2 An ultrasound beam is a continuous or intermittent train of mechanical or pressure waves emitted by a transducer or wave generator. These waves can exist in any solid medium (i.e., not in a vacuum.) As the waves travel past any fixed point along an ultrasound beam, the pressure cycles regularly and continuously between a high and a low value. The number of cycles per second (Hertz [Hz]) is called the frequency of the wave. Perceptible sound waves have frequencies from 20 to 20,000 Hz. Ultrasound is sound with frequencies above 20 kilohertz (kHz), while medical ultrasound often uses frequencies between 2 and 12 megahertz (MHz). In addition to frequency, ultrasound waves are characterized by their wavelength and velocity. 1 Wavelength is the distance between the two nearest points of equal pressure or density along an ultrasound beam, and velocity is the speed at which the waves propagate through a medium. The relationship among the frequency (f), wavelength (λ), and velocity (v) of an ultrasound wave is defined by the formula: Ultrasound waves transport energy through a given medium; the rate of energy transported per time is expressed as “power” (P), which is expressed in joules per second or watts. 1 Since medical ultrasound is normally concentrated in a small area, the strength of the beam is usually expressed as power per unit area or “intensity” (W/m2). In most circumstances, intensity is expressed with respect to a standard intensity. For example, the intensity of the original ultrasound signal may be compared with the reflected signal. Since ultrasound amplitudes may vary by a factor of 105 or greater, amplitudes are expressed using a logarithmic scale, the decibel, which is defined as: where P1 is the power of the wave to be compared, and Pref is the power of the reference waves. The three-dimensional shape of the ultrasound beam is dependent upon both the physical aspects of the ultrasound signal and the design of the transducer, especially its aperture. Further details of ultrasound transducers will be discussed in Chapter 5. An unfocused ultrasound beam may be thought of as an inverted funnel where the initial straight columnar area is known as the “near field” (also known as the Fresnel zone), followed by a conical divergent area known as the “far field” (also known as the Fraunhofer zone) ( Fig. 2-1). The length of the near field is directly proportional to the square of the transducer diameter and inversely proportional to the wavelength; specifically: where Fn is the near-field length, D is the diameter of the transducer, and λ is the ultrasound wavelength. where D is the diameter of the transducer.
Principles and Physics
Principles of Ultrasound
Ultrasound Beam
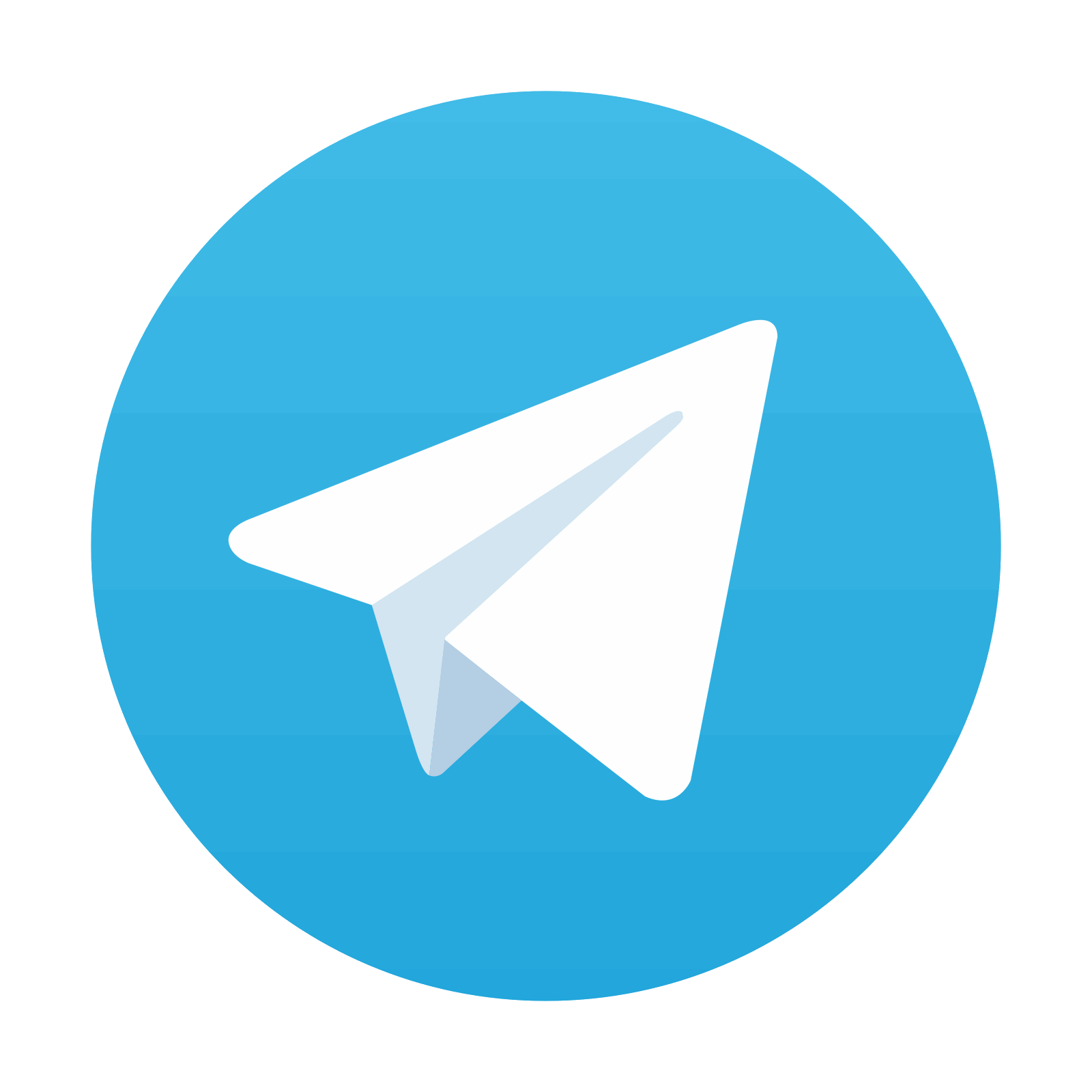
Stay updated, free articles. Join our Telegram channel

Full access? Get Clinical Tree
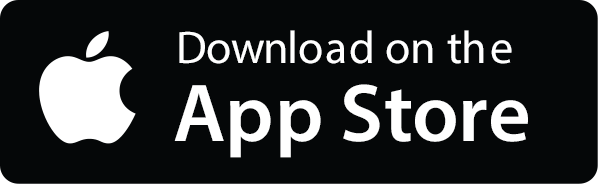
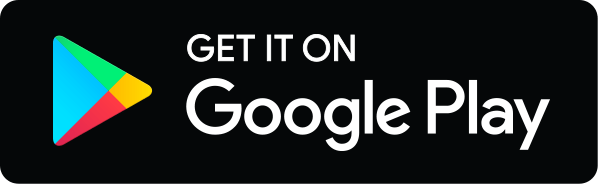