Chapter 16 Ablation Technologies and Delivery Systems
Comparison of Ablation Technologies
Each energy source used for catheter ablation has particular characteristics that determine how the energy interacts with myocardial tissue. As seen in Table 16-1, each technology is distinguished by the characteristics of the energy source. In RF energy, the 300- to 700-kHz energy source is produced by an RF generator that uses resistive heating. In contrast, the 915 to 2450 mHz microwave energy produced by a microwave generator is absorbed by substances on the basis of their di-electric properties, resulting in increased molecular motion that translates into tissue heating. Because of the differences in frequency, microwave energy uses an antenna operating at the delivered frequency, whereas RF energy may be delivered from an electrode. Cryothermia relies on the extraction of heat rather than its delivery and usually is the result of a pressure drop of a suitable gas such as nitrous oxide. Laser energy may be delivered at a range of frequencies, most commonly from 300 to 2000 nm. Each wavelength has specific absorption properties that will determine its effect on blood and body tissue. The wavelength of light is delivered via a fiberoptic, usually at a pinpoint. The fiber may be coupled to a diffusing element, which will activate to deliver the energy over a specific region, permitting the energy delivery to be adapted to the target. Ultrasound energy may be delivered using a piezo-electric crystal that converts electrical energy to mechanical energy.
The ability of each energy source to affect the properties of the ablation is illustrated in Table 16-2. Only cryothermal energy can reliably cause reversible effects for true mapping. By reducing tissue temperature to a level above freezing, it may be possible to have the bulk of the tissue be reversibly affected. Heating also has some reversible effects, but it is difficult to avoid irreversible cell death. RF energy and cryothermia are nearly completely dependent on tissue contact, whereas microwave energy, laser, and ultrasound may be delivered without tissue contact. Lesion size is considerably variable across energy sources, but in general, RF energy and cryothermia are believed to produce smaller lesions for a given maximal surface area of contact. Ultrasound and laser permit focusing of energy, which may permit controlled delivery to a specific location or even depth.
The biologic effects of the energy sources are described in Table 16-3. Heating occurs by different mechanisms, depending on the energy source. Microwave energy is absorbed by tissue, causing increased molecular motion, particularly of water molecules, which results in heating. Laser energy has several effects, including heating, vaporization, and coagulation. Ultrasound energy acts by sonication, boiling of water, and tissue heating. Cryothermia acts by its freezing the tissue, which produces ice crystals and a change in the osmotic properties of tissue. With thawing of the ice crystals, further damage and rupture of the cell may occur. Cryothermia also disrupts the vascular supply to the tissue. Overall, cryothermia is felt to result in the greatest preservation of the architecture of tissue. Cryothermia also causes the least destruction of the endothelial surface, which may help reduce the incidence of thrombus formation. Although the risk of esophageal damage is largely unknown, studies indicate that esophageal damage resulting in fistula formation may be less with cryothermia compared with other energy sources.
A summary of the advantages and disadvantages of each energy source is given in Table 16-4. RF energy has been extensively used clinically, and its relative safety has been demonstrated. The need for tissue contact and the ability of impedance rise to limit lesion formation has helped prevent complications and the destruction of collateral tissue. Microwave energy permits a variety of antenna configurations to be developed and does not require tissue contact, although the distance between the antenna and the tissue must be held relatively constant for constant energy delivery. Cryothermia’s greatest advantage is the minimal disruption of tissue architecture and the endothelium and its reversible effects. In addition, tissue adherence permits excellent contact. Laser energy is capable of giving energy rapidly to create large-sized lesions and can be controlled. Ultrasound energy is capable of creating larger lesions and may permit combined imaging.
Table 16-4 Advantages of Energy Sources
ABLATION TECHNOLOGY | ADVANTAGES | DISADVANTAGES |
---|---|---|
Radiofrequency | Proven safety; limited ability to cause collateral damage | Limited lesion size; requires contact; charring |
Microwave | Contact not required; antenna configuration may be varied for different applications | Requires control of distance to keep energy delivery constant; antenna must be efficiently coupled to tissue; heating of cable |
Cryothermia | Reversible; improved safety; minimal endothelial disruption; adheres to tissue | Slow energy delivery; reversibility at margins; inability to drag lesion |
Laser | Rapid delivery of high energy; large lesions; directional | No self-limiting feedback for energy delivery; risk of excessive energy delivery |
Ultrasound | Large lesions; heats tissue more than blood; may permit imaging | Difficulty controlling depth; dependent on tissue properties |
Ablation Technologies
Cooled Radiofrequency Ablation Systems
An example of the currently available internally cooled RF ablation system is the Boston Scientific (Natick, MA) Chilli Catheter (Figure 16-1). Clinical trials using this system have demonstrated that this catheter may be used effectively during the ablation of ventricular tachycardia (VT).
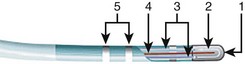
FIGURE 16-1 Saline-irrigated radiofrequency ablation catheter with internal cooling (Chilli ablation catheter).
(Courtesy Boston Scientific, Natick, MA.)
Externally cooled RF ablation systems use small holes in the ablation electrode to permit saline to escape the catheter and be introduced into the body. Because a return path is not required, current designs permit the forward flow to be greater compared with internally cooled RF ablation systems. In addition, it is possible that the saline that escapes into myocardial tissue directly cools the myocardium in addition to cooling the ablation electrode. Present designs incorporate several holes in the electrode that are able to cool the electrode efficiently. The Biosense Webster (Waterloo, Belgium) Thermocool (Figure 16-2) catheter and the St Jude Medical (St Paul, MN) catheter (Figure 16-3) have lateral holes at the catheter electrode. The Thermocool catheter has been approved for ablation of atrial fibrillation (AF) and VT.
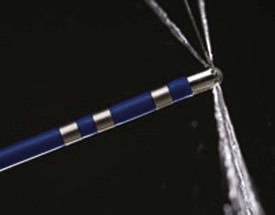
FIGURE 16-3 Saline-irrigated radiofrequency catheter with external cooling (St Jude Medical, St Paul, MN).
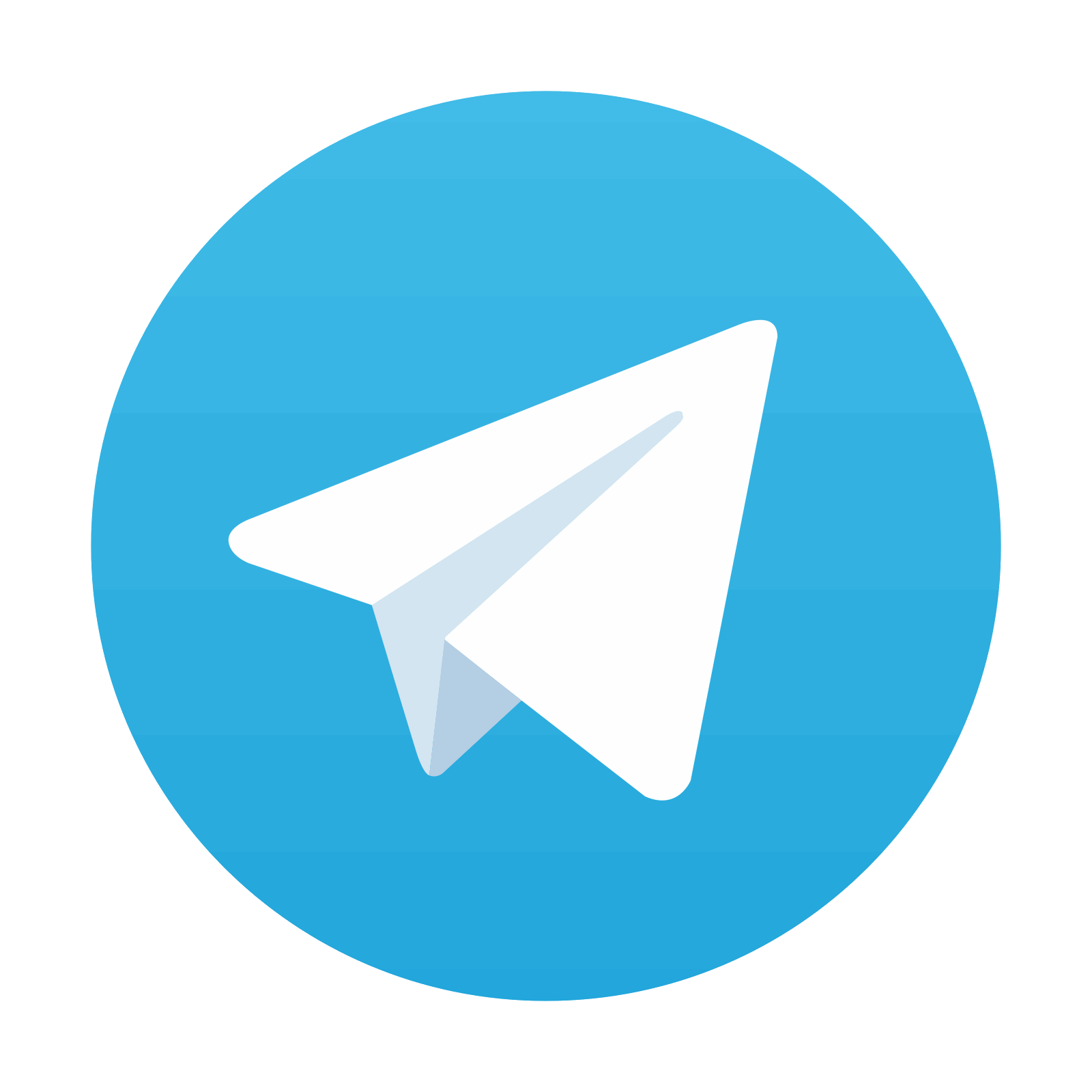
Stay updated, free articles. Join our Telegram channel

Full access? Get Clinical Tree
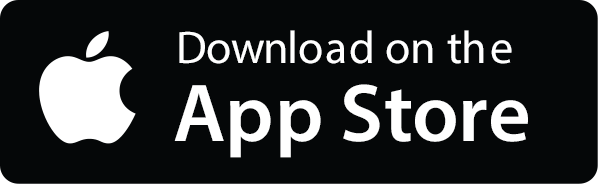
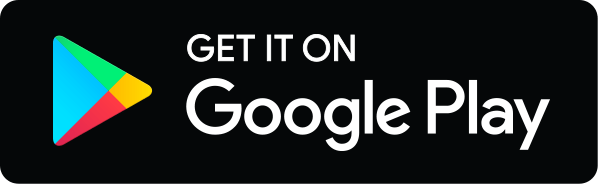