Fig. 28.1
Effects of exercise on cardiovascular risk factors. HDL high-density lipoprotein cholesterol, TG triglycerides, sd-LDL small-dense low-density lipoprotein cholesterol, HTN hypertension
Blood Pressure
Exercise plays a significant role in improving blood pressure control [30]. A recent meta-analysis of 54 randomized controlled trials comprising 2,419 subjects demonstrated reductions of both systolic and diatolic blood pressure with aerobic exercise regardless of baseline blood pressure, obesity, weight loss achieved, frequency or intensity of exercise [31]. However, other studies have demonstrated relatively greater blood pressure-lowering effects from exercise in hypertensive subjects compared with normotensive subjects [32].
Even small improvements in blood pressure can impart large improvements in clinical outcomes; a 2 mmHg reduction in diastolic blood pressure can reduce the incidence of CHD by 6 % and stroke or transient ischemic attack by 15 % [33]. The beneficial effects of aerobic exercise on blood pressure are mediated in part through changes in skeletal muscle conductance and peripheral resistance, mediated both by structural changes in resistance vessels, as well as by reducing sympathetic nerve activity [34]. Resistance training is also beneficial, particularly because it promotes weight loss and enhanced aerobic activity [35].
While salt reduction may provide synergistic BP-lowering benefit, studies analyzing exercise and diet have not always demonstrated the anticipated BP-lowering efficacy of combined therapy. It has been observed in individuals who report higher levels of physical activity their blood pressure was less sensitive to the effects of salt intake [36]. Habitual exercise was equivalent to diet in lowering blood pressure and the combination had no greater reduction [37]. A study of 4,820 normotensive adults showed greater onset of hypertension among those with a lower level of fitness [38]. Therefore, exercise not only moderates hypertension but also protects against its incidence.
Lipids
Exercise has beneficial effects upon atherogenic lipid profiles, yet the magnitude of these effects is not straightforward. An older meta-analysis of 95 studies demonstrated reductions in total cholesterol of 6.3 %, LDL cholesterol of 13.4 %, and increases in HDL of 5 % with exercise [39]. However, since many of these exercise interventions were accompanied by weight loss, the weight change may have been the primary determinant of the lipid shifts [40]. There also appears to be a synergistic effect between exercise training and diet on improving lipid profiles, especially when there is an associated weight loss [41, 42]. Combining therapies result in the greatest reduction in weight, but lesser improvements in HDL levels than the exercise group as the low-fat diet also produced HDL-lowering effects [43].
A meta-analysis of 51 studies, 28 of which were randomized controlled trials, differentiated between exercise alone vs. exercise combined with diet interventions [44]. In the 2,200 subjects without concomitant dietary interventions, there were more consistent effects of exercise on lipid levels with increases in HDL cholesterol, however, reductions in total cholesterol, LDL cholesterol and triglycerides were more variable between studies. The largest randomized trial to examine the relationship of exercise and lipid levels, the HERITAGE Family Study, demonstrated increased HDL levels, but no significant reduction of total cholesterol or LDL levels [45]. Triglyceride reduction tends to be greatest in those with elevated baseline values and also those with higher baseline insulin resistance [46, 47]. Overall, exercise alone has modest effects on standard lipid parameters, with both HDL-increasing and triglyceride-lowering effects, but with more substantive LDL-lowering and total cholesterol-lowering changes achieved by the combination of exercise training and dietary therapy.
Increasing attention has been given to the relationship of lipid subfractions to risk of CV disease, with smaller, denser LDL particles being more atherogenic than the larger and more buoyant ones [48–51]. Despite minimal direct effects of exercise on LDL cholesterol levels, exercise training produces larger mean LDL particle size, reduced concentrations of small-dense LDL, and decreased LDL particle number [52]. Higher amounts of exercise are also associated with increases in large HDL and HDL2 particles [53]. While the clinical benefit of these exercise-induced lipid changes is usually assumed, confirmation with randomized controlled trials is still needed.
Insulin Sensitivity
Physical activity has many beneficial effects on glucose homeostasis, including decreased hepatic glucose production and improved insulin resistance [54]. Exercise improves glucose disposal and reduces insulin resistance in skeletal muscle, primarily by inducing increased glucose transport protein GLUT 4 [55] as well as the activity of hexokinase and glycogen synthase [56, 57]. In addition, glucose delivery accelerates amidst increased muscle blood flow and capillary density [57, 58]. The improvements in insulin resistance have observed with both vigorous (≥6 METS) and non-vigorous activity (<6 METS) [59].
Acutely, 45–60 min of moderate-intensity exercise lowers plasma glucose by approximately 20–40 mg/dL in subjects with type II diabetes mellitus [60]. Boulé et al. reviewed 14 studies of exercise interventions of at least 8 weeks in duration in diabetic patients and found that exercise was associated with 0.66 % average reduction in hemoglobin A 1C , independent to exercise intensity or volume, or to the amount of weight loss [61]. Notably, these exercise benefits approach the reduction in hemoglobin A1C that was achieved with intensive glycemic control (compared to conventional treatment) in the United Kingdom Prospective Diabetes Study [62].
Obesity
Assuming diets are similar, subjects engaging in relatively greater amounts of leisure time physical activity are less likely to be overweight [63] as more calories are burned. However, the relationship between exercise and weight loss is usually more complex than this simple principle implies. Exercise alone has only modest effects on weight loss, generally a 2–3 kg reduction, but the addition of exercise to dieting is more likely to achieve substantial weight reductions [62, 64]. In addition, increased physical activity is associated with reduced weight gain in healthy populations as well as improved maintenance of weight loss in adults who were previously obese [65, 66]. The weight loss associated with exercise may also preferentially affect visceral adiposity, which may be a stronger risk factor than overall adiposity [67, 68]. Current recommendations from the U.S. Department of Health and Human Services for physical activity and weight management suggest approximately 60 min of moderate to vigorous weight bearing (e.g., a treadmill rather than a cycle ergometer) intensity exercise on most days of the week for weight maintenance in adulthood, and 60–90 min of moderate intensity activity for sustained weight loss, though these targets may be extremely difficult to achieve for most individuals [69].
Although there has traditionally a focus on aerobic exercise to promote weight loss based primarily on the principle of burning calories, strength training has synergistic effects [70]. Strength training increases skeletal muscle mass, and thereby induces higher resting energy expenditure [71, 72]. A study of aerobic exercise vs. strength training in conjunction with caloric restriction found similar reductions in body weight, visceral, and subcutaneous adipose tissue in both groups [73] and strength training was as effective at reducing body weight as aerobic exercise while muscular strength also increased [74]. This combined effect can also promote increased aerobic capacity, essentially compounding training benefits by increasing submaximal walking time to further increase caloric expenditure and promote weight loss [75]. Overall, strength and aerobic exercise are well validated as combined therapy to reduce obesity and overall body fat [76].
Inflammation
A recent study of 27,000 healthy women reported that differences in cardiovascular risk factors accounted for 59 % of the risk reduction associated with aerobic exercise [77]. Metabolic syndrome is also closely linked to inflammation either as a cause or consequence of the pathophysiological process [78, 79]. Multiple epidemiological studies have demonstrated that higher levels of inflammation are associated with a greater risk of subsequent CV events in subjects with and without the metabolic syndrome [80–84]. Increased exercise has consistently been associated with reduced inflammation as measured by C-reactive protein (CRP) or other inflammatory markers [85–93], although some of these studies have attributed the reduced inflammation to exercise-associated weight loss [85, 88, 91, 94]. Other large population-based cross-sectional studies have demonstrated that higher levels of self-reported leisure time physical activity are associated with lower levels of CRP, even after multivariable adjustment for other cardiovascular risk factors including BMI [86, 89, 92, 93]. The British Regional Heart Study examined the relationship between changes in levels of reported physical activity over 20 years and current levels of inflammatory markers [92]. Men who were initially sedentary but who subsequently became active had lower CRP levels than those who were persistently sedentary or those who were initially active and became sedentary.
The DNA Polymorphisms and Carotid Atherosclerosis (DNASCO) study, a large randomized trial, examined the relationship between exercise and inflammation as a secondary endpoint [90]. In this study, 140 middle-aged Finnish men were randomly assigned to an individualized aerobic exercise program or usual activity for a 6 years period. While ventilatory aerobic threshold increased in the intervention group, CRP only trended lower with exercise at all time points, likely due to insufficient power to detect differences in CRP levels.
Even patients with known cardiovascular disease can achieve reduced inflammation with exercise training [87]. Milani et al. demonstrated that a 3 month of phase II rehabilitation resulted in improvements in peak oxygen consumption, as well as significant reductions in CRP levels that were greater than those observed for a control population [87]. These improvements were also seen among those who failed to lose weight and were similar between users and non-users of statin medications.
Oxidative Stress
Oxidative stress can be most simply understood as an imbalance between the production and consumption of reactive oxygen species within the body which cause cellular damage. Increased formation of reactive oxygen species indicating elevation in oxidative stress have been observed in a variety of cardiovascular diseases [95]. During resting conditions free radicals are generated at a low rate and controlled by antioxidant systems, but strenuous exercise increases oxidative stress acutely in the body due to an elevated metabolic rate and increase in oxygen consumption which may exceed the capacity of the cellular antioxidant homeostasis capacity [96]. Oxidative stress also tends to accumulate as a function of mounting chronic diseases and age, particularly as natural homeostatic mechanisms tend to erode over time. Although exercise acutely increases oxidative stress, adaptive mechanisms to chronic exercise reduce oxidative stress responses in as few as 12 weeks [97].
Cardiomyocyte senescence, defined by decreased telomere length and increased oxidative stress injury [98]. Whereas telomeres usually provide the genetic template to facilitate homeostatic repair from oxidative stress, shortened telomeres with age essentially determine the reduced capacity to counterbalance oxidative stress risk [99]. Exercise and caloric restriction are two mechanisms combat cumulative reactive oxygen species and offset typical aging risks [100]. Exercise increases volume of mitochondria with related augmentation of oxidative ATP synthesis and reduced reactive oxygen species production [101]. Oxidant scavengers can also increase [102].
High caloric intake may also be harmful in respect to oxidative stress as it suppresses “longevity genes” that promote cellular defenses against aging and age-related disease [103]. Markers of oxidative stress were significantly reduced with an intervention of a low fat diet and 45–60 min of exercise daily [104]. Antioxidant enzymes are inducible and adapt to chronic exercise over time to reduce oxidative stress by achieving a favorable balance between pro-oxidants and antioxidants [105]. A key point is that while calorie restriction may provide important anti-oxidant benefits in relation to age, it is still essential that a diet be replete with vital nutrients. Calorie restriction implies restricting high caloric foods that lack healthful properties.
Metabolic Syndrome
The metabolic syndrome is a clustering of atherosclerotic risk factors characterized by hypertension, abdominal obesity, dyslipidemia and insulin resistance [106]. Approximately 27 % of the US adult population is affected, and the prevalence continues to increase amidst the growing epidemic of obesity [107] and also as a consequence of predominant aging (and related tendencies to be sedentary). The risk of coronary artery disease is increased in those with metabolic syndrome, such that risk is usually greater than the sum of individual risk factors [108–110].
Exercise improves all components of the metabolic syndrome, particularly the characteristic pattern of dyslipidemia (elevated triglycerides and low HDL cholesterol), abdominal obesity, and insulin resistance. Improved cardiorespiratory fitness also diminishes the incidence of the metabolic syndrome [111] and attenuates its deleterious effects on CV mortality. In one study of 19,000 white men, subjects with the metabolic syndrome had a relative risk of CV mortality of 1.89 compared to healthy men [112]. After adjusting for level of fitness based on maximal oxygen uptake on a treadmill exercise test, these differences in mortality were no longer observed. Furthermore, the death rate of individuals with the metabolic syndrome who were fit (11.9 per 10,000 person-years) was almost one-third the rate of unfit subjects with the metabolic syndrome (31.0 per 10,000 person-years), and comparable to the death rate of unfit subjects without metabolic syndrome (19.0 per 10,000 person-years).
Insulin resistance is thought to be one of the principle pathophysiologic factors underlying the metabolic abnormalities in the metabolic syndrome [106]; adults with metabolic syndrome have a greatly increased risk of developing diabetes mellitus [108]. Moreover, utility of exercise to moderate the progression to diabetes was demonstrated in the Diabetes Prevention Program study [113]. Subjects with impaired fasting glucose were randomized to a lifestyle intervention consisting of dietary modifications and increased moderate intensity physical activity, metformin therapy, or placebo. The mean body mass index in these subjects was 34 kg/m2 and baseline levels of physical activity were 15.5–17 MET-h/week. After approximately 2.8 years, subjects in the lifestyle intervention group had an average 5.6 kg reduction in body weight and an 8 MET-h/week increase in leisure time exercise. More importantly, these subjects had an impressive 58 % reduction in the incidence of new onset type II diabetes, which was considerably greater than the 31 % reduction in subjects receiving metformin.
Sleep Quality
Individuals with poor sleep hygiene are at increased risk of heart disease. A recent review of studies involving nearly a half million people determined that short duration of sleep was associated with a greater risk of developing or mortality due to CHD [114]. The relationship was U-shaped with the lowest risk being in individuals with 7–8 h of sleep per night but with greater risk associated with too little or too much sleep. A number of mechanisms may mediate the harmful effects of too little sleep, particularly increased coronary calcifications. King et al. examined the development of coronary calcifications in subjects without any at baseline over 5 years and observed greater incidence of calcifications among those who slept the shortest time [115]. Another study demonstrated that individuals who sleep poorly have higher levels of C-reactive protein, another known risk factor for CHD [116].
Exercise may often improve sleep quality. A randomized controlled trial of moderate-intensity exercise training for 16 weeks in 67 subjects aged 50–76 who were sedentary and without cardiovascular disease improved self-rated sleep quality [117]. A study of 3,081 adults in the NHANES database between 2005 and 2006 who exercised for at least 150 min per week at a moderate level had a 45 % reduction in poor sleep quality [118]. In another study 32 individuals with depression were randomized to supervised weight training and the intervention resulted in improved sleep quality, depression, and quality of life scores [119].
Additional Mechanisms of Exercise Benefit
While many describe exercise benefits in terms of performance indices, exercise also fundamentally changes morphology and physiology that determine physical function as well as predisposition to disease. These mechanisms are complex but can be conceptualized in terms of central, peripheral, and neural components.
1.
Central – coronary perfusion (endothelial function, intrinsic flow as well as differences in capillarity and coronary artery caliber), cardiac output (stroke volume, inotropy, chronotropic responses), cardiac compliance, and ischemic conditioning of the myocardium.
2.
Peripheral – skeletal muscle, peripheral vascular dynamics (endothelial function, vessel distensibility), ventriculo-arterial coupling
3.
Neural – Autonomic function, sympathetic activation
Central Exercise Training Effects
Vascular Dynamics in Coronary Arteries
Substantial evidence has accumulated that exercise training leads to increased vascular responsiveness mediated primarily by increased formation of endothelium-dependent nitric oxide [120–122]. Exercise improves endothelial function by inducing increased production and phosphorylation of endothelial nitric oxide synthase (eNOS) in response to higher shear stresses, with increased nitric oxide production (Fig. 28.2) [122].
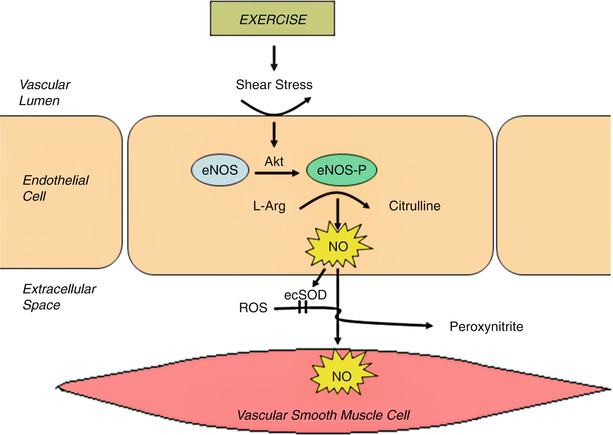
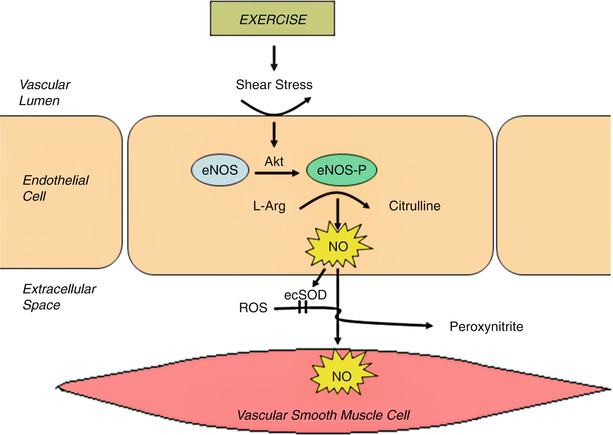
Fig. 28.2
The effects of exercise on endothelial function. Exercise induced shear stress results in phsophorlyation of endothelial nitric oxide synthase (eNOS) by serine/threonine kinase, Akt. Nitric oxide (NO) is generated which has beneficial effects on the endothelium and vascular smooth muscle, and is broken down by extracellular superoxide dismutase (ecSOD). L-Arg L-arginine, ROS reactive oxygen species (From Gielen and Hambrecth [363]. Reprinted with permission from Elsevier)
Exercise induced improvements in endothelial function may be a principle mechanism for the improved myocardial perfusion and reductions in ischemia observed with exercise [123]. In a cohort of patients with CHD, Hambrecht et al. observed a 54 % reduction of acetylcholine-induced coronary artery vasospasm, which was significantly different than sedentary controls after a 4 week intensive aerobic training program [121]. In addition, after the administration of adenosine (to trigger endothelial-mediated responses), coronary blood flow reserve increased by 29 % and the coronary flow-mediated dilation by 105 % compared with pre-training measures, with little change seen in the controls.
While exercise-related endothelial-mediated vasodilation benefit many include increased coronary flow reserve that can limit exercise induced ischemia, thereby delaying the onset of angina and perhaps reducing the risk for sudden cardiac death, it does not thereby reduce the incidence of myocardial infarction. Marathon runners still develop and suffer the consequences of coronary atherosclerosis [1]. In fact, plaque rupture and related acute coronary events depends more on the composition of the atherosclerotic plaque, i.e., soft and lipid filled (rupture prone) vs. hard and collagenous (rupture resistant), than on the volume of plaque, degree of stenosis, and/or vasomotor capacity [124, 125]. Nonetheless, exercise also improves coronary plaque stability and reduces long term cardiovascular mortality through other mechanisms [126]. For examples, Lamonte and colleagues showed that even for the same amount of atherosclerosis as assessed by coronary calcium >100, the risk for CV events was reduced by 75 % in patients who were fit (exercise capacity >10 METS) compared to those that were unfit (<10 METS) [127]. Exercise-related reductions in macrophages and inflammation as well as increased smooth muscle plaque composition are among the key mechanisms by which this additional benefit may be achieved.
Exercise training increases coronary vascular transport capacity, both by structural and functional adaptations within the coronary vascular bed [128], resulting in improved myocardial perfusion (Fig. 28.3) [129, 130]. One mechanism of enhanced perfusion is an increase in coronary collateral vessels, however, there is conflicting evidence for this process occurring in humans [129, 131–136]. Two larger randomized trials of exercise interventions have presented opposite conclusions regarding the effects of exercise on collateral formation, which may reflect the dose of exercise and/or differences in the study populations. One randomized trial of 46 subjects with chronic CHD and left ventricular dysfunction showed that exercise three times weekly for 8 weeks promoted coronary collateral development which was more marked in subjects with more collaterals at baseline [129]. Although another study of men who underwent angiography for angina did not develop any new collateral coronary arteries by angiography after 1 year in those randomized to daily exercise or in the controls [135].
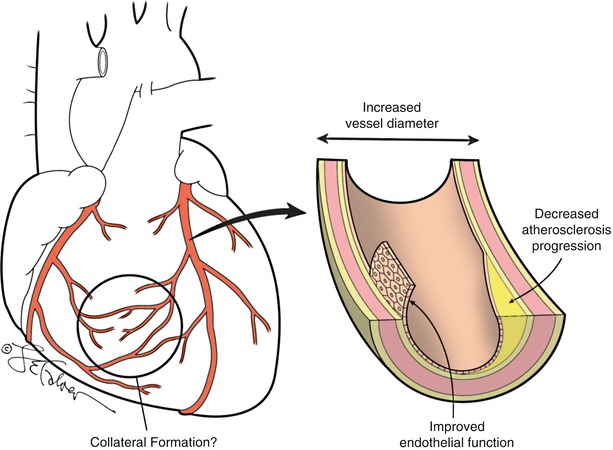
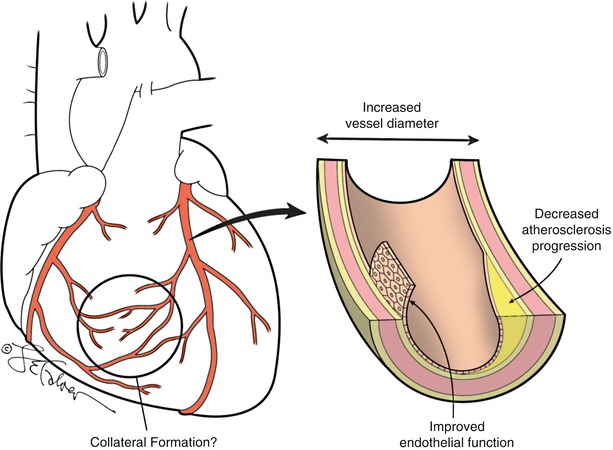
Fig. 28.3
Vascular effects of exercise. Exercise results in improved endothelial function, increased arterial vessel diameter, and may delay the progression of atherosclerosis and promote collateral vessel formation
Exercise may also increase coronary artery diameter and flow. Rodent studies suggest that endurance training lead to such increased coronary artery caliber and similar studies in dogs demonstrate larger epicardial coronary arteries, but it remains difficult to prove in humans [137–139]. Anecdotal reports [140], and autopsy studies in British citizens [140], and Masai tribesman [141] suggest that while atherosclerosis may develop in endurance trained individuals, exercise enlarges the coronary lumen preserving normal luminal volume and coronary blood flow. In contrast, Haskell et al. performed quantitative angiography in a group of ultra-marathoners who competed in races of more than 100 miles [142]. They found that at rest, the combined cross-sectional area of the right, left main, left anterior descending and circumflex coronary arteries was not larger than a group of control patients with chest pain but angiographically normal coronary arteries. However the lower myocardial oxygen consumption of the athletes during the study who had lower heart rates and lower blood pressure than the controls may have prevented the investigators from observing a greater coronary diameter [143]. More importantly, after the infusion of the NO donor nitroglycerin, the change in size of the coronary arteries was much larger, demonstrating much greater potential vasodilator capacity from “ultra” level marathon training.
The role of exercise may be particularly important in modifying the development and progression of atherosclerosis. In patients with and without known coronary artery disease, exercise can attenuate the progression of atherosclerosis, independent of its effects on other risk factors [90, 144, 145]. Hambrecht et al. studied a 1 year exercise program in patients with coronary artery disease and discovered that the exercise group had greater rates of coronary lesion regression and reduced rates of progression compared with the control population [144]. Similarly, in the DNASCO study, middle-aged men not taking statin medications that were randomized to an aerobic exercise program had 40 % less progression of carotid intima-media thickness after 6 years compared with those continuing regular activity [90]. Exercise may also moderate development of atherosclerosis in those without known vascular disease, as increased exercise was independently associated with a reduced prevalence of subclinical coronary atherosclerosis as measured by coronary artery calcium scanning [146].
Modified Myocardial Oxygen Demand
Improvement in symptoms is an important goal in patients with ischemic heart disease. An additional benefit of exercise training is improved myocardial work (Fig. 28.4) [147], both with increased oxygen uptake and utilization [148, 149]. A study of individuals who underwent exercise training had lower heart rates and blood pressure (lower myocardial oxygen consumption) during submaximal treadmill testing allowing the same external work to be accomplished with a lower myocardial work [150]. These patients also reported significantly reduced symptoms of physical exertion on the Borg scale after exercise training.
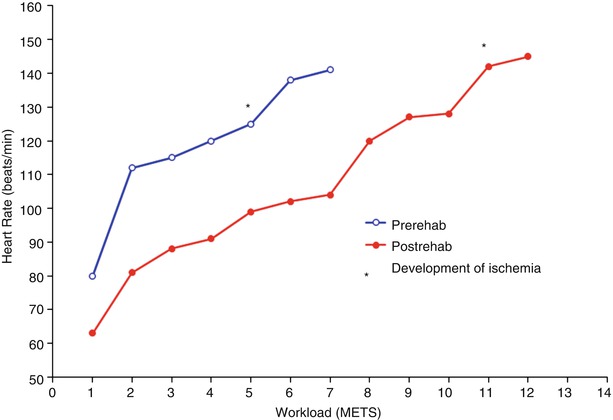
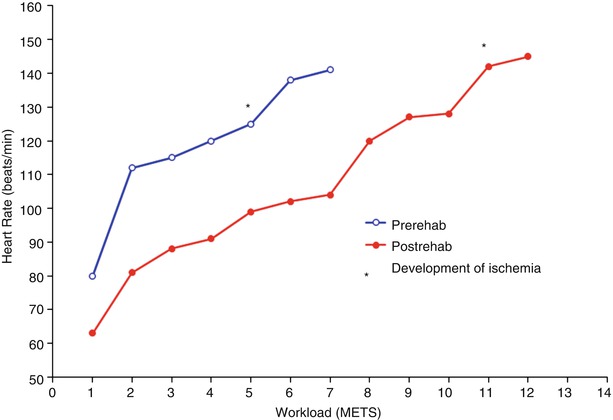
Fig. 28.4
Change in heart rate during a standard exercise test (Bruce protocol) in a patient with severe coronary artery disease, before (open circles) and after (closed circles) 12 weeks of cardiac rehabilitation. Ischemia, manifested by typical angina pectoris and significant ST segment depression developed at a relatively low workload (5 METS) before training. After training, ischemia does not develop until very high workloads are achieved (11 METS)
A number of central adaptations that occur in the myocardium may be responsible for this effect. Exercise training raises the ischemic threshold and decreases the amount of ischemia for any given workload (Fig. 28.4) [151]. The reductions in ischemia may be related to both reduced myocardial work (Fig. 28.4) or enhanced myocardial perfusion [130, 152], which is likely a product of improved coronary endothelial function [121] as opposed to increased coronary vessel structural caliber or collateral development as explained previously. The precise cellular mechanisms in the myocardium which protect against ischemia are not entirely understood. A recent review by Golbidi and Laher discusses the proposed exercise-induced myocardial mechanisms including decreasing reactive oxygen species (increased NO, mitochondrial adaptations, sarcolemmal Na/K ATPase) and repairing cellular damage (heat shock proteins, increased endoplasmic reticulum stress proteins, increased autophagy) [153]. Ultimately, these physiologic adaptations of exercise allow patients to perform activities of daily living as well as more vigorous activities with fewer symptoms [154]. One study compared the relative cardiovascular effects of exercise training vs. percutaneous coronary interventions (PCI) in a select group of 101 male patients with stable CHD symptoms and at least one significant coronary lesion on angiography [155]. After 12 months, subjects randomized to either therapy had significant improvements in anginal symptoms (change in Canadian Cardiovascular Society angina class 1.5–0.4 in exercise group; class 1.7–0.7 in PCI group). In addition, both groups had improved myocardial perfusion in the region of their coronary lesions on nuclear perfusion imaging, and increased ischemia thresholds. However, only the exercise training group had an increase in work capacity and maximal oxygen uptake.
When training is prolonged and intense, even patients with cardiac disease can achieve improvements in myocardial contractile function [156], possibly facilitating increased stroke volume and cardiac output [157, 158]. A study of patients with CHD and left ventricular ejection fractions ranging from 29 to 76 % (mean 52 %) who completed a 12 month endurance exercise training program compared and achieved significant improvements of their ejection fractions with smaller end-systolic volume compared to sedentary controls [156]. Exercise training can also lead to improved diastolic filling parameters that can also augment stroke volume and cardiac output. Levy et al. investigated the effects of endurance exercise training on diastolic function and discovered improved early diastolic filling at rest and during exercise which facilitates increased stroke volume and cardiac output [159]. However endurance training also increases the plasma volume so this observation may be simply due to changes in loading conditions. Conversely, life-long exercise training at a very high level does not alter Doppler measures of relaxation and diastolic filling when filling pressure is controlled [160].
Exercise training also leads to advantageous remodeling benefits. Among 90 patients with stable chronic heart failure due to systolic dysfunction, those who were randomized to a 6 month exercise training program demonstrated reduced left ventricular end-diastolic and end-systolic volumes and improved ejection fraction while controls had increased volumes and ejection fraction was unchanged [161]. In addition, life-long training in Masters athletes preserves youthful levels of cardiac chamber and myocardial compliance as directly measured from pressure-volume curves [162].
Peripheral Exercise Training Effects
Skeletal Muscle
In addition to central benefits of exercise training, peripheral effects on skeletal muscle and the vasculature are also important. Lean muscle mass plays a central role in metabolism and functional status. Loss of muscle mass is an determinant of survival in relation to age, disease, or even deconditioning [163]. Similarly, loss of muscle mass also contributes to development of frailty a concept which also includes elements of motor and cognitive slowing and even to increased incidence and/or progression of cardiovascular disease [164]. Therefore, exercise training to maintain lean muscle mass is an important goal for individuals with cardiovascular disease. Furthermore, sarcopenia is difficult to reverse once it is established and emphasis on prevention of is preferable [165]. Education and regular exercise at younger ages increases chances of optimal muscle health in old age.
Peripheral Vasculature
Endothelial dysfunction is a marker for cardiovascular risk that is commonly assessed as brachial flow-mediated dilation [166]. In a cohort of 3,026 adults in the Multi-Ethnic Study of Atherosclerosis (MESA) who were free of cardiovascular disease at baseline brachial flow-mediated dilation was a predictor of cardiovascular events [167]. Moreover, Clarkson et al. showed that this risk profile was modifiable; this study examined the effect of a 10-week aerobic exercise program in 25 healthy military recruits and observed improved endothelium-dependent dilation after exercise [168]. Gokce et al. also demonstrated improved flow mediated dilation even in CHD patients after 10 weeks of moderate intensity leg exercise training [169]. Just like with the myocardium, life-long training preserves youthful compliance of the large blood vessels, and reduces the effective biological age of the aorta by nearly 25 years compared to the chronological age [170]. Even though exercise training up to a year in duration may not change large vessel [171] or cardiac compliance [172], it does seem to improve ventriculo-arterial coupling [172, 173].
Systemic Exercise Training Benefits
Autonomic Modulation
Exercise also helps modulate autonomic tone which can significantly reduce vulnerability to arrhythmia and sudden death. Autonomic tone is the balance between sympathetic and parasympathetic efferent neural influences on the heart. In general, reduced sympathetic tone and increased parasympathetic tone helps mitigate genesis of cardiac arrhythmias [174, 175]. Consistently, carotid baroreflex control of heart rate, assessed by measuring the reduction in heart rate induced by infusion of phenylephrine (at a dose sufficient to raise arterial pressure) was shown by Billman et al. to be an important predictor of mortality after myocardial infarction [176].
Heart rate variability, a more general index of cardiac-vagal tone that is relatively easy to obtain using Holter technology, is reduced acutely in acute myocardial infarction [177], and is an important marker for late mortality after MI [178]. Depressed heart rate variability was the most powerful independent predictor of life-threatening arrhythmias in myocardial infarction survivors. When combined with late potentials on signal averaged ECG and repetitive ventricular ectopic forms on Holter monitoring, the combined assessment provided high prognostic sensitivity and specificity [179]. Moreover, these indices were superior to left ventricular ejection fraction, exercise ECG responses, and/or number of ventricular ectopic beats. Multiple studies of thousands of adults have shown low heart rate variability increases the risk for cardiovascular events and mortality [180, 181]. Animal studies have confirmed that exercise induced changes in autonomic control, by increasing vagal tone, may prevent ventricular fibrillation associated with myocardial ischemia and reduce the risk of sudden death [182, 183]. Similar benefits have also been demonstrated in humans (Fig. 28.5) [184].
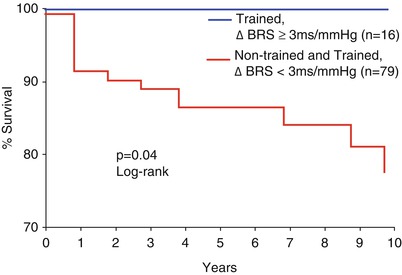
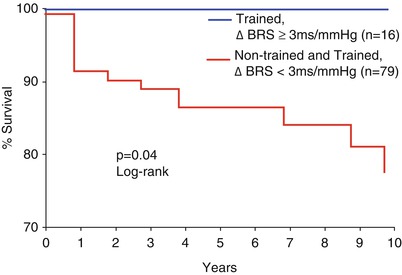
Fig. 28.5
Cardiac mortality and autonomic function. Kaplan-Meir estimates of cardiac mortality compared between subjects with an exercise training-induced increase in baroreflex sensitivity (BRS) =3 ms/mmHG compared with a group without training induced increases in BRS =3 ms/mmHG and all non-trained patients (From La Rovere et al. [184]. Reprinted with permission from Wolters Kluwer Health)
A number of clinical studies have demonstrated increases in heart rate variability with exercise training, including normal athletes [185–187], as well as patients with hypertension [188], congestive heart failure [189], or coronary heart disease [189, 190]. It appears that moderate amounts of exercise training may be optimal to achieve these autonomic benefits of exercise [190, 191]. On the contrary, prolonged bed rest deconditioning can reduce both heart rate variability and baroreflex sensitivity arguing strongly for early mobilization as a key part of convalescence [192]. Older individuals also tend to have depressed heart rate variability as a direct effect of aging, and may particularly benefit from exercise training, as decreased fitness with age may be at least partly responsible for the alterations in autonomic function in this population [193–195]. These studies support the hypothesis that changes in autonomic tone may be an important mechanism for the reduction in sudden death caused by exercise training. Finland et al. also demonstrated significant reduction in sudden death in a post-myocardial infarction population that was apparent within the first 6 months of the program [196]. The distinguishing feature of Finland’s study is that the patients were enrolled very early after their myocardial infarction, within 2 weeks of hospital discharge, timing that suggested that mortality benefits were primarily attributable to improvements in autonomic tone.
Coagulation
Advances in understanding regarding cardiovascular pathophysiology indicate that acute intravascular thrombosis underlies most episodes of myocardial infarction [197]. Such pathophysiologic centrality of thrombosis has led to significant interest regarding the effects of exercise on modifying the coagulation system. Notably, blood from animals that were run to death in the eighteenth century was recognized not to clot [198], with many thus assuming exercise may provide important anti-coagulant benefit. However recent data have been somewhat contradictory; effects of exercise appear to pivot between thrombotic and fibrinolytic promoters and inhibitors that may be modified by the intensity and duration of the exercise, and also in relation to the population being studied [199–203].
Acutely, high intensity exercise appears to actually increase concentrations of clotting factors, particularly Factor VIII [204, 205]. This increase, persists for at least 1 h, and probably does not occur until the level of exercise reaches a high enough intensity, i.e., 95–100 % of maximal oxygen uptake [206]. When high intensity exercise is sustained over more prolonged periods of time (up to 1 h), coagulation may be particularly activated, with increased formation of both thrombin and fibrin, possibly due to mechanical stimulation of endothelial cells [207]. While only heavy exercise is accompanied by a significant increase in coagulation factors, both moderate and high intensity exercise are associated with increased plasma fibrinolytic activity, which appears directly related to the intensity and duration of exercise [199–206, 208]. The mechanisms of this increase fibrinolysis appear to be via increases in endogenous tissue plasminogen activator (t-PA) activity and reductions in fibrinogen [92] and plasminogen activator inhibitor-1 (PAI-1) activity [209–211], probably mediated by increased circulating epinephrine [212].
Acutely, short-term strenuous exercise is associated with an increase in platelets, probably by a combination of hemoconcentration and aggregability [213, 214]. However, long-term moderate-intensity training decreases platelet adhesion and aggregability both at rest and that induced by exercise, and these adaptations revert back to baseline after deconditioning [213, 215, 216]. These observations may help to explain the early increased risk of myocardial infarction after heavy physical activity, particularly in sedentary individuals [217].
Primary Prevention of Ischemic Heart Disease
Physical Activity and Exercise
Physical activity is risk factor for development of cardiovascular disease and was first established in studies comparing activity levels during occupational activities. In the 1950s, Morris et al. first suggested that the rate of CHD was inversely related to the level of physical activity levels at work [218]. In the London transportation study, sedentary bus drivers had almost twice the incidence of CHD as compared to conductors who regularly walked up and down the stairs of double-decker busses [219]. Additionally, overall death rates, including sudden death, were twice as high in the drivers as compared to the conductors. Paffenbarger et al. showed that San Francisco Longshoremen who performed heavy physical labor, requiring bursts of energy of 5.2–7.5 kcal/min (equivalent to brisk walking, slow jogging, or activity such as shoveling snow – 1 kcal/min is roughly equivalent to 1 MET) with a mean energy expenditure of 1,876 kcal above basal requirements/workday, benefited from a 25 % reduction in coronary death rates over 16 years of follow-up compared to workers who were more sedentary (1.5–5.0 kcal/min maximal energy requirements during work) [220, 221]. Virtually every subsequent study comparing sedentary occupations to active ones has confirmed this protective benefit of regular occupational exercise [222]. Farmers in North Dakota [223], U.S. postal employees [224], American railroad workers [225], and participants of the Health Insurance Plan of New York [226] offered further support to the hypothesis that exercise protects against coronary disease. However confounding variables such as obesity, hypertension and hyperlipidemia made it difficult in these early studies to clearly demonstrate an independent effect of exercise. A more recent study of firefighters by Durand et al. determined that physical activity was associated with greater cardiorespiratory fitness after adjustment for age, obesity, and smoking status as well as more favorable lipid and glucose levels [227].
As mechanization and automation have reduced the energy expenditure of many workers on the job, more recent studies have focused on the beneficial effects of recreational activity. Several studies have demonstrated a reduction in total mortality and cardiovascular events with increasing leisure time physical activity [15–29, 228]. These salutary effects of exercise apply not only to men [18–20, 24–27], but also to women [16, 21, 22, 29] and the elderly [15, 17, 28]. In the Multiple Risk Factor Intervention Trial (MRFIT), moderate leisure-time physical activity in middle-aged men was associated with a 37 % reduction in fatal cardiac events and sudden deaths, and a 30 % reduction in total deaths as compared to lower activity [19]. Similarly, in both the Nurses’ Health Study of women age 40–65 and the Women’s Health Initiative of postmenopausal women, those in the highest quintile of physical activity had less than half the risk of subsequent cardiovascular events compared to those in the lowest quintile [21, 22]. In addition, diverse forms of leisure time physical activity including running, weight training, and walking have all been associated with lower risk of cardiovascular events [27]. In 2007 the physical activity of over 250,000 men and women in the NIH-American Association of Retired Persons Diet and Health Study was compared and those who were are least moderately active (30 min most days of the week) had a lower risk of cardiovascular mortality [229]. This protective benefit of physical activity may explain a substantial proportion of the difference in cardiovascular mortality seen between rural and urban populations, both by a global reduction of risk factors for coronary disease in rural populations [230].
A significant reduction in cardiovascular mortality can still be achieved even if regular activity is not begun until later in life [21, 28, 231]. For example, in 4,311 British men age 40–59 that had measures of physical activity 12–14 years apart, a change from a sedentary lifestyle to at least light activity resulted in a 34 % adjusted risk reduction in cardiovascular death [28]. Similarly, sedentary women aged 40–65 who became active over a 6 year period had an approximately 30 % reduction in cardiovascular events. Additionally, these benefits of increasing physical activity have even been observed in those greater than age 60 [231].
Sedentary Lifestyle Risk
Conversely, studies investigating a sedentary lifestyle have shown it is associated with an increased risk of cardiovascular mortality. Cassel et al. examined residents of Evans county in rural Georgia, and were able to show that a sedentary occupation was an independent risk factor for CHD [232]. Brunner et al. took advantage of the relatively homogeneous lifestyle of the Kibbutzim of Israel to minimize variability in diet, race, and socioeconomic status. In this population, the sedentary residents still demonstrated two to four times the incidence of CHD as their active counterparts over a 15 years period [233]. A recent survey of more than 120,000 US adults examining leisure time physical activity showed sedentary individuals who reported sitting >6 h per day vs. <3 h per day had higher association with mortality [234]. This association was even greater when >6 h per day sitting was combined with low physical activity, but time spent sitting was independently associated with mortality.
Fitness
Physical activity, exercise and fitness are not synonymous [235]. Physical fitness consists of physical attributes that people have or achieve, including muscle strength and endurance, flexibility, body composition and cardiovascular endurance [236]. Regular exercise is associated with physical fitness as exercise can facilitate improvement of fitness indices, for example, objective measures such as peak workrate on a treadmill or bicycle ergometer or directly measured peak ventilatory oxygen uptake [237, 238]. However, genetics also factors into fitness. Although there is significant variability among studies, one study of identical twins suggests that up to 40 % of fitness measures may be genetically determined. However, such genetic impact is greatest in younger ages [239]. As adults reach older age, lifestyle, exercise, and environment become relatively more dominant determinant influences of fitness. Moreover, physical activity and exercise tend to supersede fitness as the most significant factors underlying CV health. Being very fit but sedentary provides little protection against CHD or all-cause mortality [240]. Moreover, unfit but active men have a lower risk of CHD than fit, sedentary men [240]. Blair and colleagues have also demonstrated that individuals who increased their fitness from one follow-up period to another, experienced a decrease in cardiovascular mortality by more than 40 %, providing strong evidence that exercise training as a means to acquire or maintain fitness, rather than fitness per se, are the keys to reducing CV mortality and morbidity [241].
Fitness and physical activity are both independently associated with cardiovascular disease, although it is important to recognize that fitness can also be modified by physical activity [18, 238, 242–247]. In The Lipid Research Clinics Mortality study, 4,276 men were followed for an average of 8.5 years after a baseline exam which included a treadmill test [244]. An assessment of fitness was based on the cardiac workload generated during submaximal exercise (stage 2 of a Bruce protocol treadmill test) by a combination of heart rate and the duration of exercise. Subjects who had higher heart rates or shorter duration of exercise had a significantly greater incidence of cardiovascular death. Blair et al. examined physical fitness (maximal treadmill exercise test) and risk for all cause and cause specific mortality in 10,224 men and 3,120 women [245]. Cardiovascular and all cause mortality declined across higher quintiles of physical fitness in both men and women, even after adjusting for age, smoking, cholesterol, blood pressure and glucose levels, family history of CHD, and follow-up interval (mean 8 years). Aerobic capacity has also been shown to be comparable to many other traditional risk factors such as smoking, diabetes, and hypertension, in its relationship to mortality [243]. Even in those with a significant burden of subclinical atherosclerosis, a high level of fitness denotes a greatly improved cardiovascular prognosis relative to those who are less fit [127].
It is possible that lower prevalence cardiovascular risk factors and/or selection bias has contributed to the apparent protective advantage of exercise in many studies ostensibly studying survival benefits of physical activity and/or exercise. Most prospective epidemiologic studies have demonstrated consistent reduction in cardiovascular events with increasing physical activity, despite multivariable adjustment [18, 19, 21, 22, 27]. However, it is also possible that naturally robust persons are more likely to exercise or participate in sports than individuals predisposed to coronary artery disease. This question was addressed in a comprehensive investigation of 16,936 Harvard alumni aged 35–72 comparing past and present habitual energy expenditure and the risk of myocardial infarction and death [25]. Total and cardiovascular mortality were both decreased in those with greater recreational exercise, irrespective of the level of athleticism or sports participation during college. Ex-varsity athletes were at lower risk of cardiovascular disease only if they continued to exercise later in life suggesting that exercise itself, rather than a genetic predisposition to fitness was responsible for the protective effect. This value of habitual exercise over genetically endowed fitness was also confirmed with data from the Finnish Twin Cohort Study [248], in which nearly 8,000 pairs of twins were asked about their exercise habits in 1975, and then followed for 20 years. Among twin pairs who were healthy at the initial examination, and discordant for death at follow up (n = 434), the odds ratio for death was 0.44 among individuals who exercised with vigorous walking at least 6 times/month, compared to those who did not exercise regularly.
Intensity of Exercise
While some early studies suggest that only vigorous activity produces the beneficial effects of exercise on CV morbidity and mortality [18, 23, 24], others show benefits at all intensities. The optimal exercise regimen remains somewhat controversial. British male civil servants who performed at least brisk walking (>4 mph) or more vigorous sporting activities were demonstrated to have reduced coronary events, but no benefit was observed for those with more moderate levels of activity [24]. Similarly, in Finnish men, a reduction in the risk of myocardial infarctions was only associated with >2.2 h/week of conditioning activities such as swimming and bicycling [18]. No reduction in events was seen for durations of <2.2 h/week or for non-conditioning activities like yard work or low-intensity walking.
Nonetheless, in both the MRFIT trial and the British Regional Heart Study, moderate levels of physical activity (i.e., frequent walking and gardening or mean of 1,568 kcal/week, respectively) were associated with lower coronary heart disease events, but there were no further reductions seen in highest categories of exercise [20, 26]. The Health Professionals Follow-up Study, in contrast, demonstrated a graded effect between physical activity intensity and reduction in cardiovascular events, including those with high intensity exercise (>6 METS) [27]. After excluding those with greater than 1 h/week of vigorous exercise to minimize confounding, the relative risks of CHD for normal pace (2–3 mph), brisk pace (3–4 mph), and very brisk pace (>4 mph) walking compared to those who walked at an easy pace were 0.72, 0.61, and 0.51 (p trend <0.001). Other studies have confirmed the cardiovascular benefits of walking in men [15, 17]. If physical activity is quantified as distance walked and modeled as a continuous variable, the risk of CHD was shown in the Honolulu Heart Study to be reduced by 15 % for every ½ mile per day increase in walking distance [15].
Manson et al. have extended analyses regarding exercise intensity specifically to women [21, 22]. In the Nurses’ Health Study and Women’s Health Initiative Observational Study, there was a graded decline in new CHD events with increased exercise dose as measured in quintiles of MET-hours/week [21, 22]. Furthermore, increased walking dose and walking intensity (miles/hour) were both inversely related to the risk of CHD, even in the cohort of women who reported no vigorous exercise.
For both men and women, survival advantage is usually evident at relatively low levels of exercise capacity [245]. Blair et al. have shown that a maximal treadmill workrate equivalent to approximately 10 METS in men and 9 METS in women conferred virtually all the exercise-related protection against cardiovascular disease. These studies suggest that a relatively modest increase in physical activity confers a meaningful reduction in death rates from coronary artery disease, and they provide a compelling basis for the current guideline recommendation of 30 min or more of accumulated moderate-intensity exercise on most days of the week for all individuals [4–7]. Of particular note, even a small increase in physical activity, from <500 kcal/week to 500–999 kcal/week was associated with a substantial decrease in total and cardiovascular mortality [25].
Benefits of moderate intensity exercise, including walking, has been confirmed by several other trials [9, 20, 25–27, 231]. For the Harvard alumni, death rates declined steadily as men increased their energy expenditure during recreational activity from 500 to 2,000 kcal per week (equivalent to walking or running approximately 16–18 miles/week). There was no apparent benefit in terms of cardiovascular mortality for those exceeding this level, at least after the first 10 years of follow-up, but the numbers of subjects who sustained such a high level of activity were small [25]. More extended follow-up however suggests that extreme levels of physical activity (>3,500 kcal/week additional exercise training) may further reduce CHD incidence [231].
Elite older athletes have been demonstrated to have youthful cardiac and vascular compliance [162, 170], and extraordinary coronary vasodilatory capacity [142], which is usually used as rationale for high intensity training. However, it has been questioned whether the benefits of exercise plateau at some level of energy expenditure and may even increase health risk when extreme levels are reached [249, 250]. Investigation of runners has shown cardiac repolarization to be altered after a marathon which occurred in the setting of electrolyte disturbances and increased inflammatory markers [251]. Intensive exercise, for long durations and on a constant basis has also been associated with right ventricular dysfunction [252].
Acute Risks of Exercise
Although exercise confers substantial benefits over time, it is also associated with acute risks. The hemodynamic and neuroendocrine responses to an acute bout of exercise raise myocardial oxygen requirements and alter the functional milieu of the myocardium which may increase the risk of arrhythmias and sudden cardiac death [253–257], or myocardial infarction [217, 258]. Fortunately, the exercise-related risks for ostensibly healthy individuals are very small [259, 260]. For joggers in the state of Rhode Island, Thompson has estimated the risk to be one death per 7,620 joggers/year [261]. Examined in another fashion, this risk of sudden death in the Physician’s Health Study after each episode of vigorous exercise was estimated at 1 death for every 1.51 million episodes [253]. Importantly, the risk of dying or triggering a myocardial infarction while exercising is substantially higher for normally sedentary individuals who exercise occasionally, compared to habitual exercisers [217, 258, 261]. Moreover, there is an inverse dose–response relationship between the frequency of regular exercise and the risk of exercise induced cardiovascular complications. Thus the relative risk of triggering a myocardial infarction during or immediately after heavy exercise compared to other times during the day is only 2.4 for individuals who exercise five times or more per week but increases exponentially to 8.6 (three-four times/week), 19.4 (one-two times/week) up to 107 for those very sedentary individuals who engage in heavy physical exertion less than one time/week [217]. If the relative risk of dying during exercise is compared to the long-term health benefits of exercise, the balance of effects still favors regular exercise [256, 257]. Thus while habitual exercisers have a relative risk of dying suddenly at any time, it is less than one-half that of sedentary individuals [254, 256].
There are unique risks of high intensity training (e.g., participating in marathons or triathlons) due to the intensity of the exercise involved, although the risk of sudden cardiac death appears to be exceedingly low [262]. A more recent study of nearly 11 million runners determined that the incidence of cardiac arrest was higher during marathons (1 per 100,000 participants) than shorter races (0.27 per 100,000 participants), but the overall risk was low [263]. Ultimately, aerobic exercise training reduces cardiovascular risk in a dose-dependent manner, but extreme levels of exercise of greater than 1 h daily produces diminishing returns and may cause adverse cardiovascular effects [252, 264].
Initiating an Exercise Program
Prior to initiating regular physical activity, it is generally recommended that sedentary patients should undergo history and physical examination to identify cardiovascular risk factors and any potential contraindications to activity. The American College of Cardiology and American Heart Association recommend screening with exercise treadmill testing for asymptomatic individuals with diabetes mellitus, men over 45 years of age, and women over 55 years of age before undergoing vigorous physical activity [265]. Although it may be reasonable to forgo stress testing in asymptomatic individuals prior to exercise, a more conservative exercise progression may help to maintain optimal safety. Patients can be instructed to advance exercise progressively but to report concerning symptoms or concerns if they occur.
In these instances, moderate intensity exercise is usually initiated with a gradual increase in activity [266]. It is certainly possible that occult CHD, valve disease, and arrhythmias may manifest themselves only once an individual becomes active, i.e., many affected adults are asymptomatic only because they are sedentary. Therefore a cautious approach is to advance exercise intensity incrementally from a starting point that is modest such that symptoms become evident before there are serious risks to the individual.
It is important to emphasize that pharmacologic stress testing usually provides a poor substitute for exercise testing assessments. Exercise stress testing is indicated to assess for exercise-induced symptoms, arrhythmia, hemodynamic parameters, as well as ischemia, and to serve as the basis for clinical recommendations. Exercise testing can usually be modified to provide clinically meaningful risk stratification and diagnosis even for men and women, including those who are elderly, deconditioned, or obese if time and effort is taken to select protocols and modes appropriate for each patient.
Patients should have a goal of obtaining at least 150 min of moderate or 60 min of vigorous exercise weekly [267]. Moderate exercise includes those activities in which the patient can still comfortably converse during the activity, whereas vigorous activity precludes comfortable conversation [267]. Patients who were previously sedentary will require guidance on steadily increasing physical activity over the course of weeks to reach desired levels.
Strength training is often critical as a means to build enough strength to tolerate aerobic activity. For deconditioned and/or frail adults, increasing duration of low-intensity exercise should usually precede steps to increase exercise intensity. Frequent follow-up and encouragement by physicians are important for improving patient adherence and success with an exercise program. Supervision is particularly important for patients beginning a strength training regimen because of the importance of teaching proper breathing (avoiding Valsalva breathing) and to foster techniques that minimize the risks of musculoskeletal injury.
Secondary Prevention of Ischemic Heart Disease
Once an individual has suffered the consequences of coronary artery disease, attention turns to secondary prevention, i.e., minimizing morbidity and mortality, as well as to limiting the progression of disease and increasing function/independence/and QOL despite having the disease. Cardiac rehabilitation is the model for accomplishing these goals.
Cardiac rehabilitation may be broadly defined as the process by which patients with heart disease are assisted in achieving their maximal physical capacity and psychosocial well-being while reducing their future cardiovascular risks, which is typically after a period of acute cardiovascular illness. Patients who are considered eligible for cardiac rehabilitation include any patient who has had one of the following as a primary diagnosis within the previous year: myocardial infarction or acute coronary syndrome, coronary artery bypass graft surgery, percutaneous coronary intervention, stable angina, heart valve surgical repair or replacement, and/or heart or heart/lung transplantation [268]. Many contend that HF and PAD should also be eligible for CR, based on strong evidence-based rationales. While this has been impeded by fiscal concerns, it continues to be a dynamic debate at CMS. Many assert that upfront costs associated with cardiac rehabilitation for HF and or PAD would be counterbalanced by significant savings in relation to other health care costs (e.g., hospitalizations and morbidity) that are prevented by cardiac rehabilitation therapy.
Although commonly conceptualized as an exercise program, contemporary cardiac rehabilitation standards have evolved into an individualized, multidisciplinary, comprehensive approach to cardiovascular risk reduction [269–272]. The core components of a cardiac rehabilitation and secondary cardiovascular prevention program include: nutritional counseling, weight management, blood pressure and lipid management, diabetes management, tobacco cessation and psychosocial management in addition to physical activity counseling and exercise training [272]. In fact, the American Heart Association has stated that exercise only programs are not considered cardiac rehabilitation [270, 271]. Furthermore, in 2007 performance measures were first published on cardiac rehabilitation for secondary prevention highlighting the critical importance of rehabilitation programs to increase the delivery of cardiac rehabilitation to promote overall healthy behaviors and active lifestyles which will perpetuate beyond the early intervention periods [268].
Reduction in Cardiovascular Events
The scientific evidence of the benefits of cardiac rehabilitation for patients with cardiovascular disease has been growing [273]. In contrast to the extensive data from apparently healthy persons, early studies of the effect of cardiac rehabilitation on mortality following a myocardial infarction were rarely of sufficient size to document a prolongation of life from exercise training. When the experiences of major post-infarction trials are combined in a meta-analysis, the evidence suggests that cardiac rehabilitation has a significant impact upon improving outcomes [274–276]. A recent review of 48 trials encompassing 8,940 patients [276] confirmed previous meta-analyses [274, 275] demonstrating a reduction in cardiovascular mortality, including the incidence of sudden death or fatal myocardial infarction, by approximately 25 % over 3 years; a magnitude equivalent to the beneficial effect of beta blockade or statin medications. Cardiac rehabilitation also reduces all-cause mortality [274–276], but no difference was identified however for the incidence of recurrent non-fatal myocardial infarction. It is important to note that this analysis included a greater proportion of women, elderly, and patients who had undergone coronary revascularization than previous meta-analyses further expanding the evidence base for a variety of patients. Suaya et al. evaluated Medicare beneficiaries using propensity analysis to match for common confounders (e.g., extent of CHD, HF, comorbidity, socioeconomics, and advanced patient age) and consistently showed a significant reduction in mortality of 21–34 % associated with cardiac rehabilitation [277]. A retrospective analysis of 2,395 consecutive patients who underwent percutaneous coronary intervention between 1994 and 2008 in Olmsted County, Minnesota demonstrated a significant reduction in all-cause mortality and trend towards decreased cardiac mortality [278, 279].
Further investigation has shown a strong association between the number of sessions of cardiac rehabilitation completed and clinical outcomes. In the previous study there was 19 % relative reduction in 5-year mortality for subjects who completed >24 sessions than those who ≤24 session [277]. In another study of Medicare beneficiaries who attended 36 sessions, their risk of death and myocardial infarction was 14 and 12 % lower than those who attended 24 sessions and 22 and 23 % lower than those who attended 12 sessions, respectively [280]. This dose response to cardiac rehabilitation supports the importance of not only referral of patients to a program, but to long-term adherence.
The American Association of Cardiovascular and Pulmonary Rehabilitation published a position statement highlighting the importance of identifying depressed patients at cardiac rehabilitation programs because depression is highly prevalent in patients who have suffered a myocardial infarction [281]. Presence of psychological disease is a strong risk factor for ischemic heart disease and depressed patients with ischemic heart disease have a threefold higher mortality than non-depressed patients [282, 283]. While depression is associated with poor adherence to cardiac rehabilitation [284], it has also been demonstrated that CR provides important benefits particularly among patients who are depressed [283].
Phases of Cardiac Rehabilitation
Cardiac rehabilitation has traditionally been structured into various phases although to some extent these concepts have been changed by the accelerated hospitalization of most contemporary cardiac patients [285]. Phase I or inpatient cardiac rehabilitation was particularly useful during a time when cardiac patients were usually in the hospital for prolonged lengths of stay. Phase I would begin as soon as the patient stabilized after an acute cardiac event (Table 28.1). Prolonged bed rest and inactivity result in a marked reduction in maximal aerobic performance along with increased risk of blood clots, depression, and deconditioning [286]. So-called “bed rest deconditioning” was a common cause of morbidity in the acute period after a myocardial infarction or surgery. Early, safe mobilization and exercise was the primary goal of phase I cardiac rehabilitation. Other goals include early risk factor and exercise education, promoting independence, conveying medical instructions and arranging transition into phase II (outpatient) cardiac rehabilitation. To a large extent, the need for Phase I cardiac rehabilitation has diminished due to improved interventional and medical management which facilitate early mobilization and discharge. Moreover, physical therapy and nursing often supplant Phase I cardiac rehabilitation even for patients who are relatively more deconditioned and slow to recover. The diminished use of Phase I cardiac rehabilitation has had the ironic effect of undermining what was originally a seamless transition from primary to phase I to phase II cardiac rehabilitation. A recent AHA scientific statement emphasized the importance of a coordinated effort by hospital providers to emphasize phase II cardiac rehabilitation even for patients who may no longer receive phase I [287].
Table 28.1
Goals of phase I cardiac rehabilitation
1. To prevent deconditioning from prolonged bed rest |
2. To facilitate rapid recovery of functional capacity |
3. To shorten duration of CCU care and to enable early hospital discharge safely in appropriate patients |
4. To minimize disability associated with an acute cardiac event and encourage rapid return to an active lifestyle |
5. To improve communication between health care providers and patients, and thus smooth the transition into Phase II Cardiac Rehabilitation and outpatient care |
Phase II or early outpatient cardiac rehabilitation is the short term (36 sessions over 12 weeks), initial outpatient phase of rehabilitation. An expeditious and orderly transition between phase I and phase II is critical to the success of the rehabilitation process and ensures that patients are properly prepared to respond to the education and training programs. Automatic referral for appropriate patients before discharge can increase participation rates in cardiac rehabilitation [288, 289]. During the acute phase of hospitalization, patients are just beginning to cope with the ramifications of their disease and often have substantial difficulty processing new information [290]. It is during the first few weeks of their outpatient care that they are most open to making significant life style adjustments, and risk factor modification is most likely to be successful. This time period is also a critical one from a medical perspective. The period of greatest risk for sudden death after a myocardial infarction is the first 3 months following hospitalization [291], and rehabilitation programs that enroll patients early after a myocardial infarction demonstrate a significant reduction in the rate of sudden death during this high risk time [196]. Major goals of this phase include aggressive interventions to modify cardiovascular risk factors, many of which involve adherence to life-style changes including tobacco cessation and weight loss counseling (Table 28.2). Thus this transition period from inpatient to outpatient represents a physiologic and psychologic “window of opportunity” for maximizing the benefit of the rehabilitation process. It is also important to emphasize that cardiac rehabilitation and successful changes in lifestyle may be achieved by patients regardless of ethnic group, socioeconomic status, or age [292].
Table 28.2
Core components of phase II cardiac rehabilitation programs
1. Patient assessment (history, examination, testing) |
2. Nutritional counseling |
3. Weight management |
4. Blood pressure management |
5. Lipid management |
6. Diabetes management |
7. Tobacco cessation counseling |
8. Psychosocial management |
9. Physical activity counseling |
10. Exercise training |
In addition to education, a major emphasis in Phase II cardiac rehab is on exercise conditioning. Both static and dynamic exercise training programs are utilized to increase strength and endurance. When starting the program, an exercise prescription is usually established based principally on a symptom limited exercise test. The target or training heart rate is usually set at 75–85 % of the measured maximal heart rate or 40–60 % of the heart rate reserve [(maximal heart rate – resting heart rate) × (40–60 %) + resting heart rate], based on the predictable relationship between oxygen uptake and heart rate [44]. Heart rate is thus used as an easily measured estimate of relative exercise intensity. However in some patients, such as those with chronotropic incompetence and/ or who are taking beta blockers or other medications that may affect the heart rate responses, heart rate may not accurately reflect exercise intensity. In such cases, the RPE or Rating of Perceived Exertion (Borg scale) may be helpful. This measure rates the perceived effort on a scale from 6 to 20 with 6–7 being “very, very light”, 19–20 being “very, very hard”, and 12–13 (the optimal training level) being “somewhat hard” [293]. This strategy quantifies effort level independently of heart rate level, and is scaled based on a physiological heart rate range in young healthy subjects from 60 at rest to 200 during maximal exercise [293]. This rating system is remarkably consistent within individuals [294], and thus is independent of pharmacologic therapy that may attenuate the normal heart rate response to exercise. Once patients are trained in its use, they can effectively monitor their own exercise intensity at home without the need for sophisticated heart rate monitoring systems. Cardiopulmonary exercise testing is also a valid alternative to determine appropriate exercise targets based on oxygen consumption (VO2) at the ventilatory threshold. Cardiopulmonary testing also provides additional diagnostic information in patients with excessive dyspnea [295]. A precise work rate that includes VO2 and heart rate range can be identified.
Phase III cardiac rehabilitation is a long-term maintenance program that is designed to continue throughout a patient’s lifetime [285]. The goals of this phase include maintaining physical activity and ongoing risk factor modification with progressive patient self-direction that does not usually require medical supervision. Many patients are initially uncomfortable exercising unsupervised at a gym or at home due to intimidation, fear of the risks, or uncertainty about proper activity and techniques. Patients often prefer a medically sophisticated staff who have capabilities to care for patients in case an adverse event occurs. Cardiac rehabilitation should build patient confidence in their physical ability and body image to overcome these concerns if present. These issues are particularly import for patients who are obese, frail, have obstructive lung disease, and the elderly. Ultimately, patients should gain comfort and acceptance of lifelong adherence to these lifestyle changes. It has been demonstrated in carefully selected, relatively well educated patients, that home-based exercise training and cardiac rehabilitation can be performed safely and with the anticipated training effect [296]. Furthermore, a significant reduction in the incidence of cardiovascular events has been among male patients who attended phase III cardiac rehab for 6 months [297].
Issues in Clinical Practice
Despite the well-established benefits of reducing morbidity and mortality in patients with ischemic heart disease with cardiac rehabilitation, these programs severely underutilized with a minority of eligible patients participating [298]. This poor utilization is due to both low referrals by providers and poor participation – both enrollment and adherence – by patients. Major factors that contribute to this underuse of programs by patients are the lack of accessibility to program sites, inadequate insurance coverage, and different priorities. Providers refer patients infrequently to programs, especially women, older adults, and ethnic minorities, although these groups are also less likely to participate in programs even if referred [273, 299]. Poor adherence in cardiac rehabilitation programs has been associated with being older, female gender, less education, lack of understanding of the benefits, and less baseline physical activity [300]. In contrast, the strongest predictor of both referral to a program and patient participation is physician endorsement emphasizing the importance of supportive and engaged providers in the process [301, 302].
Decisions on the optimal training load are complicated in patients with provocable ischemia, for whom the desired training intensity may fall above the ischemic threshold. There currently is no consensus on how to train patients who have clear provocable ischemia at a low workload and thus are limited by myocardial rather than systemic oxygen transport. Some centers train patients at 75–85 % of directly measured maximal heart rate despite the presence of ischemia in an attempt to maximize the training effect. However a meaningful training effect can be obtained in patients with or without provocable ischemia using more moderate exercise, at an intensity equivalent to 60–75 % of maximal heart rate [303]. In addition, data in animals suggest that ischemic exercise results in profound and prolonged depression of ventricular contractile function that persists for hours after an exercise bout [304]. Despite the potential benefits of ischemic preconditioning in patients with ischemic heart disease [305], training above the ischemic threshold is likely to increase the risk of exercise [306, 307]. Training in these patients thus should take place at a heart rate no higher than 10 beats/min below the value at which ischemia develops (or below the ventilatory anaerobic threshold) [44].
Previous case reports suggested that some caution was warranted for exercise in patients who have recently undergone percutaneous revascularization, with or without stent implantation [308–310]. However, a case series of 261 patients at one institution who had coronary stenting and subsequent exercise treadmill testing within 60 days revealed no cases of exercise induced stent thrombosis [311]. In addition, a recent randomized study of 1,000 subjects assessed the safety of symptom-limited exercise treadmill testing 1 day after coronary stent placement. After 14 days, the incidence of acute stent thrombosis in those undergoing exercise testing was identical to the usual care group (1 % each group; p = 1.0) [312]. However, the risks associated with regular vigorous exertion or with unsupervised exercise soon after PCI are unknown, and it may be prudent to wait at least 5–7 days before initiating an exercise program [44]. Similarly, the optimal timing of exercise after coronary artery bypass grafting surgery is not known but due to additional concerns such as deconditioning and wound healing, it may be appropriate to wait at least 2–4 weeks before initiating an exercise program and possibly longer before weight-bearing exercises are introduced into the patient’s regimen.
There is little doubt that vigorous exercise itself is an important risk factor for sudden death, particularly in unfit individuals [253, 257, 261, 306, 313, 314]. However these risks are mitigated if exercise is advanced progressively in a chronic regimen to allow for physiologic adaptations to occur that have been highlighted such as improved risk factors, improved coronary and peripheral vascular dynamics, and autonomic tone [256, 315]. The risk of exercise induced cardiac arrest is also directly related to the intensity of the exercise [307]. Lower intensity training might therefore theoretically be safer than vigorous exercise, particularly in the unsupervised cardiac patient. In fact, for both men and women, low – moderate intensity exercise training at home, at heart rates from 60 to 75 % maximum, can result in substantial improvements in functional capacity, with an excellent degree of safety and compliance [303, 316]. In addition, as described above, even relatively small increases in fitness, equivalent to that obtained in a low – moderate intensity training program (emphasizing walking or slow jogging), can result in significant decreases in cardiovascular mortality [245]. High intensity interval exercise training has been described for specific patient populations, including older and or higher risk patients [317]. Training benefits are increased due to the higher intensity of training, and risks are modified due to integrated periods of lower intensity that promote equilibration and stabilization. Close supervision is particularly critical for this type of training and also allows for ongoing education regarding lipid reduction, medication compliance, smoking cessation counseling, and a healthy diet which are the components of an effective cardiac rehabilitation program.
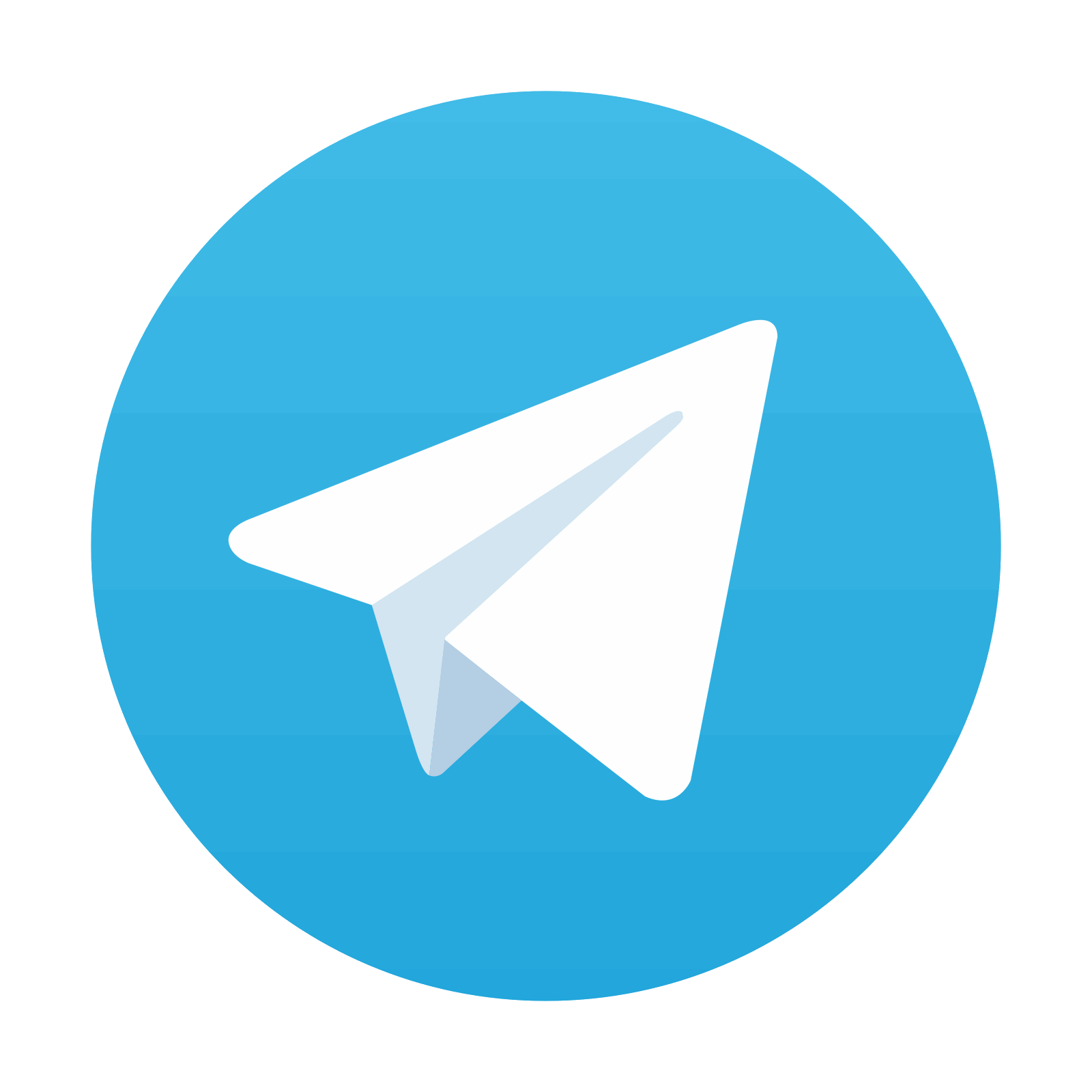
Stay updated, free articles. Join our Telegram channel

Full access? Get Clinical Tree
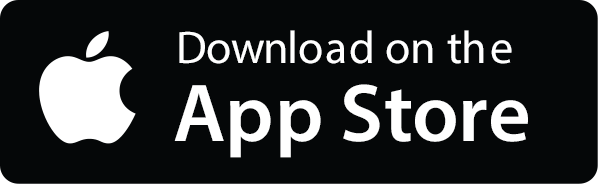
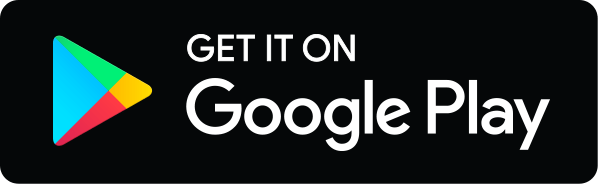