Chapter 74 Assessment and Treatment of Fetal Arrhythmias
The assessment and management of the human fetus with an arrhythmia is an area of medicine that presents many complexities. Because the fetus is hidden within an environment that is accessible only through remote means, direct knowledge of the condition and stability of the fetus is often in question. Current noninvasive and safe methods for fetal assessment, largely dominated by echocardiography, are limited and need to be expanded. The arrhythmias that are being treated are only those that are known at present. Potentially diagnosable and treatable intrauterine electrophysiological conditions may exist entirely hidden from the scope of perinatal medicine as it is practiced today.1–6 Many arrhythmias can be diagnosed by pulsed Doppler, tissue Doppler, and M-mode echocardiographic assessment of the atrioventricular (AV) relationship; however, the ventricular and atrial rate echo techniques do not measure the electrophysiological activity of the fetal heart but, rather, the mechanical consequences of that activity.7 Even the most sophisticated echocardiographic techniques cannot measure cardiac intervals (PR, QRS, QT duration) or repolarization characteristics. Nor is it possible to evaluate the fetus continuously over time, such as is done with ambulatory electrocardiography (ECG) monitoring. Despite these shortcomings, with the application of newer technologies such as fetal electrocardiography (fECG) and fetal magnetocardiography (fMCG) and more evolved echocardiographic techniques such as tissue Doppler, current understanding of arrhythmia mechanisms and the natural history of arrhythmia disturbances in the fetus has greatly expanded.8–15 Hidden fetal arrhythmias may contribute to the high rate of intrauterine fetal demise, unexplained nonimmune hydrops, and prematurity to a greater extent than has been appreciated. This chapter will focus on what is known at present about the assessment and treatment of fetal arrhythmias. It is divided into sections covering bradycardias, tachycardias, ectopy, diagnostic techniques, and management of the high-risk fetus.
Electrophysiological Diagnostic Techniques
Fetal Echocardiography
Fetal echocardiography provides many of the views necessary to risk-stratify patients with fetal arrhythmias; however, it is limited by the fact that it measures mechanical events only, and this can overestimate the P-R interval while underestimating the severity of fetal disease related to acquired and congenital depolarization or repolarization abnormalities. Echocardiography also can provide individual rates for atrial or ventricular rhythms; however, trending of these rates over time and catching rapid changes in rate and rhythm are difficult with echocardiography. Until techniques used below become more available, echocardiography remains a mainstay of diagnosis. Many excellent reviews have been written describing techniques for echocardiographic assessment of fetal arrhythmias.16–21
Fetal Magnetocardiography and Electrocardiography
Many experts believe fMCG to be the most reliable method for the full analysis of fetal arrhythmias because it can provide real-time telemetry as well as signal-averaged waveform (see Figure 74-13).2–6,10,12,22–32 While these technologies are not readily available in most institutions, current biotechnology development projects funded by the National Institutes of Health (NIH) will likely bring fMCG to common use within the next 3 to 5 years. Several fECG devices are available on the market worldwide. The ECG devices can provide up to 24 hours of rhythm recordings and a signal-averaged ECG waveform (provided the rhythm is stable). Acquisition success using the device is variable, and during the period from 26 to 36 weeks, when vernix is present, electrical insulation of the fetus leads to a poor signal-to-noise ratio. In this setting, the echocardiograms, nonstress testing, and biophysical profiles are usually used, but abnormalities seen in any one of these three may lead to early delivery. The addition of fECG or fMCG might reduce the need for some early deliveries by providing reassuring heart rate reactivity trends, Q-T intervals, and QRS durations.
Fetal Bradycardias
The standard obstetric definition of fetal bradycardia is a sustained (>10 minutes) fetal heart rate (FHR) of less than 110 beats/min with the usual heart rate ranging between 110 and 180 beats/min. However, as gestation progresses, basal FHR decreases significantly—from a mean and range of 141 beats/min (135 to 147 beats/min) at less than 32 weeks, to 137 beats/min (130 to 144 beats/min) at 37 to 40 weeks.33 Thus, the absolute value of the FHR cannot be deemed normal without considering gestational age. For example, a basal FHR of 125 beats/min at 32 weeks is in the tenth percentile, while the same rate at 25 weeks is well below the first percentile. The “low” FHR early in gestation, which does not meet the classic definition of bradycardia and “normalizes” in later gestation, has been seen in fetuses with long QT syndrome (LQTS).
The most prevalent mechanisms of bradycardia in the fetus are sinus bradycardia, blocked atrial bigeminy, and AV block. The echocardiographic presentation, incidence, and relevance of the different mechanisms of bradycardia are shown in Table 74-1. In studies, approximately 70% of fetuses with bradycardia referred to either fMCG or echocardiography had AV block, 10% to 20% had blocked atrial bigeminy, and 10% to 20% had sinus bradycardia. Of those with sinus bradycardia evaluated by fMCG, 40% had LQTS (Cuneo, AHA November 2009, unpublished data).34
Sinus Bradycardia
Sinus bradycardia can also be secondary to inflammation, fibrosis, and damage to a developmentally normal sinus node caused by viruses or maternal Sjögren’s antibodies.35 An extreme form of sinus node fibrosis, that is, complete replacement of normal atrial tissue with fibrotic cells, was reported in a fetus presenting with atrial standstill and bradycardia with a junctional escape rhythm.34 However, the most common cause of sinus bradycardia with a structurally normal or abnormal heart is vagally mediated bradycardia from fetal head or umbilical cord compression. If undue pressure is placed on the maternal abdomen by the transducer during ultrasound studies, transient but rather profound bradycardia can occur, but it disappears immediately when the pressure is relieved. Persistent sinus bradycardia can indicate fetal distress from hypoxia caused by placental insufficiency or cord compression. Other causes of sinus bradycardia are fetal hypothyroidism, central nervous system (CNS) abnormalities, intrauterine growth restriction, and maternal treatment with β-blockers.36
Fetal sinus bradycardia has also been reported in congenital sick sinus syndrome and, in the absence of AV conduction system disease, in fetuses with maternal Sjögren (SSA/Ro, SSB/La) antibodies.37,38 The causative role of a sodium channelopathy in both diseases is supported (1) by the demonstration of sinus node dysfunction in fetal hearts perfused in vitro with anti–52-kD SSA antibodies from mothers whose children developed AV block and (2) by the fact that recessive mutations in the sodium channel gene SCNA are found in congenital sick sinus syndrome.37,39
Persistent bradycardia, a marker in infants and children younger than 3 years for congenital LQTS, is also the most common presentation in fetuses with LQTS.40,41 After 30 weeks’ gestation, the fetal heart rate over time can be reactive or nonreactive during nonstress tests (Figure 74-1). Numerous mutations in the genes coding critical ion channel subunits have been reported to cause congenital LQTS, but 60% of affected individuals will have mutations in five genes coding critical ion channel subunits. The etiology of the bradycardia is purported to be either an autonomic imbalance with deficient sympathetic activity or a pathologic mutation reducing the activity of a G-protein mandatory for the coupling of β-adrenergic and sinus node receptors.42 Thus, signal transduction at the sinus node is impaired, which, in turn, affects the functioning of the pacemaker current, If.43
It has been reported that neonates with sinus bradycardia are more likely to have KCNQ1 mutations (LQTS1), while those who manifest 2 : 1 second-degree AV block are more likely to have HERG mutations (LQTS2).44 Further recognition and characterization of fetal LQTS is necessary to determine if the genotype can be predicted on the basis of clinical presentation before birth.
Long QT Syndrome
While LQTS is not always associated with fetal bradycardia, this is the most common fetal presentation. In addition to sinus bradycardia, congenital LQTS can also manifest as intermittent or persistent torsades de pointes (TdP), second-degree AV block, or both. All manifestations of congenital LQTS, including T-wave alternans, have been described by fMCG (Figures 74-2 to 74-5).1,6,45 The more widespread use of noninvasive recording may enhance the ability to identify individuals with de novo mutations associated with fetal arrhythmias, especially fetal bradycardia, as well as to recognize the presence or absence of Q-T abnormalities in pregnancies of affected family members.1,2,28 The normative data for Q-T intervals in utero as well as in the preterm infant have recently been reported.46 Values for term infants have been previously reported and corrected Q-T intervals greater than 0.48 seconds at birth have been associated with a 41-fold increase in the incidence of sudden infant death syndrome.40,47 Preliminary data suggest that a QTc of more than 580 ms before birth correlates with the presentation of TdP and 2 : 1 second-degree AV block either prenatally or postnatally (Cuneo, AHA November 2009, unpublished data).
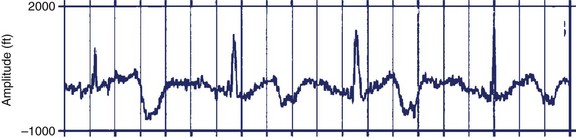
FIGURE 74-4 Rhythm strip showing macroscopic T-wave alternans during sinus bradycardia in the 32-week-old fetus with congenital long QT syndrome type 3 described in Figure 74-3.
It is important to consider a diagnosis of congenital LQTS using the same criteria applied to a child or a young adult, recognizing that established normative data for LQTS are based on only a very limited number of fetuses. Further, Zhao and colleagues recognized that the rate dependence of the Q-T interval on cycle length is much stronger in the fetus than in children of other ages.5 The mother should be questioned for a history of stillbirths at whatever gestational age, as fetal loss may be associated with familial mutations.48 The cardiac registry, which is composed mostly of patients with LQTS type 1 (LQTS1), did not find a higher association with miscarriage, but this may have been caused by bias introduced by the inclusion of extended families and LQTS1. If the specific familial mutation is known, prenatal genetic diagnosis of at-risk fetuses is possible from genomic deoxyribonucleic acid (DNA) extracted from cultured amniocytes.49
Blocked Atrial Bigeminy
A common cause of bradycardia in the fetus is blocked atrial bigeminy, or nonconducted premature atrial contractions. Occasionally, at first, the fetus will have atrial ectopy and an irregular rate, but at other times a persistent bradycardia will be present. Typically, the rate detected is lower than that in sinus bradycardia but faster than that in complete AV block (see Table 74-1).26 During blocked atrial bigeminy, a normally conducted atrial contraction is followed by a premature atrial contraction, which finds the AV node refractory and unable to conduct the impulse. Thus, every other atrial contraction is conducted (see Figure 74-5). This rhythm can persist for many weeks or for only a few hours. The management of blocked atrial contractions is the same as that of atrial ectopy: weekly or biweekly fetal heart rate auscultation to ensure that supraventricular tachycardia (SVT) has not developed. Isolated blocked atrial bigeminy does not require antiarrhythmic treatment in utero. Like atrial ectopy, blocked atrial bigeminy can be associated with SVT. The incidence of associated SVT is about 0.5% to 3%.50 The most important point to keep in mind about blocked atrial bigeminy is that it should not be mistaken for either sinus bradycardia caused by fetal distress, resulting in an unnecessary emergency cesarean section, or AV block, which has a very different prognosis and management protocol.51,52
Atrioventricular Block
Diagnosis and Etiology
Fetal AV block is secondary to either a congenitally malformed conduction system associated with complex structural cardiac defects or immune or infectious damage to a morphologically normal conduction system.53,54 The hallmark of high-grade (second-degree or third-degree) AV block is an atrial rate more regular and faster than the ventricular rate, ventricular bradycardia, and AV dissociation. AV block can be suspected during fetal heart rate auscultation as either an irregular rhythm (type 1 or intermittent type 2, second-degree AV block) or as bradycardia (type 2, second-degree AV block with 2 : 1 conduction, or third-degree AV block), and confirmed by M-mode or pulsed Doppler echocardiography (see below). Fetal heart rate auscultation cannot detect first-degree AV block; rather, this can be diagnosed on the basis of a prolonged mechanical P-R interval (>150 ms) measured by simultaneous mitral inflow and aortic outflow pulsed Doppler or simultaneous interrogation of the superior vena cava (SVC) and the aorta from the so-called three-chamber view (Figure 74-6).7,55
Using either technique, type 1, second-degree AV block can be distinguished from second-degree AV block with 2 : 1 conduction, and third-degree AV block (Figure 74-7).
Atrioventricular Block with a Congenitally Malformed Conduction System
The cardiac defects are complex because embryologically the right and left chambers are malaligned with the inflow and outflow portions of the heart. Because of this, a discontinuity between the AV node and the conduction system exists. The discontinuity of the conduction system results in AV block. The AV block has been reported to progress from second degree to third degree during gestation, but this has not been corroborated by fECG or fMCG. In a recent large series of 35 cases of heterotaxy diagnosed over a 10-year period, AV block was seen in 12 (55%) of 22.56 Of fetuses diagnosed with AV septal defects, approximately 20% will have LAI, and of those diagnosed with AV block, approximately 50% will have LAI.56,57
In addition to the complex structural cardiac defects and the conduction system abnormalities, the myocardium in LAI has an unusual spongiform texture, similar to ventricular noncompaction (VNC). VNC can be identified echocardiographically in the left ventricular (LV) apex and mid-portion as numerous excessively prominent trabeculations with deep intertrabecular recesses that communicate with the ventricular cavity. This primary myocardial abnormality results in abnormal systolic and diastolic function.58
The prognostic factors for prenatal survival have been evaluated in several studies. In a series of 31 fetuses, 22 pregnancies were interrupted and 3 fetuses died in utero.59 Of the 6 live-born infants, 2 survived. Five hydropic and 3 non-hydropic fetuses were given transplacental sympathomimetic therapy, which successfully augmented the fetal heart rate (FHR) but did not improve the hydropic fetuses. Interestingly, the atrial rate and not the ventricular rate predicted the development of hydrops with lower atrial rates having a worse prognosis (but neither the ventricular rate nor the atrial rate was associated with outcome in this series). Hydrops and cardiomegaly were associated with a poor outcome: Every fetus with a cardiothoracic (CT) ratio of more than 61% died. In another large series, hydrops and a low ventricular rate (<60 beats/min) were associated with a poor prognosis.60 Of 11 fetuses with LAI in continued pregnancies, only 1 survived to 1 year, despite in utero sympathomimetic therapy. A third study again demonstrated that the findings of AV block and anomalous pulmonary venous connections had the highest association and postnatal death in fetuses diagnosed with single-ventricle physiology.61
Prenatal diagnosis has not been shown by any study to make a difference in outcome (except for elective termination of pregnancy). The outcome of LAI and AV block is bleak: Even with in utero terbutaline treatment to augment ventricular rate and prompt pacing in the newborn period, the postnatal mortality in most series exceeded 50%.56,57,59–66 In the United States, terbutaline is generally the β-sympathomimetic of choice because it is available for oral as well as parenteral administration. Salbutamol can be administered only by the inhalation or oral route.
In contrast to extensive data on the fetal presentation and outcome of LAI and AV block, only limited data on AV block and congenitally corrected transposition of the great vessels are available. These data suggest that AV block is not a common presentation of congenitally corrected transposition. Additionally, once detected, the in utero survival is high: Of 17 reported cases detected in utero, all survived the prenatal period, and only 1 died during epicardial pacemaker implantation.5,59,60,67–69
Atrioventricular Block with a Structurally Normal Conduction System
The other major category of AV block that develops during fetal life occurs in a structurally normal conduction system. In the vast majority, the block is secondary to immune-mediated inflammation and fibrosis of the fetal conduction system from maternal SSA (Ro) antibodies, SSB (La) antibodies, or both.35,70–72 These antibodies cross the placenta and result in AV block in 2% to 3% of lupus pregnancies. If a previous child has been affected, the incidence increases 10-fold.71 A developmental susceptibility to the immune-mediated effects on the conduction system appears to exist in the fetus: AV block is generally not seen before 18 weeks, and onset is rare after 28 weeks. This appears to be related to the timing during which immunoglobulin G (IgG) antibodies cross the placenta. About 50% of all cases develop in mothers who are asymptomatic and unaware that they carry the SSA antibodies, SSB antibodies, or both.73
In addition to AV conduction disease, patchy areas of echogenicity (by echocardiography) seen in the ventricular endocardium, in the chordae of the AV valves, and in the atrial septum and free walls can be seen with or without AV block. Histologically, these areas represent endocardial fibroelastosis (EFE), which may be a precursor to dilated cardiomyopathy, the most dreaded sequela of immune-mediated fetal cardiac disease. Dilated cardiomyopathy has a high mortality rate without transplantation. Endocardial fibrosis and dilated cardiomyopathy can develop with or without conduction system disease.74–78 Other manifestations of SSA-mediated or SSB-mediated cardiac disease include sinus node dysfunction, bundle branch block, and a late-onset rupture of the AV valve chordae.38,79
The diagnosis of fetal SSA-mediated or SSB-mediated AV block is made on the basis of an increased titer of maternal SSA or SSB antibodies. Prospective evaluation of pregnancies complicated by maternal SSA or SSB antibodies using weekly echocardiographic measurement of the mechanical P-R interval has not successfully identified an orderly progression in the fetus, from first-degree through second-degree to third-degree AV block. Rather, as one study showed, third-degree AV block with ventricular dysfunction developed in less than 1 week, and first-degree AV block identified in the third trimester did not progress.80 Rein and colleagues recently reported that first-degree AV block could be reversed if recognized.81 Similarly, progression from second-degree AV block to third-degree AV block did not occur in fetuses evaluated longitudinally by fMCG (see Figure 74-13).5 Thus, it seems that the clinical phenotype of SSA-mediated or SSB-mediated disease varies from normal rhythm followed by sudden progression over a few hours to severe conduction system and myocardial disease and to a more indolent course. Either mild low-grade conduction system disease is seen or, as some believe, slow progressive conduction disease.81
If AV block is detected in the fetus and the maternal SSA or SSB antibody titers are negative, a channelopathy may be the cause. Both NKX2.5 mutation and SCN5A mutation have been linked to progressive AV block. Other clinical manifestations of these mutations include cardiomyopathy (Lenegre-Lev disease, caused by SCN5A mutation) and congenital heart disease. Questioning the family may elucidate a history of congenital heart disease, conduction system disease, sudden death, or cardiomyopathy in first-degree relatives of the fetus.82–85
The electrophysiological characteristics and in utero history of AV block have been elucidated by fMCG in a large study of 28 fetuses.5 About 30% of fetuses had other complex and unsuspected arrhythmias, including ventricular tachycardia (VT) and junctional ectopic tachycardia (JET). JET occurred in the mid-second trimester and decreased in rate and duration as gestation progressed. VT occurred at any time during gestation. Isolated ventricular ectopy and atrial ectopy were also common and seen in 70% of fetuses.
Although it is generally assumed that during fetal AV block the ventricular rate is monotonous, two distinct patterns of FHR acceleration exist. In a study, the first, a nonreactive pattern (Figure 74-8, A), was seen in fetuses who were paced as neonates on the basis of standard recommendations.86 The second, a reactive pattern of acceleration (Figure 74-8, B), predicted freedom from pacing for at least 4 months after birth. Additional information about patterns of acceleration in AV block was detected by fMCG evaluation of fetuses with low ventricular rates augmented by terbutaline.87 In this study, heart rate patterns of acceleration differed based on the etiology (associated with LAI, or SSA-mediated or SSB-mediated damage) of the AV block.
Unsuspected repolarization abnormalities have also been identified by fMCG in a high proportion of fetuses with conduction system disease, including prolonged QTc interval and T-wave alternans. QTc interval prolongation has been identified in a much higher proportion of fetuses than in newborns with isoimmune disease.6
Treatment and Outcome of SSA-Mediated or SSB-Mediated Atrioventricular Block
Fetal survival and 1-year survival of patients with AV block diagnosed in utero has greatly improved over the last 20 years (Table 74-2).69,88–94 Improved survival can probably be attributed to a combination of factors, including earlier identification of at-risk fetuses and more vigilant follow-up during pregnancy. In addition, the improvements in perinatal care of high-risk fetuses have allowed many without risk factors for intrauterine demise to be managed to term or nearly to term despite very low FHRs. The benefits of transplacental pharmacologic treatment have not been proven in a prospective randomized trial. Treatment options include β-agonists such as terbutaline to augment the fetal ventricular rate; dexamethasone, a fluorinated steroid, which crosses the placenta and appears to reduce inflammation; and intravenous immunoglobulin (IVIG), which decreases the amount of circulating maternal antibodies.95–99 In anecdotal experience, IVIG was given to fetuses with severe EFE or cardiac dysfunction who did not respond to fluorinated steroids.33 The ability of IVIG to prevent progression in at-risk pregnancies is also under study; however, outcomes have been mixed, with better outcomes seen in those receiving higher doses or more frequent doses. Its use for prophylaxis remains unproven as yet.70,71 Risks to the mother and the fetus are mainly from exposure to blood products. Terbutaline appears to be well tolerated, with no serious sequelae in most mothers and fetuses.87
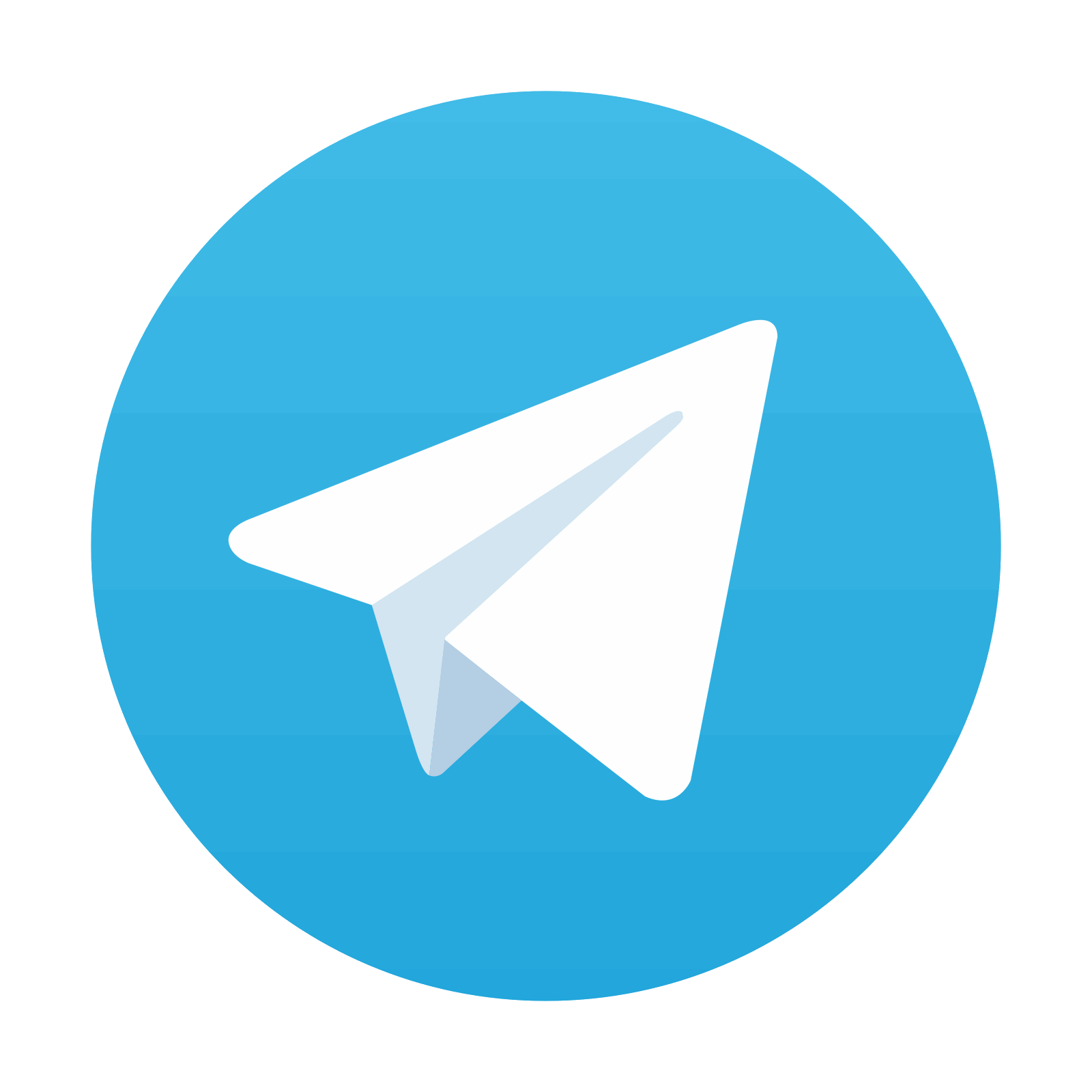
Stay updated, free articles. Join our Telegram channel

Full access? Get Clinical Tree
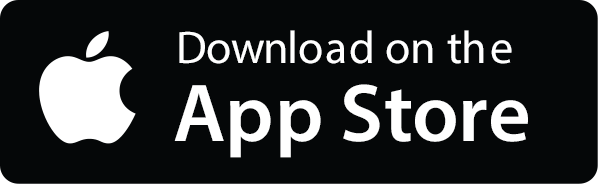
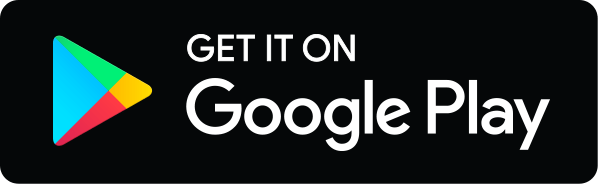