Lower CROP index values are predictive of extubation failure; especially with a CROP value of ≥ 0.15 ml/kg/breaths/min (Baumeister et al. 1997; Thiagarajan et al. 1999; Newth et al. 2009; Emeriaud and Newth 2015).
Volumetric capnography draws the diagram of capnography considering concentration of CO2 in airway gas versus volume of expiration. The slop of the diagram helps us calculate physiologic dead space which is the ratio of “dead space/tidal volume” or “VD/VT.” If the VD/VT ratio is less than 0.05, extubation could be predicted successful; however, if the VD/VT ratio is more than 0.65, then extubation is predicted to be failed.
“Ready for Departure”: Preparing for Extubation
Extubation criteria include a number of general clinical conditions and some measurable tests; clinical criteria for extubation are briefed in Table 38.1.
Table 38.1
Clinical criteria for extubation
CNS and muscular system | Wakefulness: the patient should be sufficiently awake |
The tone of muscles should be working completely in such a way to grasp firmly the objects like fingers of examiner or produce strong and forceful cough | |
Airway | Patent upper airway is mandatory which is tested through performing air leak test around the endotracheal tube |
Airway reflexes intact in such a way that could protect the lungs from aspiration | |
Cardiovascular system | Stable cardiovascular and hemodynamic parameters |
Minimal dose of inotropes | |
Respiratory system | Minimal respiratory secretions which will not create a clinical problem Normal range for breath sounds |
Ability to produce strong and forceful cough | |
SpO2 > 94 with minimal supplemental O2 requirement (i.e., <30 %) Minimal need for pressure support (5–10 more than PEEP) | |
Water and electrolytes | No significant blood gas and/or electrolyte abnormalities |
Controlled water and loading status |
Common tests used for assessment of extubation readiness include:
Respiratory muscle strength demonstrated by negative inspiratory force (NIF)
ETT “leak test” which assesses the leakage of air around the endotracheal tube and checks for upper airway patency; however, Wratney et al. demonstrated that an air leak pressure ≥ 30 cm H2O in the non-paralyzed patient (either in the time before extubation or during the time of mechanical ventilation) cannot predict an increased risk for extubation failure (Wratney et al. 2008)
The “Extubation Procedure”
The following steps are basic steps in the extubation procedure:
Ensuring adequacy of clinical criteria for extubation (Table 38.1).
Sterile suctioning of the oropharynx, nostrils, and endotracheal tube.
Emptying gastric contents through suctioning nasogastric tube.
Having nasal prongs on the face before extubation and preparing oxygen facemask, in order to decrease the chance of immediate hypoxia.
Checking equipment for assisting ventilation devices including mask and bag attached to oxygen; devices for NIPPV or CPAP should be available.
Checking for laryngoscope and different blades and blade sizes, different size tubes, and other ventilation devices to be available.
Ventilator and related tubing should be ready.
Checking for sedatives and muscle relaxants, adjusted by weight and including rapid sequence induction agents per needed.
Checking for nebulizers including epinephrine and other agents to be available.
Selected corticosteroids should be available as bolus and infusion doses to decrease any hoarseness or respiratory distress; also, diuretics should be available to treat volume overload and respiratory distress; of course, there is no proof that corticosteroids could prevent or treat post-extubation stridor in neonates or children (Khemani et al. 2009).
Respiratory monitoring including arterial blood gases should be available.
Describing the procedure for parents.
Predictors of Extubation Failure
Extubation failure (EF) is defined as reintubation within 24–72 h; based on pediatric studies, a failed extubation rate less than 10 % is accepted as the norm (Kurachek et al. 2003; Rothaar and Epstein 2003; Newth et al. 2009; Artime and Hagberg 2014; Saikia et al. 2015). Whatever the rate of EF, the trend of pediatric EF has significant differences with adults, including:
The majority of pediatric patients weaned from mechanical ventilation in 2 days or less.
RSBI, CROP, and volumetric capnography, though considered as criteria for prediction of extubation success, could be used as measures of prediction for EF.
Protocols used in adult weaning with significant effect on extubation trend do not have a significant effect on time for weaning in pediatrics and neonates; in fact, there is paucity of evidence in supporting or rejecting the effects of “protocolled” over “non-protocolled” weaning regarding duration of mechanical ventilation.
EF rate does not have significant difference between pressure support ventilation weaning, volume support ventilation weaning, and other methods.
Obstruction of the upper airway is claimed as the most common etiologic factor for EF (Newth et al. 2009).
The incidence of EF is higher in these patients:
- 1.
Neonates and infants.
- 2.
Patients with underlying cyanotic congenital heart disease.
- 3.
Patients under prolonged mechanical ventilation.
- 4.
Patients with increased RSBI, inadequate cough reflex, and thick unmanageable secretions could increase the chance for EF and could be used as predictive measures (Thiagarajan et al. 1999; Randolph et al. 2002; Rothaar and Epstein 2003; Newth et al. 2009; Khemani and Newth 2010; Artime and Hagberg 2014; Gupta et al. 2014; Saikia et al. 2015; Wielenga et al. 2016).
Noninvasive Ventilation
RAM Cannula
Nasal cannulas, nasal prongs, or often called RAM cannulas can deliver supplementary oxygen to the patient in relatively low amounts. They are mainly used when we decide to administer oxygen but the desired increase in FiO2 is not so much high. FiO2 increase by nasal prongs depends mainly on these factors:
Oxygen flow
The pattern of respiration of the patient
The following table demonstrates estimates of FiO2 when applying nasal prongs:
Oxygen flow (liters per minute) | Highest achievable FiO2 (%) |
---|---|
1 | 24 |
2 | 27 |
3 | 30 |
4 (needs humidification) | 33 |
5 (needs humidification) | 35 |
6 (needs humidification) | 38 |
nCPAP
Neonatal continuous positive airway pressure (nCPAP) is the special CPAP used for neonates aiming to use a noninvasive ventilation mode with the following goals:
Improving oxygenation status
Increasing functional residual capacity (FRC)
Decreasing intrapulmonary shunts
Decreasing the degree of alveolar collapse
Decreasing ventilator-induced lung injury
Decreasing the incidence of lung complications like VAP
Decreasing the chance for reintubation
Reducing the rate of readmission to critical care unit
Decreasing mortality
More than 40 years has passed from invention of nCPAP; however, during the last years, great improvements have been made. According to Diblasi, the two most influential factors affecting outcome in neonates treated with nCPAP are “clinicians’ abilities to perceive changes” which occur in pathophysiology of the infants who are under treatment with nCPAP and “quality of airway management”; also, other studies have demonstrated that careful selection of appropriate patient associated with the level of training and experience in ICU team affects the outcome. Although some believe that nCPAP and other noninvasive methods are good enough to prevent reintubation in neonates and infants after cardiac surgery, there is a significant difference between alternative modes of nCPAP and other noninvasive modes of ventilation; also, in neonates, risk of nasal injury and sternal dehiscence is real (Kurt et al. 2008; Diblasi 2009; Squires and Hyndman 2009; Zarbock et al. 2009; Boeken et al. 2010; Pelosi and Jaber 2010; Drevhammar et al. 2012; Bancalari and Claure 2013).
One of the Cochrane systematic reviews published in 2014 has compared noninvasive positive pressure ventilation (NIPPV) with nCPAP for preterm neonates after extubation (Lemyre et al. 2014) and has stated that:
Clinical signs of extubation failure are significantly less common after using NIPPV compared with nCPAP.
Reintubation within 2–7 days after extubation is less with NIPPV.
These differences have no effect on chronic lung disease or mortality rate.
Synchronization could have an important role in NIPPV.
High-Flow Nasal Cannula (HFNC)
HFNC has been extensively used in pediatric ICUs during the last 10 years, at times replacing nCPAP, due to its simple basics of function and excellent tolerance by the patients, especially because HFNC delivers humidified and warm air which is much better tolerated than cold air. HFNC delivers high flow rate of heated (34°C and 37°C) and humidified gas, often as much as 2 L/kg/min of blended air and oxygen which is heated and humidified. Some clinicians believe that it can improve the respiratory function in the following items (Manley et al. 2013; Yoder et al. 2013; Milesi et al. 2014; Wilkinson et al. 2016):
Washout of dead space in the nasopharynx
Improved gas exchange
Clearance of the pulmonary mucociliary tract
Lung oxygen delivery
Humidification of delivered gases to the lungs
Improved breathing pattern and decreased work of ventilation and breathing
Post-extubation ventilatory support
There are some controversies about the efficacy of HFNCs. For example, two different Cochrane Database of Systematic Reviews published in 2014 have somewhat questioned (with different degrees of uncertainty) the efficacy of HFNC for infants with bronchiolitis and for children (Beggs et al. 2014; Mayfield et al. 2014). On the other hand, a Cochrane Database of Systematic Reviews published in 2016 concluded that using HFNC could be useful as post-extubation support, with decreased chance of nasal trauma and pneumothorax in comparison with nasal CPAP (Wilkinson et al. 2016).
Miscellaneous Issues
Extracorporeal CO2 Removal: ECCO2R
ECCO2R implies partial respiratory support which can help remove carbon dioxide out of the blood using low levels of blood flow through an extracorporeal circuit with minimal effects on blood oxygenation. In patients under mechanical ventilation, there is always a chance for ventilator-induced lung injury (VILI); there are some ventilation modalities to counteract VILI; among them, ultra-protective ventilation strategies could be named which results in normal peak inspiratory pressure, very low tidal volumes, near normal lung oxygenation, and lower values for minute ventilation; this leads to nearly appropriate oxygenation, while hypercarbia remains a problem. ECCO2R is often used as part of ultra-protective ventilation strategies in order to remove CO2 out of the body and compensate for CO2 accumulation in ultra-protective ventilation strategies. In ECCO2R, the amount of blood being perfused is very much lower than the amount of perfusion provided by ECMO. Also, ECCO2R is not a new modality; however, during the last years, its application in association with ultra-protective ventilation strategies has gained much more popularity (Habashi et al. 1995a; Habashi et al. 1995b; Kaushik et al. 2012; Camporota and Barrett 2016; Deniau et al. 2016).
HFV or HFOV
High-frequency ventilation or high-frequency oscillatory ventilation seems a superb protective measure in perioperative care of congenital heart disease children with injured lungs using very small and tiny tidal volumes and high rate of ventilation in order to keep airway pressure at the modest possible level. Though institution of HFV is delayed, there is a shift toward the early use of HFV during the last years. However, there is still paucity of evidence to demonstrate clearly the improved outcomes of early institution of HFV in respect to lung protection (Fessler et al. 2008; Khemani and Newth 2010).
Effect of HFV on outcome
Based on the systematic review and meta-analysis performed by Duyndam et al., the available evidence scarcely supports that HFV can decrease mortality and length of stay in critically ill children beyond the newborn period (Duyndam et al. 2011). In addition, Henderson–Smart et al. conducted a Cochrane Database of Systematic Reviews and showed that no controlled trial supports the use of HFOV in term or near-term infants with severe pulmonary dysfunction (Henderson-Smart et al. 2009).
Inhalational Routes of Drug Delivery
The list of drugs administered through inhalational route is an increasing list, especially with the application of newly designed targeted therapies, including nanostructured materials and nanostructured carriers (Dabbagh and Rajaei 2011). This is a list of drugs being used as inhalational therapies:
Inhalational drugs used in treatment of pulmonary hypertension are very good and common examples; agents like iNO and treprostinil are used for treatment of pulmonary hypertension; they are fully discussed in Chap. 29– Pulmonary Hypertension.
Inhaled insulin which is recently approved by FDA.
Many antibiotics like inhalational ciprofloxacin encapsulated in liposomes or antituberculosis drugs which causes “massive reduction” of drug dose and decreased toxicity (Wood 1991; O’Callaghan 1994; Rastogi et al. 2006; Gupta and Ahsan 2010; Dabbagh and Rajaei 2011; Petkar et al. 2011; Stream and Bull 2012; Hamblin et al. 2014; Brashier et al. 2015).
Chest Physiotherapy
In patients undergoing congenital cardiac surgeries, using standard protocols for physiotherapy might improve pulmonary status; physiotherapy, especially aerobic exercise, could be useful in improving exercise capacity and also postoperative pulmonary function and cardiopulmonary fitness; however, this is a matter of controversy. In a systematic review, Pasquina et al. demonstrated that there is no proof for usefulness of prophylactic respiratory physiotherapy in prevention of pulmonary complications after cardiac surgery (Pasquina et al. 2003; Nagarajan et al. 2011; Kaminski et al. 2013; Duppen et al. 2015).
Chronic Respiratory Failure and Tracheostomy
Many years have passed since the first introduction of tracheostomy; even, many believe that it has been used in Medieval Islamic physicians, Avicenna to be named (Golzari et al. 2013; Dabbagh et al. 2014). The recently published analysis of the Society of Thoracic Surgeons Congenital Heart Surgery Database demonstrated an increasing rate of tracheostomy due to congenital heart surgery. The main indications were divided as preoperative indications (mainly prematurity, genetic anomalies, and preoperative mechanical ventilation) vs. postoperative ones (cardiac arrest, modes of extracorporeal support, injuries to the phrenic or laryngeal nerves, and neurologic insults); also, based on this report, the operative mortality was 25 % (Donnelly et al. 1996; Al-Samri et al. 2010; Mastropietro et al. 2016).
On the other hand, when speaking about different aspects of tracheostomy in congenital heart surgeries, the following items are still among the most controversial issues for tracheostomy, including pediatric surgery ICUs:
Timing (i.e., early versus delayed): the only justified indication for early tracheostomy is patient comfort, i.e., when the patient suffers from pain due to endotracheal tube or other translaryngeal devices and tracheostomy is the preferred method for patient safety (Donnelly et al. 1996; Kremer et al. 2002; Al-Samri et al. 2010).
Indications: prolonged ventilation is the most common indication in pediatric tracheostomy, though it has many controversial issues due to its risk/benefit assessments; this is followed by other less common indications like using aerosol treatments, nosocomial pneumonia, witnessed aspiration event needing emergent tracheostomy, and repeated reintubations (Donnelly et al. 1996; Kremer et al. 2002; Al-Samri et al. 2010).
Techniques: unless there is a life-threatening emergency, pediatric tracheostomy is a procedure that should be done in the operating room while the patient is intubated; it is recommended to use a horizontal incision on the skin and then vertical tracheal incision; any tracheal resection is prohibited (Donnelly et al. 1996; Kremer et al. 2002; Fraga et al. 2009).
Risks/benefits: benefits of tracheostomy include patient comfort, mobility and speech, oral ingestion, improved suctioning of the airway, decreased airway resistance and more secure airway, improved weaning process from mechanical ventilation, and decreased rate of VAP; none of them are yet proved to be correct; the main etiologies for tracheostomy-related death include cannula obstruction and accidental decannulation; however, the most frequent early complications are pneumomediastinum, pneumothorax, wound-related complications, and tracheostomy site bleedings; common late complications include tracheal stenosis and formation of granulation tissue (Donnelly et al. 1996; Kremer et al. 2002; Al-Samri et al. 2010; Mastropietro et al. 2016).
References
Ahmadpour-Kacho M, Zahedpasha Y, Hadipoor A, Akbarian-Rad Z. Early surgical intervention for diaphragmatic paralysis in a neonate; report of a case and literature review. Iranian J Pediatr. 2011;21:116–20.
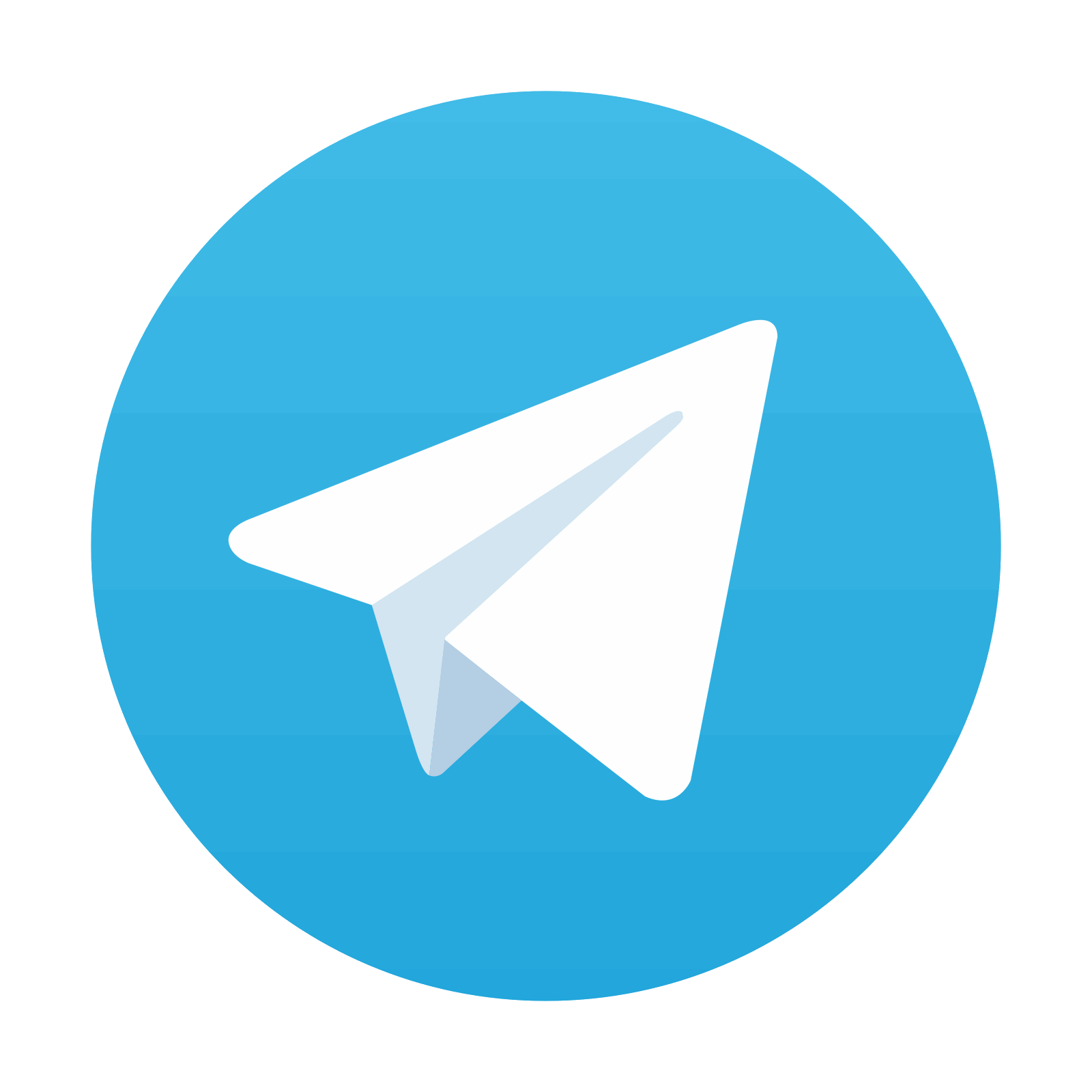
Stay updated, free articles. Join our Telegram channel

Full access? Get Clinical Tree
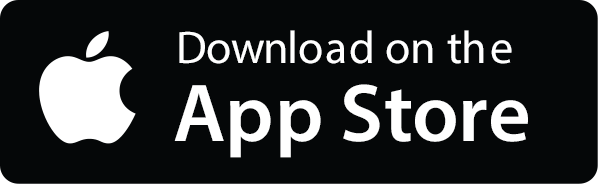
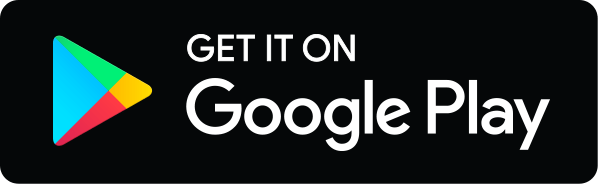