Risk factor
Relationship with CNS disorders
Genetic risk factors
Genetic syndromes have a very worse effect on CNS outcome; among them, these are the main ones: trisomy 21, DiGeorge syndrome, conotruncal anomalies, VACTERL, and velocardiofacial syndrome (Atallah et al. 2007; Gaynor et al. 2007; Lee et al. 2008; Kaltman et al. 2010; Chung et al. 2015)
Most of the above genetic syndromes have a common syndrome: 22q11 chromosome deletion (Goldmuntz 2005)
In neonates with 22q11.2 deletion syndrome, the chance for congenital heart disease is about 80 % (Momma 2010)
Carotti et al. have described a number of conotruncal anomalies associated with 22q11 deletion syndrome: “tetralogy of Fallot, pulmonary atresia with ventricular septal defect, truncus arteriosus, interrupted aortic arch, isolated anomalies of the aortic arch, and ventricular septal defect” which are usually associated with other “anomalies of the aortic arch, pulmonary arteries, infundibular septum, and semilunar valves” (Carotti et al. 2008)
Other genetic syndromes that are associated with developmental delay in children with congenital heart disease are Alagille, CHARGE, Down, Jacobsen, Noonan, Turner, and Williams (Marino et al. 2012)
Impaired development of CNS in utero
Antenatal administration of the following could help prevent in utero CNS injuries especially for protection of oligodendrocytes:
Free radical scavengers including vitamin E
Anti-inflammatory and/or anti-cytokine agents to decrease chance of fetal/maternal inflammation
Antibiotics to decrease chance of infection
Congenital structural CNS malformations and/or acquired CNS disorders or injuries in utero or during the perioperative period
There is increased chance of congenital structural CNS disorders in patients with CHD; cerebral dysgenesis has a wide spectrum from microdysgenesis to major anatomical congenital defects like corpus callosum agenesis, white matter injury, microcephaly, and incomplete opercularization; also, “hypotonia, seizures, feeding difficulties, and brain imaging abnormalities like stroke, hemorrhage, or periventricular leukomalacia” are among the lesions in these patients (Newburger and Bellinger 2006; Licht et al. 2009; Rollins and Newburger 2014)
Antenatal hemodynamic instability and/or preoperative hemodynamic instability including preoperative hypoxia or severe acidosis
Before birth, impaired fetal blood flow and the resulting decrease in brain oxygen delivery are the main sources of brain insults (Licht et al. 2009; Kaltman et al. 2010)
After birth, decreased or impaired oxygen delivery to brain results in injuries to the CNS especially the white matter or those regions communicating different parts of the brain (Kaltman et al. 2010)
Preoperative hyperthermia
Even mild degrees of hyperthermia in ischemic CNS regions could have considerable and significant impacts on CNS status; these deleterious effects should especially be avoided in patients undergoing DHCA; CNS tissue is highly sensitive to ischemia, and temperature changes especially during pre-bypass period and early post-bypass (Shum-Tim et al. 1998; Nussmeier 2005; Shamsuddin et al. 2015; Hu et al. 2016)
Preoperative hypoglycemia
Tight control of blood glucose is controversial; however, hypoglycemia should be avoided (Hirsch et al. 2012)
Prematurity
Congenital heart disease patients with prematurity (<37 weeks, especially those weighing less than 1500 g at birth) are at high risk for developmental delays and/or disorders (Marino et al. 2012; Rollins and Newburger 2014); currently, despite great improvements in care of the neonates in NICUs, about 5–10 % of premature neonates have major motor deficits, while more than 50 % have significant impairments in behavioral, cognitive, or sensory fields (Khalil et al. 2014; Back 2015; Li et al. 2015)
Low birth weight
Perinatal asphyxia and/or cardiorespiratory problems in perinatal period
Trauma during birth
Age at the time of surgery
Early corrective surgery vs. late correction is still controversial; early correction is in favor of improved cardiac; also, CNS plasticity in neonates could possibly compensate for the effects of CPB; on the other hand, later correction might be in favor of improved CNS outcome and preventing untoward effects of CPB on CNS; controversy still exists (Kaltman et al. 2010; Barron 2013)
Preoperative treatments
Underlying cardiac lesion
Mechanical support/heart transplantation
Patients receiving mechanical support both in preoperative period or during postoperative care (either as extracorporeal membrane oxygenation or ventricular assist devices) are at risk of hemodynamic instability; besides, they are at increased risk of thromboembolic events; on the other hand, patients with heart transplantation are at increased risk for worse CNS outcome (Massaro et al. 2008; Marino et al. 2012; Rollins and Newburger 2014)
Intraoperative Risk Factors for CNS Injuries in Neonates and Children
The main intraoperative risk factors could be mainly categorized under cardiopulmonary bypass (CPB)-related factors which are summarized in Table 37.2; however, intraoperative risk factors are not just limited to this table (Shen et al. 2003; Hsia and Gruber 2006; Lee et al. 2008; Massaro et al. 2008; Albers et al. 2010; Kaltman et al. 2010; Dabbagh et al. 2012).
Table 37.2
Intraoperative and cardiopulmonary bypass (CPB)-related factors affecting CNS outcome in neonatal and pediatric congenital heart surgery
Potential risks | How to prevent or compensate |
---|---|
Gaseous microemboli | Using arterial filters (at least 40 μ; smaller 20 μ filters are superior); using microporous hollow fiber oxygenators; debubbling the oxygenator before CPB with extreme accuracy and vigilance |
Macroemboli and particles (especially clots); low levels of antithrombin III | Ensuring enough dose of heparin for anticoagulation before establishment of CPB; using fresh frozen plasma (FFP) in prime volume to compensate for lower levels of antithrombin III, which is common especially in neonates and infants less than 6 months of age; possibly, antithrombin III supplementation is much more efficacious than FFP (Codispoti et al. 2001; Kaltman et al. 2010) |
Inflammation due to CPB circuit | A number of anti-inflammatory strategies have been suggested in many studies, in order to be used during CPB; these include steroids (especially methylprednisolone), heparin-coated circuits, leukocyte filtration strategies, using ischemic preconditioning models for the brain, institution of modified ultrafiltration, etc.; none have been proved as a definite therapy (Shen et al. 2003; Ungerleider and Shen 2003; Lee et al. 2008; Kaltman et al. 2010) |
Potential episodes of systemic hypotension or hypoperfusion during CPB | Appropriate monitoring; administering more flow during CPB than “full-flow bypass” (Shamsuddin et al. 2015) |
Hemodilution during CPB | Avoiding extreme hemodilution during CPB with different methods like priming part of the circuit with RBCs, managing cardioplegia, and using minimum volume circuits; it has been recommended to adjust hematocrit levels with CPB temperature (in Celsius); however, strong evidence recommends strict avoidance of hematocrit levels below 24 % (Ungerleider and Shen 2003; Durandy 2010; Kaltman et al. 2010; Hirsch et al. 2012) |
DHCA (deep hypothermic circulatory arrest) | DHCA is a real risk especially when prolonged more than 45 min; administering antegrade cerebral perfusion or regional low flow cerebral perfusion (Gaynor et al. 2007; Kaltman et al. 2010) Alternative neuroprotective strategies like antegrade cerebral perfusion (ACP) have been used for CNS preservation; however, regional cerebral perfusion (RCP) has not been much in favor of improved outcomes (Fraser and Andropoulos 2008; Nelson et al. 2008; Ohye et al. 2009; Kaltman et al. 2010) At least 20 min of cooling should be allowed in order to have appropriate cerebral cooling before DHCA (Hsia and Gruber 2006) |
Rate and duration of core cooling | |
pH management during core cooling | |
Air trapping and air emboli after cardiac chambers are opened | Preventing air ejection into the arterial system after removal of aortic cross clamp by the surgeon; if air bubbling occurs, reestablishment of CPB and other measures like hyperbaric oxygen therapy could be useful |
Impaired cerebral perfusion; hemodynamic perturbations of cerebral blood flow (CBF) | Ensuring adequate cerebral perfusion pressure (CPP) and cerebral blood flow (CBF) during CPB; preventing hypotension during CPB; avoiding hemoglobin drop; checking CPB circuit for proper and appropriate size of arterial and venous cannulae; sufficient setting of the CPB reservoir and venous cannulae to ensure venous drainage (Dehaes et al. 2015) |
Oxidative stress of the CNS | Antioxidative measurements; normoxic management and avoidance of hyperoxia and controlled reoxygenation during CPB especially in cyanotic patients; monitoring with F(2)-isoprostanes (F(2)-IsoPs), neuron-specific enolase (NSE), S-100 beta, C-reactive protein (Arneson and Roberts 2007; Kaltman et al. 2010; Morita 2012; Cardenas-Rodriguez et al. 2013; Kochanek et al. 2013; Caputo et al. 2014) |
Anesthetic drugs | There is great amount of concern in favor of anesthetic neurotoxicity in animal models of developing brain; however, clinically there is still controversy without decisive results, and those studies performed in human have failed to confirm these studies in human pediatric and neonatal anesthesia (McCann and Soriano 2012; Vutskits 2012; Zhou and Ma 2014; Hansen 2015) |
Postoperative Risk Factors for CNS Injuries in Neonates and Children
During the postoperative period, a number of events go on. First of all, the aftermath of surgical trauma, with all its inflammatory events, affects different organs including CNS. Second, factors like “impaired autoregulation, unstable hemodynamics, hyperthermia after cardiopulmonary bypass, pain, and vanished effects of anesthetic drugs leading to arousal after anesthesia” superimpose the potential underlying factors which may lead to unwanted CNS complications. The main postoperative risk factors are categorized in Table 37.3 (Hsia and Gruber 2006; Lee et al. 2008; Massaro et al. 2008; Albers et al. 2010; Kaltman et al. 2010).
Table 37.3
Postoperative risk factors affecting CNS outcome in neonatal and pediatric congenital heart surgery
Risk factor | How to prevent or compensate |
---|---|
Impaired CNS autoregulation | Hemodynamic stability and prevention of hypotension; ensuring adequate CNS oxygen delivery; prevention of low hematocrit levels; ensuring adequate ventilation and oxygenation |
Clinical seizure and/or electroencephalographic (EEG) seizure (i.e., nonconvulsive seizure) | Continuous EEG monitoring; especially for patients at risk of seizure or with underlying CNS disorders; if seizure starts from frontal lobe, a worse “Psychomotor Developmental Index” is predicted in patients under 1 year |
Postoperative hyperthermia | Even mild degrees of hyperthermia in ischemic CNS regions could have considerable and significant impacts on CNS status; these deleterious effects should especially be avoided in patients undergoing DHCA; CNS tissue is highly sensitive to ischemia and temperature changes especially during pre-bypass period and early post-bypass (Shum-Tim et al. 1998; Nussmeier 2005; Shamsuddin et al. 2015; Hu et al. 2016) |
Postoperative arterial and/or venous desaturation | Optimizing oxygenation, ventilation, hemodynamics, and hematocrit level prevents systemic desaturation; residual shunts or single ventricle anatomy affect significantly the level of postoperative saturation; systemic venous desaturation is an important indicator of decreased systemic oxygen delivery; postoperative monitoring with both “cerebral NIRS” and “somatic NIRS” could be a useful guide to foresee this event |
Single ventricle anatomy | Increases the risk of arterial hypoxemia; also, there is increased chance for systemic emboli; all are potential risk factors for postoperative CNS injuries; respiratory maneuvers, preventing hyperventilation and hypocapnia and stable hemodynamics, could help decrease the risk; also, cerebral and somatic NIRS could be of use (Dehaes et al. 2015). Goldberg et al. demonstrated impaired neurodevelopmental state in children with single right ventricle anatomy at 3 years of age (Goldberg et al. 2014) |
Increased intracranial pressure (ICP) | Increased ICP leads to decreased cerebral perfusion pressure; head elevation and other maneuvers for prevention of ICP increase should be used |
Low cardiac output state (LCOS) | LCOS is predicted to occur 6–18 h after surgery and occurs in 25 % of infants and neonates undergoing congenital heart surgery; residual defects like hypoplastic left heart syndrome are associated with increased chance of LCOS and hemodynamic support with pharmacologic agents (including but not limited to milrinone, dobutamine, and levosimendan) could be used to treat LCOS |
Role of oxidative stress on CNS injury and refractory seizure | |
ICU length of stay | |
Mechanical support/heart transplantation | Patients receiving mechanical support both in preoperative period or during postoperative care (either as extracorporeal membrane oxygenation or ventricular assist devices) are at risk of hemodynamic instability; besides, they are at increased risk of thromboembolic events; on the other hand, patients with heart transplantation are at increased risk for worse CNS outcome (Massaro et al. 2008; Marino et al. 2012; Rollins and Newburger 2014) |
Classification of CNS Deficits
The risk of neurologic injuries and adverse neurodevelopmental outcomes is higher in neonates and infants with congenital heart disease (Sherlock et al. 2009). In these patients, CNS deficits are categorized under three main classes:
Neurologic deficits
Neurocognitive and neurodevelopmental disorders
White matter injuries (WMI) including periventricular leukomalacia (PVL)
Neurologic Deficits
Neurologic injuries are a common finding in neonates and infants with underlying congenital heart diseases; also, these lesions are among the most important perioperative complications in these patients. The following are among the most common neurologic disorders in these patients (Kinney et al. 2005; Chen et al. 2009; Sherlock et al. 2009; Block et al. 2010; Gaynor et al. 2015):
White matter injuries (including periventricular leukomalacia or diffuse white matter gliosis)
Stroke with a 10 % prevalence; half occurring preoperatively (Chen et al. 2009)
Seizures
Intraventricular hemorrhage (IVH)
Visual disturbances
Gray matter lesions
Focal neurologic injuries
Neonatal stroke and periventricular leukomalacia (PVL) are the two most significant lesions in patients undergoing congenital heart surgery (Sherlock et al. 2009); however, seizures, either convulsive or nonconvulsive, are among the most serious neurologic complications after congenital heart surgery in neonates and infants; nonconvulsive seizure presenting only as EEG seizure activity is much more common than convulsive seizures in this patient population (Abend et al. 2013).
Neonatal Seizure
Neonatal seizure still remains a great challenge and a clinical dilemma; among the main reasons for this fact, the following could be mentioned:
Ambiguous presentations.
Failure of immediate detection.
Paucity of evidence-based management protocols, especially regarding the standard treatment.
Poor outcomes.
Adverse neurodevelopmental outcome and epilepsy are the main considerations as the aftermath of neonatal seizure in the long term, mandating careful follow-up for neonatal seizure patients.
The incidence of neonatal seizure is the highest among all age groups: 1.5–3 per 1000 live births (Silverstein and Jensen 2007; Jensen 2009).
Etiology
Among all the above, hypoxic–ischemic encephalopathy (HIE) is the etiology of neonatal seizure in about two-thirds of patients (Jensen 2009).
However, these different etiologies lead to different severities of the disease and diverse outcome; some of the seizures with non-HIE etiologies have good outcomes, while the outcome in HIE is much worse. So, underlying mechanism in neonatal seizure remains a crucial issue in diagnosis and treatment of neonatal seizure (Kang and Kadam 2015).
Risk factors for seizure in neonatal and pediatric patients undergoing cardiac surgery are diverse and heterogeneous; however, the main risk factors in this specific patient population include:
Diagnosis
In diagnosis of neonatal seizure, the availability of both amplitude-integrated EEG (aEEG) and standard EEG are very decisive; unless we use aEEG in NICU, a considerable part of patients with neonatal seizure would be undetected (Boylan et al. 2015; Kang and Kadam 2015). aEEG is a bedside tool for neurophysiologic assessment; uses fewer channels than standard EEG; so, aEEG is much easier, both regarding its use and interpretation and could help earlier diagnosis much more than conventional EEG; however standard EEG is more sensitive for diagnosis of seizure. However, it is not a mandatory that aEEG be interpreted by a neurologist. Of course, standard EEG remains the decisive method of diagnosis for neurophysiologic assessments. A full discussion on CNS monitoring tools including EEG could be found in Chap. 9 “CNS Monitoring.” For long-term brain imaging and follow-up studies, MR images are one of the best available options; long-term outcome differs based on the underlying etiology; hence, long-term follow-up is a basic rule.
Treatment
Though a considerable number of studies have been done to determine the best therapeutic protocol for neonatal seizures, satisfactory evidence is still lacking; however, phenobarbital is considered as the drug of choice; however, its efficacy and the pharmacologic profile of the drug is still the subject of some studies. The final word in selection of the best available anticonvulsant therapeutic protocol is to be said (Hellstrom-Westas et al. 2015; Kang and Kadam 2015).
Neurocognitive and Neurodevelopmental Disorders
Congenital heart disease increases the risk of neurodevelopmental performance including the scores on intelligence, alertness, executive functions, attention and memory, and possibly the daily life care and school function (Sterken et al. 2015). Since neurodevelopmental and neurocognitive aspects of care are of great concern, clinicians have tried to improve this aspect of postoperative outcome; however, difficulties in daily functioning and academic achievements in such patients still remains a problem (Gaynor et al. 2015).
Neurocognitive disorders are categorized under a common framework in DSM-5. Generally, the main characteristic of neurocognitive disorders is “a decline from a previously attained level of cognitive function” and includes “delirium, mild cognitive impairment and dementia” (Hsia and Gruber 2006; Snookes et al. 2010; Sachdev et al. 2014; Simpson 2014). Based on DSM-5 classification, there are six neurocognitive domains:
- 1.
Perceptual motor function: visual perception, visuoconstructional reasoning, and perceptual motor coordination
- 2.
Language: object naming, word finding, fluency, grammar and syntax, and receptive language
- 3.
Learning and memory: free recall, cued recall, recognition memory, semantic and autobiographical long-term memory, and implicit learning
- 4.
Social cognition: recognition of emotions, theory of mind, and insight
- 5.
Complex attention: sustained attention, divided attention, selective attention, and processing speed
- 6.
Executive function: planning, decision making, wording memory, responding to feedback, and inhibition flexibility
For assessment of cognitive function, both clinical neurodevelopmental testing and follow-up MRIs are of great importance; these two remain the main surrogate outcome measures in the aftermath of congenital heart surgeries (Kaltman et al. 2010). For example, there is a specific type of brain injury seen in pediatric patients undergoing congenital heart surgery described by Soul et al.; they found subtle hemorrhagic events, demonstrated in brain MRI as “foci of hemosiderin”; in their study, these lesions were found in postoperative brain MRI, and they described them as small hemorrhagic lesions with the etiology being different from the etiology of hypoxic–ischemic lesions or periventricular leukomalacia. These subtle hemorrhagic foci could be detected by exact MRI studies during serial assessments; also, they have an adverse impact on neurodevelopmental outcome of the children undergoing congenital heart surgery (Soul et al. 2009; Albers et al. 2010).
White Matter Injuries (WMI) Including Periventricular Leukomalacia (PVL)
During recent years, the improved quality of care in NICUs has led to improvements in outcome in some aspects of white matter injuries (WMI); however, there are still areas which are not much well managed. For a better understanding, let’s first review the current classification for WMI based on the many of studies performed in this area, both on animal and human samples (Segovia et al. 2008; Buser et al. 2010; Back et al. 2012; Dean et al. 2013; Back 2014, 2015; Back and Miller 2014; McClendon et al. 2014; van Tilborg et al. 2016).
WMI occurs in two forms:
Selective death of premyelinating oligodendrocytes is more common and involves widespread lesions which are often detected in autopsies and yield to microscopic cysts; however, they are not very well defined by MRI; this type of lesions leads to widespread renewal of premyelinating oligodendrocytes; then leading to reactive gliosis, the final outcome is arrest in maturation of premyelinating oligodendrocytes.
The other form is widespread “pan-cellular death and necrosis” resulting in cystic PVL and larger lesions compared with the first group; this type of lesion leads to glial and axonal loss.
Both of these lesions lead to “myelination failure” which is discussed more in the next paragraphs.
Definition of PVL
Necrosis in deep white matter, which is presented as multifocal symmetrical necrosis areas, often known as cystic PVL or cPVL; the process of necrosis involves premyelinating oligodendrocytes; these necrotic areas are often adjacent to the external angles of the lateral ventricles (Rezaie and Dean 2002; Back 2014; McClendon et al. 2014).
Etiology (Including Predisposing Factors and Mechanisms of Injury)
Risk Factors
These are among the mostly reported risk factors for PVL (Herzog et al. 2015; Shang et al. 2015):
Prematurity
Preterm infants
Gestational age of ≤32 weeks
Perinatal infections (especially chorioamnionitis)
Perinatal hypoxia and/or respiratory distress
Low-birth-weight infants
Maternal obesity
Preterm premature rupture of the membranes (PROM)
Also, there seems to be a negative correlation between gestational age and birth weight (Shang et al. 2015). Resch et al. in a cohort study found “respiratory distress syndrome, preterm premature rupture of the membranes, and chorioamnionitis” as the most common clinical findings associated with PVL (Resch et al. 2015). Chorioamnionitis has been proposed as a risk factor for PVL in many studies, mainly due to its inflammatory effects; however, some controversy exists (Wu and Colford 2000; Chau et al. 2014; Herzog et al. 2015).
Mechanism of Injury
Decreased perfusion of the periventricular areas during systemic hypotension and inflammatory responses during the perinatal period has been proposed as the two main mechanisms of injury for PVL; in infants with PVL, higher serum levels of interleukin-1 beta (IL-1β) and tumor necrosis factor-alpha (TNF-α) have been demonstrated, which are accompanied by other proinflammatory mechanisms leading to white matter damage in PVL, while antenatal steroid might suppress the prevalence of PVL (Canterino et al. 2001; du Plessis and Volpe 2002; Rezaie and Dean 2002; Kohelet et al. 2006; Tsukimori et al. 2007; Hagberg et al. 2015; van Tilborg et al. 2016). New insights to inflammatory mechanisms of PVL are in the way: Vontell et al. demonstrated increased expression of glial toll-like receptor 3 (TLR3) protein in infants with PVL which could result in impaired development in preterm infants (Vontell et al. 2013, 2015).
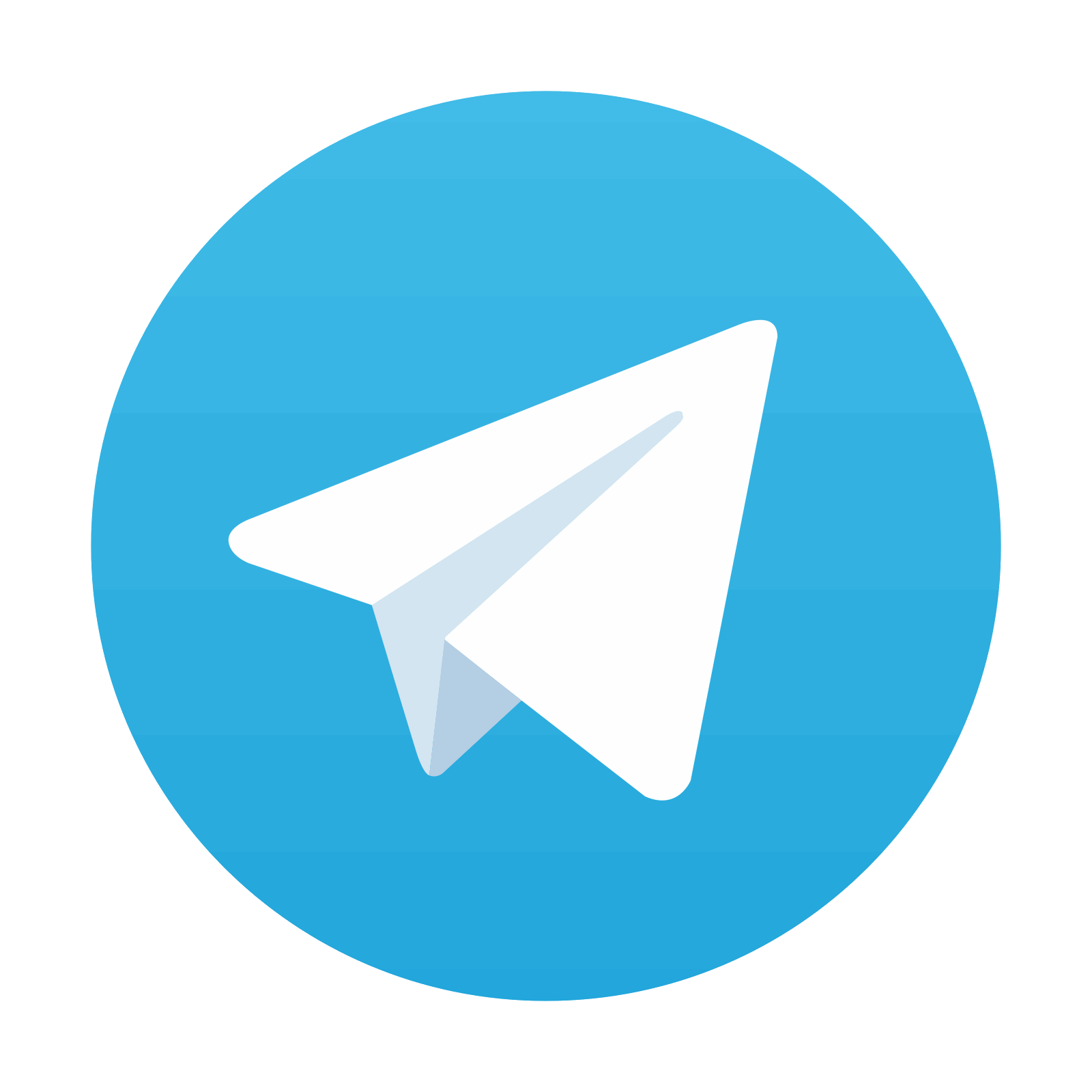
Stay updated, free articles. Join our Telegram channel

Full access? Get Clinical Tree
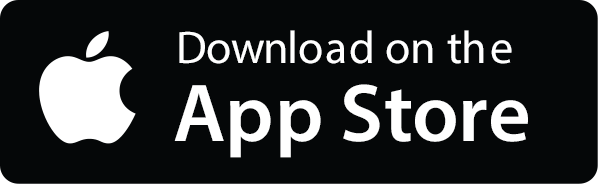
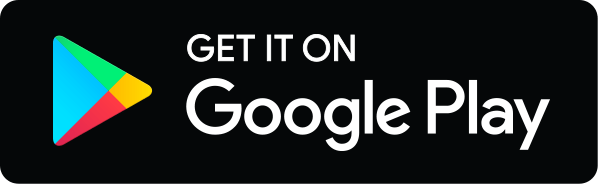
