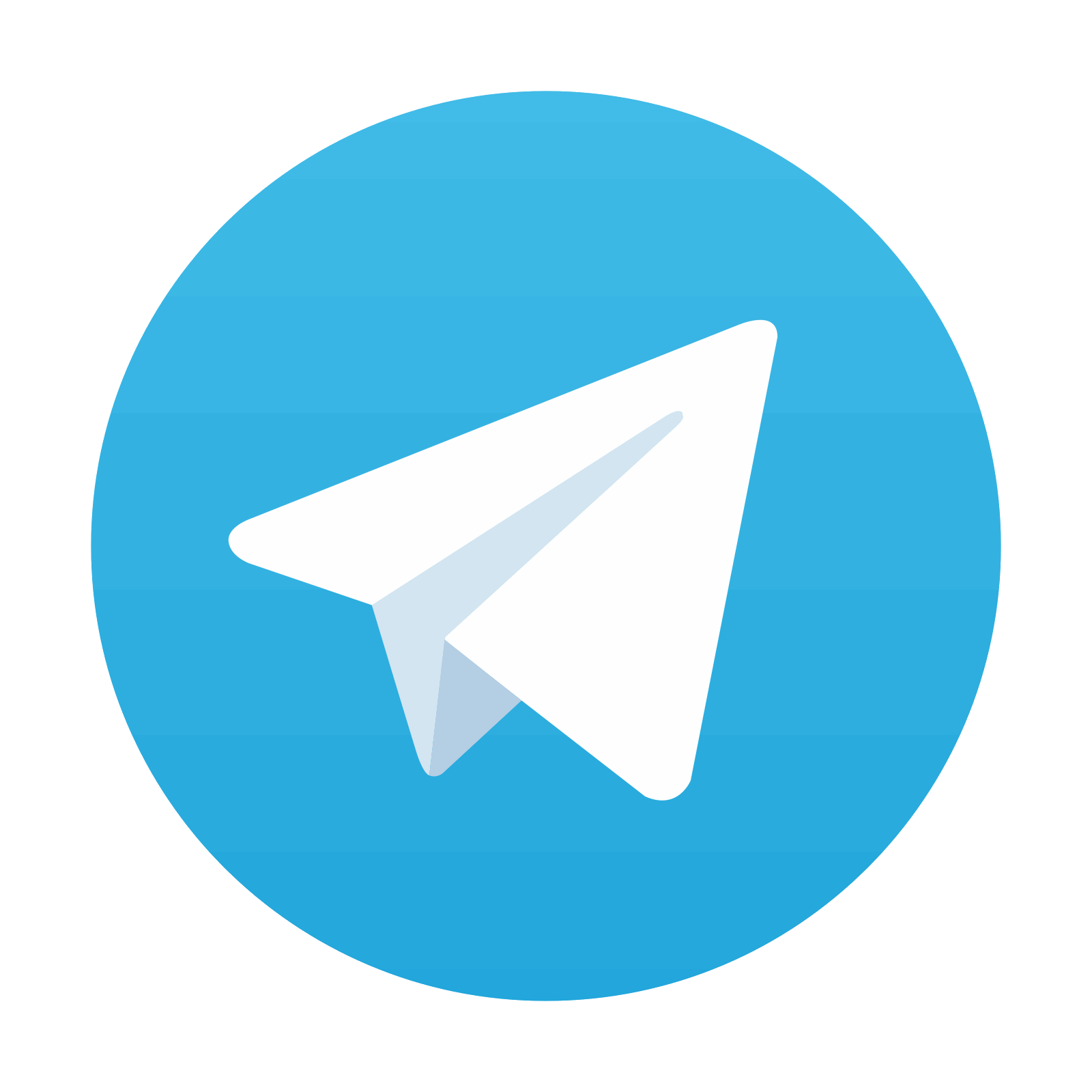
Stay updated, free articles. Join our Telegram channel

Full access? Get Clinical Tree
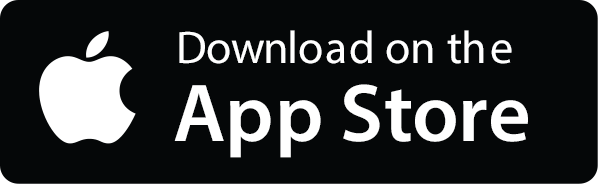
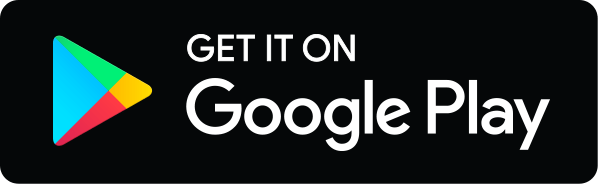