Obesity and Metabolic Syndrome
Oscar C. Marroquin
David E. Kelley
Introduction
Obesity now looms as a major public health challenge for developed societies. Cardiovascular disease (CVD) is one of the major comorbidities of obesity. The relationship between obesity and CVD is the focus of this chapter. Physicians need to integrate an assessment of obesity into patient evaluation and also promote its treatment. These principles pertain to the management of many cardiovascular illnesses and emphatically to the promotion of cardiovascular health. One of the goals of this chapter is to outline current practices for the assessment of obesity. These are simple and straightforward. The pathophysiology that links obesity with the metabolic syndrome will also be addressed, stressing the importance of regional distribution of adiposity as well as the roles of adipose tissue as an endocrine organ and a source of low-grade inflammation. Central adiposity and the closely associated phenomena of increased fat content within liver and skeletal muscle are at the core of the metabolic syndrome and insulin resistance. Delineation of the metabolic syndrome was a major step forward in the conceptualization of how insulin resistance and obesity contribute to risk for CVD. Recently, controversy has arisen as to whether the metabolic syndrome actually constitutes a treatable disease entity.
The second main aim of this chapter is to examine the mechanisms and manifestations of CVD in obesity. There are multiple pathways through which increased risk occurs. Risks for CVD associated with obesity and insulin resistance coalesce into propensity for the formation, progression, and, likely, the fragility of atherosclerotic plaques, and thus into an increased risk for coronary thrombosis and myocardial infarction. However, this is not the singular manifestation of obesity-related risk for CVD. Obesity-related risk for cardiovascular disease is also manifest as congestive heart failure, endothelial dysfunction, arterial stiffness, hypertension, and perturbations of substrate metabolism within cardiac myocytes, and these aspects will also be addressed in this chapter.
Epidemiology
During the last several decades, the prevalence of obesity has increased to epidemic proportions. The prevalence of obesity among U.S. adults increased from 12% in 1991 to 21.3% in 2002 (Fig. 3.1) (1). In 2002, 58% of adult Americans (66% of men and 50% of women) were overweight (2). Overweight is defined as a body mass index (BMI) above 25 kg/m2 but less than 30 kg/m2, and obesity is defined as a BMI of 30 kg/m2 or greater. The prevalence of class III obesity, a BMI greater than 40 kg/m2, increased from 0.9% in 1991 to 2.4% in 2002 (3). There are ethnic differences in obesity. African Americans and Hispanics have higher rates of obesity than do non-Hispanic whites, whereas the prevalence of obesity is lower among Asian Americans (4), although the BMI at which obesity-related comorbidities may develop appears to be lower in Asian Americans, so cut-points for “at-risk” BMI are lower. Of particular concern is the widespread increase in the prevalence of overweight and obesity among school-age children as well as adolescents and young adults (5,6). These trends have been observed in the United States (2,5,6), Great Britain (7), and Asia (8), where similar behavioral factors affecting diet and physical activity are presumably at work.
Clinical Assessment of Obesity
In the United States, the National Institutes of Health bases its classification of body weight on the concept of body mass index (9). The BMI is calculated by dividing the body weight in kilograms by the square of the height in meters (Table 3.1).
A lean BMI is 25 kg/m2 or less. Although a lean body weight is regarded as “normal,” as pointed out earlier, this is maintained in only a minority of adults in the United States. In children and adolescents, no specific BMI values have beenset
to identify obesity and overweight; however, BMI at or greater than the 95th percentile for sex- and age-specific distribution has been used to identify overweight (2).
to identify obesity and overweight; however, BMI at or greater than the 95th percentile for sex- and age-specific distribution has been used to identify overweight (2).
In addition to measuring height and weight (and thereby determining BMI), measuring waist circumference is also recommended. At any BMI, a higher waist circumference identifies increased metabolic risk. Although the relationship is a continuum, a cut-point for waist circumference is greater than 102 cm (>40 inches) for men and greater than 88 cm (>35 inches) for women. Measuring waist circumference takes into account the distribution of adipose tissue. For example, for the same level of BMI, the cardiovascular risk may be significantly lower in a person in whom excess body fat is predominantly in a gluteal-femoral distribution rather than upper body, abdominal adiposity (10,11,12).
Assessment of Fat Mass
More-precise measurements of the amount of body fat and more exact ascertainment of its distribution generally exceed cost constraints as well as practical needs for clinical care. In a research setting, there are several methods that can be used to accurately assess fat mass and ascertain the distribution of adipose tissue, including assessments of the lipid content of liver and skeletal muscle. Estimation of fat mass (and of fat-free mass) is usually obtained using dual-energy x-ray absorptiometry (DXA). A limitation is that DXA is not adequate for severe obesity due to the size constraint of the scanning table. The use of underwater weighing to estimate fat mass has waned with the widespread availability of DXA. Air-displacement systems that permit determination of body density have become available. Thus, calculation of fat mass is beginning to be more common (e.g., the BOD POD technology; Life Measurement Inc., Concord, CA). Bioimpedance can be used to estimate total body water based on resistance to a very small amount of transmitted electrical current. Using assumptions about tissue hydration, one can estimate fat-free mass and fat mass. This last point is crucial for understanding the limitations of bioimpedance because rapid shifts in fluid balance, as might occur with treatment of congestive heart failure, can render these estimations inaccurate.
TABLE 3.1 Clinical Classification of Obesity | ||||||||||||
---|---|---|---|---|---|---|---|---|---|---|---|---|
|
Assessment of Regional Distributions of Adipose Tissue
The importance of adipose tissue distribution in relation to metabolic risk was recognized by Vague et al. (13) more than a half century ago. This perspective has been substantiated by the findings obtained using modern bioimaging technologies for body composition, such as computed tomography (CT), magnetic resonance imaging (MRI), and magnetic resonance spectroscopy (MRS). Abdominal CT or MRI, most commonly performed using a cross-sectional image centered at the L4–5 disc space, is generally used to measure visceral abdominal adiposity (visceral adipose tissue, VAT) and subcutaneous abdominal adiposity (SAT) (Fig. 3.2) (14,15,16). Visceral adiposity generally is greater in men than in women and increases with aging and after menopause in women. As a percentage of total abdominal adiposity and in relation to BMI, VAT varies across races, being higher in Asians, lower in Africans, and intermediate in those of European ancestry. Visceral adiposity is the sum of omental, mesenteric, and perinephric adipose tissue, essentially all adipose tissue beneath the abdominal wall musculature.
![]() FIGURE 3.2. Cross-sectional image abdominal CT at the L4–5 disk space to measure visceral adiposity. |
In general, the amount of VAT correlates well with severity of insulin resistance and with dyslipidemia (14,15,16). That visceral adiposity correlates with metabolic syndrome more strongly than does an overall index of obesity such as BMI or systemic fat mass is an intriguing because the volume of VAT generally represents only about 10% of systemic adipose tissue. It remains incompletely understood why this relatively small mass of VAT correlates so well with systemic manifestations of insulin resistance, including various risk factors for CVD. VAT, or at least a portion of VAT, releases free fatty acids (FFAs) into the portal circulation (17). The strong association between VAT and severity of dyslipidemia may relate in part to this portal delivery of fatty acids, although this is a difficult concept to test in humans due to inaccessibility of portal sampling. Adipocytes within VAT do have a higher rate of lipolysis and are less responsive to suppression of lipolysis by insulin (18,19). Nonetheless, this explanation is at best only partial satisfactory because a majority of systemic FFA flux derives from subcutaneous adipose tissue and in particular abdominal subcutaneous adipose tissue (20).
The strong correlation between VAT and insulin resistance has been an impetus to regard regional depots of adipose tissue as distinct or individual “miniorgans,” each with important individual contributions. This discussion needs to take into account not only “metabolically adverse” depots such as VAT, but also “metabolically protective” depots. Gluteal-femoral adiposity poses little risk for insulin resistance; in fact, this depot appears to mitigate risk (21,22,23). A metabolic basis appears to be that adipocytes within the subcutaneous adipose tissue of the gluteal-femoral region has a lower rate of lipolysis than adipocytes of omental and mesenteric adipose tissue and of adipocytes within subcutaneous adipose tissue of the abdomen. Indeed, metabolic risk associated with gluteal-femoral adiposity appears to be associated with paucity rather than an excess (21,22,23). A decrease in gluteal-femoral adiposity is a risk factor for hyperglycemia and type 2 diabetes (24,25,26). Recent studies from the author’s laboratory indicates that matched for BMI and fat mass, men and women with type 2 diabetes have less gluteal-femoral adiposity. Also of interest is that in response to the peroxisome proliferator activated receptor (PPAR)-γ glitazones, insulin sensitivity of gluteal-femoral adipose tissue is increased in patients with type 2 diabetes, and this increase correlates strongly with improved systemic insulin sensitivity (27).
A few clinical investigations reported that abdominal subcutaneous adiposity is more strongly related to insulin resistance than visceral adiposity (28,29,30), although most studies found a stronger association for visceral adiposity (31,32,33). There may be several reasons for this ambiguity. First, there are two layers that comprise abdominal subcutaneous adipose tissue, and these differ in metabolism and association with insulin resistance (34). The outer layer, termed superficial abdominal subcutaneous adipose tissue, is larger in women than in men and seems similar to gluteal-femoral adiposity; it seems to be benign or even protective against insulin resistance. The inner layer, termed deep abdominal subcutaneous adipose tissue, is larger in men than in women, and correlates much more strongly with insulin resistance, and in a manner quite similar to that of VAT (34). Thus, part of the reason for differing patterns of association between abdominal subcutaneous adipose tissue and insulin resistance may relate to differing proportions of the superficial and deep layers. A second reason for potential ambiguity concerning the relationship between abdominal subcutaneous adiposity and insulin resistance concerns the effects of adipocyte size. Preceding the contemporary emphasis on characterizing obesity by assessing patterns of adipose tissue distribution, obesity research centered on assessments of adipocyte size and number (35). Obesity is associated with larger adipocytes (36,37). Adipocyte size in abdominal subcutaneous adipose tissue is a risk factor for type 2 diabetes even after adjustment for effects of overall obesity and central adiposity (38,39). Large adipocytes have higher rates of lipolysis and may have altered patterns of adipokine secretion that contribute to insulin resistance.
In certain respects, the differing relationships between superficial and deep abdominal subcutaneous adipose tissue with insulin resistance are analogous to the differing relationships of VAT and subcutaneous adipose tissue with insulin resistance. This comparison can be further developed by considering the role of intermuscular adipose tissue in the leg. Most of the adipose tissue in the leg is contained in subcutaneous adipose tissue, and as discussed earlier, this is gluteal-femoral adipose tissue, which is actually protective against insulin resistance. A smaller proportion, approximately 5% to 15%, concerns adipose tissue located immediately adjacent to muscle and beneath the fascia. The amount of subfascial adipose tissue correlates with insulin resistance (40). At the whole-body level, the amount of subfascial adipose tissue surrounding skeletal muscle may approximate the amount of VAT. It is not clear how subfascial adipose tissue surrounding skeletal muscle contributes to insulin resistance. Insulin regulation of lipolysis may be different in this depot than in subcutaneous adipose tissue, potentially exposing muscle to high concentrations of fatty acids and interfering with glucose uptake. Another possibility is endocrine activity of subfascial adipose tissue, although there are little direct data testing this hypothesis.
Metabolic Basis of Obesity-Related Insulin Resistance
Studies of the relationship between insulin resistance and the topography of adipose tissue distribution are quite interesting for probing the relationship between body composition and metabolic complications of obesity. However, these investigations do not of themselves delineate the mechanisms by which adiposity triggers insulin resistance. There are several mechanisms that can be considered: the effects of fatty acids,
adipokines, and inflammation. These are not mutually exclusive. It is likely that all are operative and interactive. Another important consideration concerns the accumulation of fat within liver and skeletal muscle.
adipokines, and inflammation. These are not mutually exclusive. It is likely that all are operative and interactive. Another important consideration concerns the accumulation of fat within liver and skeletal muscle.
Glucose and Fatty Acid Competition
Most tissues, and perhaps especially skeletal muscle and cardiac muscle, can oxidize and store both fatty acids and glucose. Indeed, these two substrates compete for utilization, and this competition, or at least uncontrolled competition, is central to the pathogenesis of insulin resistance. More than 40 years ago, Randle and colleagues established in a series of biochemical studies that fatty acids compete with glucose for utilization by skeletal muscle and that increased availability of fatty acids induces resistance to the effect of insulin in stimulating glucose metabolism by skeletal muscle (41). One of the key functions of insulin is to control this competition and keep this within healthy boundaries. Increases of insulin, as are triggered in response to increases in plasma glucose from food ingestion, suppresses lipolysis in adipocytes, dramatically lowers plasma FFA, and lessens competition to the utilization of glucose. Normally, in the transition from the fasted to the fed state, adipose tissue shifts from FFA efflux to FFA uptake. and systemically the marker of this transition from catabolism to anabolism is a pronounced suppression of plasma fatty acid levels (42). In metabolic studies, elevation of plasma FFA above fasting levels, as can be achieved by lipid infusions, induces fairly severe insulin resistance (43,44,45). The metabolic profile of impaired glucose metabolism that is induced is characterized by decreased glucose transport, reduction of glucose oxidation, and impaired stimulation of glycogen formation. This metabolic profile matches precisely the abnormalities that characterize those found in type 2 diabetes and in obesity (46). Moreover, the induction of insulin resistance does not even require “elevation” of fatty acids. A decade ago, in the author’s laboratory, a metabolic investigation in healthy, lean volunteers revealed that simply preventing the normal suppression of plasma FFA that occurs in response to insulin is sufficient to induce skeletal muscle insulin resistance (45
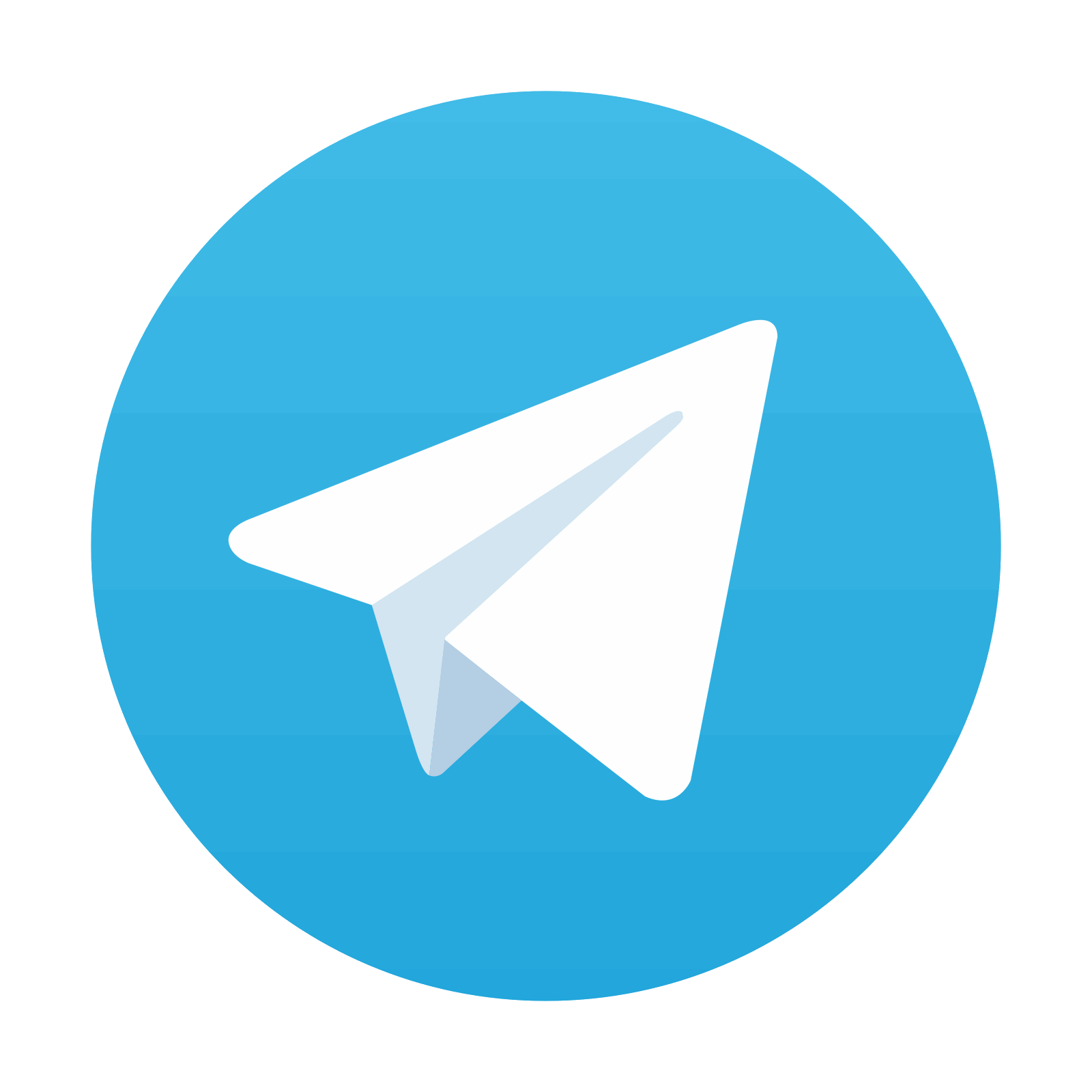
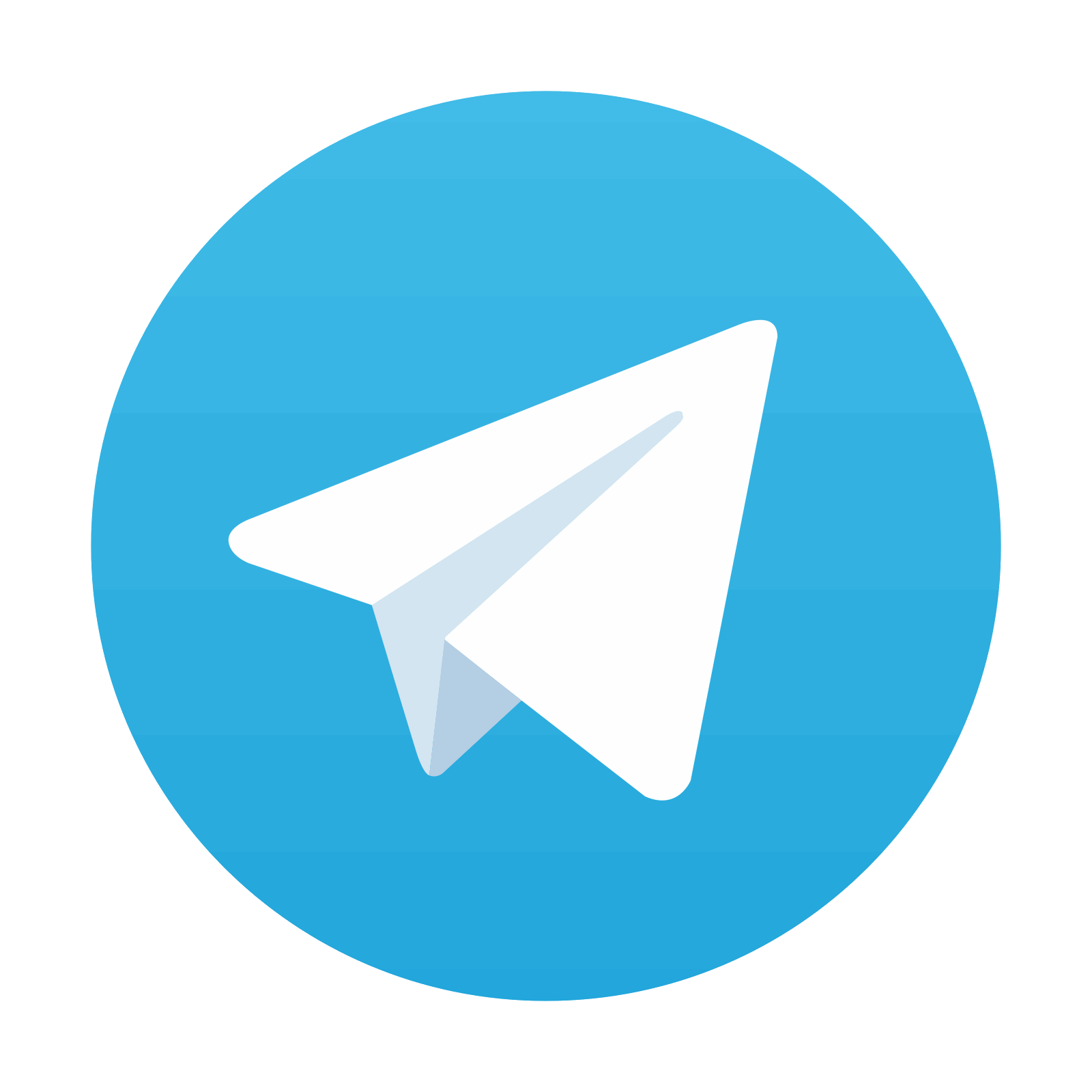
Stay updated, free articles. Join our Telegram channel

Full access? Get Clinical Tree
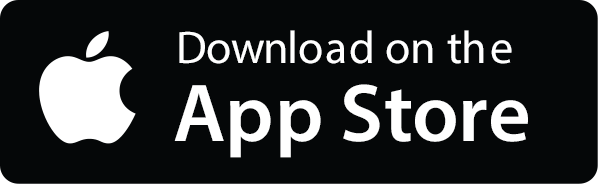
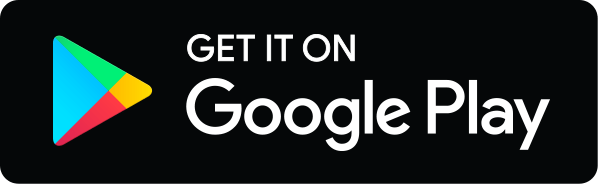
