Fig. 4.1
Ciliary electron microscopy showing a normal cross section and various ultrastructural defects causing PCD. ODA outer dynein arm, IDA inner dynein arm. Reprinted from Leigh MW, Pittman JE, Carson JL, Ferkol TW, Dell SD, Davis SD, et al. Clinical and genetic aspects of primary ciliary dyskinesia/Kartagener syndrome. Genet Med. 2009 Jul;11(7):473–87, with permission of Nature Publishing Group
Accurately processing and interpreting ciliary samples with EM requires training and expertise [5]. A standardized approach for processing these samples is limited to only a few tertiary care centers. Recent studies have demonstrated that ciliary ultrastructure may be normal in some cases of PCD confirmed by genetic testing [6]. Therefore, more emphasis has been placed on other tests, such as nasal nitric oxide measurement and functional ciliary analysis with videomicroscopy, that were originally used as “screening tests” for PCD and now are gaining momentum as “adjunctive diagnostic tests” for PCD at specialized PCD Research and Clinical Centers. With further standardization, nasal nitric oxide testing and/or functional ciliary analysis could be extended to other clinical centers.
Nasal Nitric Oxide
Nitric oxide, a colorless, odorless gas, is produced in numerous cells throughout the body, including upper and lower airway epithelial cells, vascular endothelial cells, neuronal cells, smooth muscle cells, fibroblasts, and macrophages. Nitric oxide gas is formed when l-arginine is oxidized to l-citrulline through the enzymatic action of nitric oxide synthase and a cofactor of nicotinamide adenine dinucleotide phosphate (NADPH). Concentrations of nitric oxide within the nasal cavity and paranasal sinuses are typically 10- to 100-fold greater than exhaled nitric oxide concentrations from the lower airways [7]. In PCD, exhaled and nasal nitric oxide levels are frequently quite low in comparison to healthy controls. A variety of other disorders may be associated with increased or decreased nitric oxide levels. Respiratory tract inflammation due to asthma can cause elevations in both exhaled and nasal nitric oxide levels, while inflammation due to idiopathic bronchiectasis can be associated with increased exhaled nitric oxide levels but nasal nitric oxide may be either increased or decreased [8]. In cystic fibrosis, some patients may have low nasal and exhaled nitric oxide values compared to healthy controls [9]. Sinusitis can increase or decrease nasal nitric oxide levels depending on underlying mechanisms [10, 11].
Evidence for Utility as a Test for PCD
In clinical medicine, nasal nitric oxide measurement is largely limited to evaluation of primary ciliary dyskinesia, as patients afflicted with this disease have consistently low levels. In older children (>5 years of age who can cooperate with testing) and adults with classic PCD, nasal nitric oxide levels are typically well below 77 nL/min [12], while healthy controls typically have levels above 250 nL/min [13, 14] (Fig. 4.2).
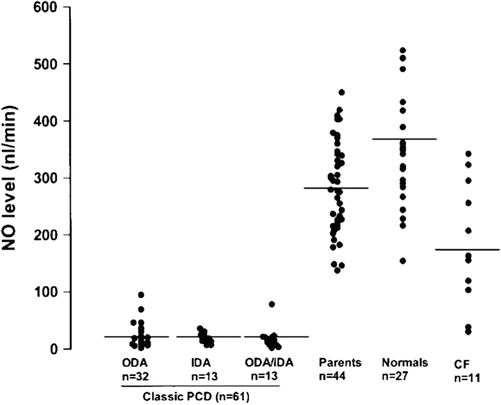
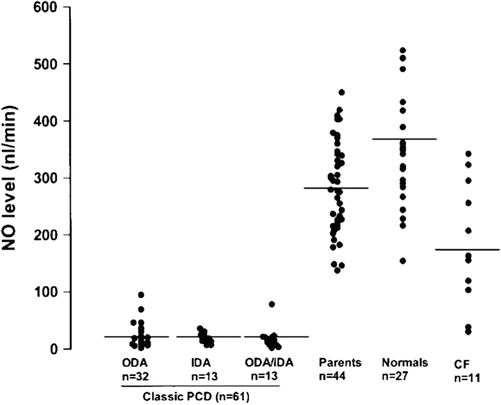
Fig. 4.2
Nasal nitric oxide levels in classic PCD with defects in outer dynein arms (ODA), inner dynein arms (IDA) or both (ODA/IDA) on electron microscopy, Parents of PCD patients, normal healthy controls, and cystic fibrosis (CF). All confirmed PCD cases, but none of healthy controls, fall below 100 nL/min; a few CF patients have values that overlap with PCD. Reprinted with permission of the American Thoracic Society. Copyright © 2013 American Thoracic Society. Noone PG, Leigh MW, Sannuti A, Minnix SL, Carson JL, Hazucha M, et al. Primary ciliary dyskinesia: diagnostic and phenotypic features. Am J Respir Crit Care Med. 2004 Feb 15;169(4):459–67. Official Journal of the American Thoracic Society
Despite intensive research evaluating the etiology for low nasal nitric oxide levels in PCD, the exact mechanism leading to this phenomenon remains unknown [15]. Possible explanations include sinus cavity hypoplasia, increased breakdown of nitric oxide by specific bacteria, decreased production of nitric oxide, or entrapment of nitric oxide in obstructed sinus passages. Others have speculated that decreased expression of inducible nitric oxide synthase, the isoenzyme most responsible for nitric oxide production in airway epithelial cells, may be responsible for the low levels in PCD; however, the etiology for diminished nasal nitric oxide remains inconclusive [16, 17]. Nasal nitric oxide may serve as a fuel for ciliary movement, but no definitive evidence exists to fully support this theory [18]. After administering the nitric oxide precursor l-arginine to cystic fibrosis and PCD patients [19, 20], ciliary activity increased; however, nitric oxide did not increase to normal levels, and pulmonary function did not improve [21]. Future treatment strategies for PCD may include techniques to increase airway nitric oxide levels if a beneficial effect can be demonstrated.
Numerous prospective studies have shown extremely low nasal nitric oxide levels in PCD patients; therefore, this test is now an accepted clinical screening method for PCD in Europe [22–24]. A consensus statement by the European Respiratory Society now includes measurement of nasal nitric oxide in children over 5 years old as a recommended screening test for PCD [25]. Nasal nitric oxide testing is approved for clinical use in Canada, Great Britain, and throughout continental Europe (CE MDD mark). Unfortunately, nasal nitric oxide measurement devices are not yet FDA approved for clinical use in the USA, but this tool is actively used in PCD research centers.
Since its clinical debut, measurement of nasal nitric oxide is becoming a more widespread screening test for PCD. Nasal nitric oxide levels below 62.5–126 nL/min have been reported to have high sensitivity for a PCD diagnosis proven with EM [9, 12, 26, 27]. While nasal nitric oxide is a highly sensitive screening test for PCD, the specificity varies depending on the chosen cutoff value. Current diagnostic strategies set a cutoff value for a positive screening test below 60–100 nL/min in children >5 years old who can comply with blowing into a resistor [12, 27, 28]. Studies have examined various other techniques for nasal nitric oxide sampling, including humming, breath holding, and tidal breathing with the mouth open or closed. All of these techniques can discriminate PCD from non-PCD patients, but each technique requires a different cutoff value for a positive PCD screen [29]. Cutoff values for children <5 years old using the tidal breathing technique are age-dependent and are currently being investigated [29–31].
A low nasal nitric oxide level coupled with the classic phenotype of PCD provides strong support for the diagnosis of PCD that may be confirmed by further diagnostic testing (EM of the cilia and/or genetic testing). This approach can be especially helpful in cases where patients have a PCD causing gene defect with normal EM results, such as in DNAH11 mutations [6]. However, physicians must be aware that other diseases can have similarly low nasal nitric oxide levels. In cystic fibrosis, nasal nitric oxide values can consistently fall below PCD cutoff ranges [8, 9, 19]. Therefore, negative sweat chloride testing or genetic testing for cystic fibrosis is essential when using nasal nitric oxide to guide clinical decision making. Nasal nitric oxide levels can also transiently fall below PCD cutoff ranges in acute respiratory illness, acute sinusitis, diffuse panbronchiolitis, HIV, and nasal polyposis [8–11, 32, 33]. Thus, repeat testing during a healthy time period is also critical to assure that the nasal nitric oxide values are consistent. Perhaps of equal importance, a nasal nitric oxide value that is well in the normal range significantly decreases the likelihood of PCD and should guide the clinician to investigate other diagnoses in the differential.
Equipment
The initial purchase cost for a stationary nasal nitric oxide machine using chemiluminescence technology may exceed $50,000 USD; however, after this initial purchase, nasal nitric oxide testing is relatively inexpensive from a disposable material per test standpoint. Only chemiluminescence technology has been prospectively used and proven effective in PCD screening with nasal nitric oxide. Less expensive electrochemical nitric oxide analyzers have been used to measure exhaled nitric oxide, but their agreement with stationary chemiluminescence analyzers in healthy controls has been inconsistent [34–36]. Efforts are underway to adapt these electrochemical analyzers to measure nasal nitric oxide; however, potential utility as a test for PCD has not yet been fully defined [37]. Use of these electrochemical analyzers requires more patient cooperation to perform controlled expiratory breathing maneuvers; therefore, these electrochemical nitric oxide analyzers have limited utility in children.
Technique
On-line nasal nitric oxide measurement is a rapid and noninvasive screening test for PCD. The entire procedure takes approximately 10 min and results are immediately available. To perform this test, a plastic catheter with a surrounding soft nasal sponge or olive is placed just deep enough into one nare to ensure an air-tight seal. Children who are able to comply with directions (typically over 5 years old) perform maneuvers to close their velum, which prevents dilution of nasal gas (containing much higher nitric oxide) with gas from the lower airways. These maneuvers include blowing into a resistor or a child’s party favor which is partially sealed at the end for at least 10 s. Alternative approaches to decrease dilution of nasal gases that can be used in cooperative children and adults include a 30 s breath hold, pursed lip exhalation via the mouth, or voluntarily closing the velum by making a repeated “ka” sound [24] (Fig. 4.3). This technique produces an initial washout phase followed by a nitric oxide concentration plateau phase, which signifies steady state nitric oxide sampling from the nasal cavity (Fig. 4.4).
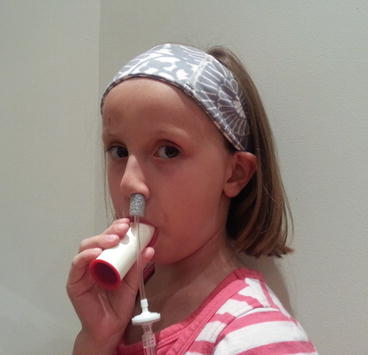
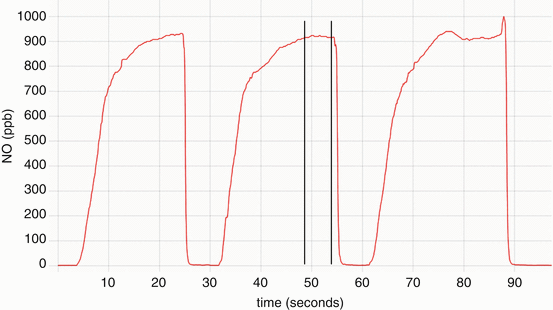
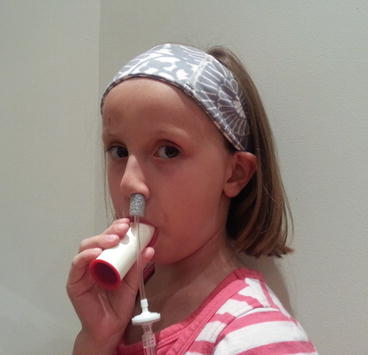
Fig. 4.3
Subject performing a nasal nitric oxide measurement by blowing into a resistor while the probe is securely in the naris. Provided by A. Shapiro, Montreal Children’s Hospital
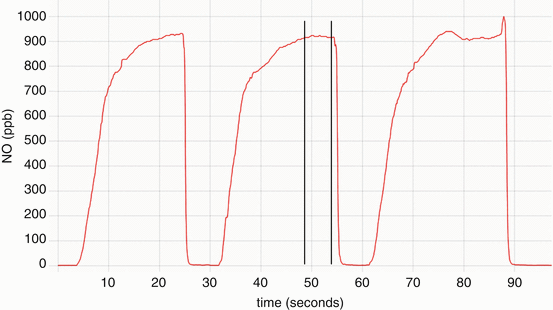
Fig. 4.4
Nitric oxide concentration (parts per billion) versus time (seconds) graph of a nasal nitric oxide measurement obtained while blowing into a resistor. Note the concentration reaches a plateau of at least 3 s, where the measurement is obtained (delineated by the vertical lines). Provided by A. Shapiro, Montreal Children’s Hospital
The same procedure is repeated in the contralateral naris; values are expressed in nanoliters per minute, which are a product of the concentration (in parts per billion) and the flow rate of transnasal airflow in the sampling catheter (in liters/minute) yielding nasal nitric oxide production values (in nL/min). The mean of three separate plateau measurements is calculated for each naris, followed by calculation of the mean for both nares together to yield a final test result. Reporting nasal nitric oxide values as a volume per unit time rather than a simple concentration is preferred [24]. Current chemiluminescence devices have various flow sampling rates that establish a steady state phase of nitric oxide in the sampling tube; nasal nitric oxide concentration is inversely proportional to transnasal flow rates [38]. Very high airflow rates can cause airflow turbulence, which can significantly alter the nitric oxide sampling results [39]. Thus, a standard transnasal airflow sampling rate (0.25–0.5 L/min in children) is currently recommended [24].
Several other testing parameters must be monitored in order to ensure adequate results. An air-tight seal of the nasal olive is essential to prevent dilution with ambient air which would result in a falsely low nitric oxide value. Rhythmic movement of the olive in and out of the naris with each breath may signify air leak. Operators may need to hold the olive steady to avoid this movement. Occlusion of the nasal catheter by secretions may cause an abrupt drop in nitric oxide concentration; therefore, operators should monitor for this problem. In children with nasal congestion (a common feature in PCD), secretions may be aspirated into the catheter necessitating several catheter changes during the course of a test. Given this issue, nasal nitric oxide measurements should ideally be performed when the patient is at his or her respiratory baseline. In addition, ambient nitric oxide values should be monitored to ensure that there is no artificial elevation of nitric oxide that could lead to false negative results. Ambient concentrations of nitric oxide should be recorded prior to each test. This screening test should only be performed when subjects are free of viral illness, as viral respiratory infection may affect nasal nitric oxide levels as noted above [40]. Lastly, there may be a normal circadian change in nasal nitric oxide levels, with significantly lower values in the morning and evening [41]. Nasal steroids, nasal decongestants, topical nasal lidocaine, systemic antibiotics, and cigarette smoke may also variably influence nasal nitric oxide levels [42].
Limitations
Recent reports identifying PCD cases with definite ultrastructural defects, but nasal nitric oxide values higher than the usual cutoff levels, have led investigators to consider the possibility of variant forms of PCD [43]. In addition, research has shown that nasal nitric oxide levels can increase with nitrate rich diets, treatment of acute sinusitis, or treatment with chronic macrolide therapy [44–46]. However to date, none of these changes in nasal nitric oxide levels have been reported in patients with PCD. Longitudinal data collected over several years have demonstrated stable nasal nitric oxide values in patients with PCD [47]. Extensive testing of nasal nitric oxide in rare disease controls (such as various immunodeficiencies, heterotaxy, variant cystic fibrosis, and primary ciliopathies) is currently lacking. Since clinical features for many of these diseases overlap with PCD, false positive nasal nitric oxide screening results may occur. Preliminary work has shown intermediate nasal nitric oxide levels in heterotaxy and autosomal recessive polycystic kidney disease patients, despite these patients not fulfilling the classic PCD phenotype [48, 49]. Further studies in non-classic forms of PCD and other associated rare diseases may lead to modification of current cutoff values. Physicians must realize that normal nasal nitric oxide levels may not completely rule out PCD, particularly in cases with chronic sino-oto-pulmonary symptoms.
Next Steps
Employing nasal nitric oxide as a screening test in children under 5 years of age, who cannot cooperate with expiratory resistance maneuvers, will allow for earlier diagnosis and treatment of PCD at a younger age. Earlier treatment may improve long term respiratory outcomes [50]. Tidal breathing measurement techniques are feasible and sensitive for PCD screening in children under 5 years old, but normal nasal nitric oxide values spanning the entire age range of this group are still being determined [29, 51, 52]. Tidal breathing procedural guidelines are also needed to successfully expand nasal nitric oxide testing to this younger population (Fig. 4.5). Children less than 5 years of age have varying normal levels of nasal nitric oxide, with age-dependant production possibly following the degree of paranasal sinus development [53, 54]. Healthy infants have a much lower nasal nitric oxide level than healthy toddlers and preschoolers, but nasal nitric oxide values become age-independent after approximately 5 years of age. Normal values for nasal nitric oxide in healthy infants under 1 year of age often fall within PCD cutoff ranges, which may preclude nasal nitric oxide as an effective screening test in this age group [53].
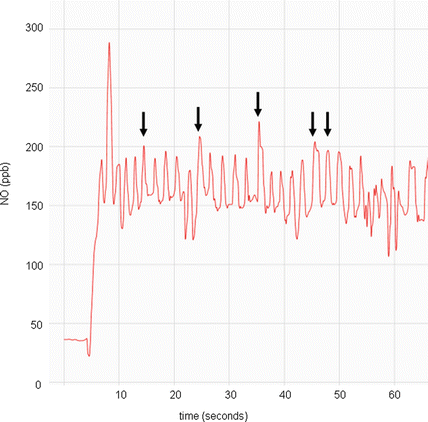
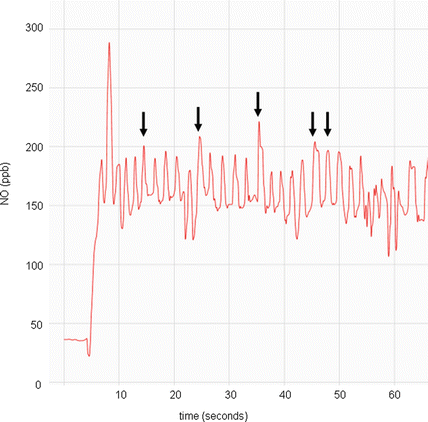
Fig. 4.5
Nitric oxide concentration (parts per billion) versus time (seconds) graph of a nasal nitric oxide measurement obtained during tidal breathing in a child <5 years old. Note the repetitive peaks (arrows), all approximately of the same value, of which 5 will be used to calculate the mean value for one naris. Provided by A. Shapiro, Montreal Children’s Hospital
For children older than 5 years of age, standardized procedures for analyzer calibration and quality control are critical. Standardization of acceptable test maneuvers and maximal allowable ambient nitric oxide concentration are also needed for broad implementation of nasal nitric oxide screening in children. Well-defined exclusion criteria for clinical testing, such as recent epistaxis or acute viral infection, should also be established. Lastly, Food and Drug Administration approval of chemiluminescence nasal nitric oxide analyzers for clinical use in the USA is a major obstacle preventing widespread use of this test in PCD.
Ciliary Videomicroscopy
Functional analysis of ciliary movement with videomicroscopy has become an integral part of PCD diagnosis in several specialized centers. Although functional analysis has been in practice for many years, older diagnostic techniques relied mainly upon calculation of ciliary beat frequency (CBF) [55]. Many clinical centers continue to use subjective light microscopy examination and/or CBF calculation as a screening test for PCD [56]. This methodology can be an arduous process when done manually, thus digital image capture software with automated whole-field analysis is often purchased to calculate CBF [57]. Photomultiplier and photodiode devices, which analyze changes in light intensity passing through beating cilia, also help to automate this meticulous process, but may underestimate CBF [58]. In addition, these automated programs only provide a CBF value and do not analyze ciliary beat pattern.
Clinicians performed initial videomicroscopy beat pattern studies with analog video recorders, which provided vast amounts of recording data and often required days or even weeks of painstaking visual interpretation per study. Maximum recording rates with analog devices are only 30 frames per second, which does not allow measurement of CBF above 15 Hz [59]. With gained experience, experts realized that CBF calculation alone is inadequate for complete PCD evaluation, as this measure is subject to bias through improper specimen preparation, transport, handling, preservation and intercurrent infection. Additionally, forms of suspected PCD that result in normal EM and normal or increased CBF demanded improved functional ciliary analysis techniques. With the advent of digital recording technology, much faster image capture rates became possible, and researchers employed this technology in ciliary analysis. Now able to capture up to 500 frames per second, high speed digital videomicroscopy is used in several European centers to analyze changes in ciliary beat pattern as a diagnostic test for PCD.
Evidence for Utility as a Test for PCD
Respiratory cilia move in a highly regulated and coordinated pattern in order to sweep mucus from the upper and lower airways. Moving in the same plane forward and backward, cilia execute a forward power stroke followed by a recovery stroke (Fig. 4.6). Contrary to older publications, there is no sideways recovery stroke causing a metachronal wave [58].
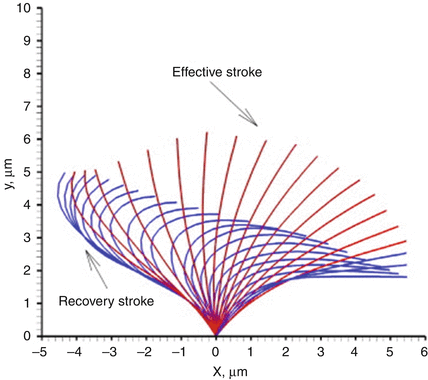
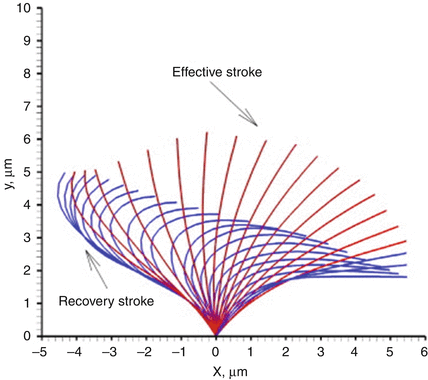
Fig. 4.6
Normal ciliary waveform pattern seen in side profile, demonstrating forward power stroke and recovery stroke. Reprinted from Jayathilake PG, Zhijun T, Le DV, Lee HP, Khoo BC, Three-dimensional numerical simulations of human pulmonary cilia in the periciliary liquid layer by the immersed boundary method, Computers & Fluids, Volume 67, 30 August 2012, Pages 130–137, with permission of Elsevier
Experts now appreciate that some ciliary movement is present in most forms of PCD, but that movement is dyskinetic (Fig. 4.7). Cilia from patients with “classic” defects in outer dynein arms (+/− accompanying inner dynein arm defects) are largely immotile, and if movement is present, it is restricted to a slow, short, stiff flickering motion with a CBF <1 Hz. Inner dynein arm defects (+/− radial spoke defects) have a very abnormal stiff forward power stroke with a markedly reduced amplitude, where cilia fail to bend along the length of the axoneme. Approximately 10–30 % of cilia are fully immotile with inner dynein arm or radial spoke defects, and the remainder can actually have a CBF at the lower end of normal. Central apparatus defects (transposition defect) cause circular ciliary motion when viewed from above, but can appear normal when viewed from the side and move with a normal CBF [60]. Lastly, PCD causing genetic defects in DNAH11 result in reduced bending capacity of cilia, with reduced amplitudes and recovery strokes, despite a greater than normal ciliary beat frequency and normal ciliary ultrastructure on electron microscopy [61].
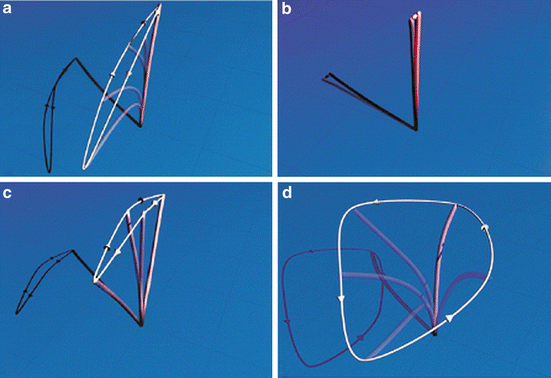
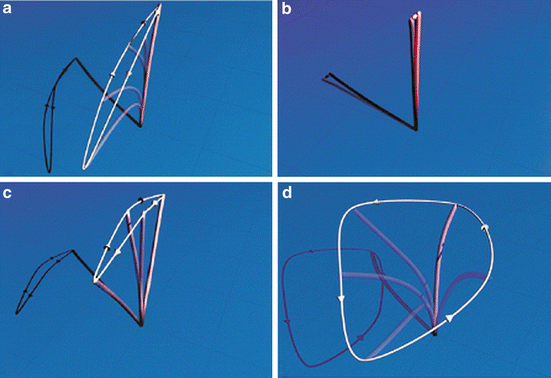
Fig. 4.7
Various ciliary beat patterns as seen on high speed videomicroscopy. (a) Normal ciliary beat pattern; (b) ciliary beat pattern with outer dynein arm defect or outer plus inner dynein arm defects; (c) ciliary beat pattern with inner dynein arm or radial spoke defect; (d) ciliary beat pattern with transposition defect affecting the central apparatus. Reprinted from Chilvers MA, Rutman A, O’Callaghan C. Ciliary beat pattern is associated with specific ultrastructural defects in primary ciliary dyskinesia. J Allergy Clin Immunol. 2003 Sep;112(3):518–24, with permission of Elsevier
High speed videomicroscopy is clearly superior for PCD diagnosis over CBF calculation alone or subjective light microscopy analysis. When making a PCD diagnosis, direct comparison of CBF alone against digital high speed videomicroscopy with beat pattern analysis confirms that using CBF alone will give a false negative result in approximately 15 % of samples [62]. The benefits of high speed videomicroscopy with beat pattern analysis include the ability to capture extremely fast frame rates, which can then be slowed down at a later time for detailed review of ciliary waveforms. Clinicians can actually examine frame-by-frame ciliary motion to detect subtle alterations in ciliary beat pattern. For instance, cilia with inner dynein arm defects, which can be difficult to diagnose even on EM ciliary studies, have abnormal and stiff forward power strokes with reduced beat amplitudes on videomicroscopy [60]. Furthermore, digital videomicroscopy can detect very subtle changes in ciliary waveforms even when EM is completely normal. This technology was essential in discovering the abnormal ciliary beat patterns associated with DNAH11 gene mutations mentioned above [61]. As newer candidate PCD genes are validated, there will likely be more PCD cases with normal EM and normal CBF; thereby, requiring digital videomicroscopy for complete phenotyping.
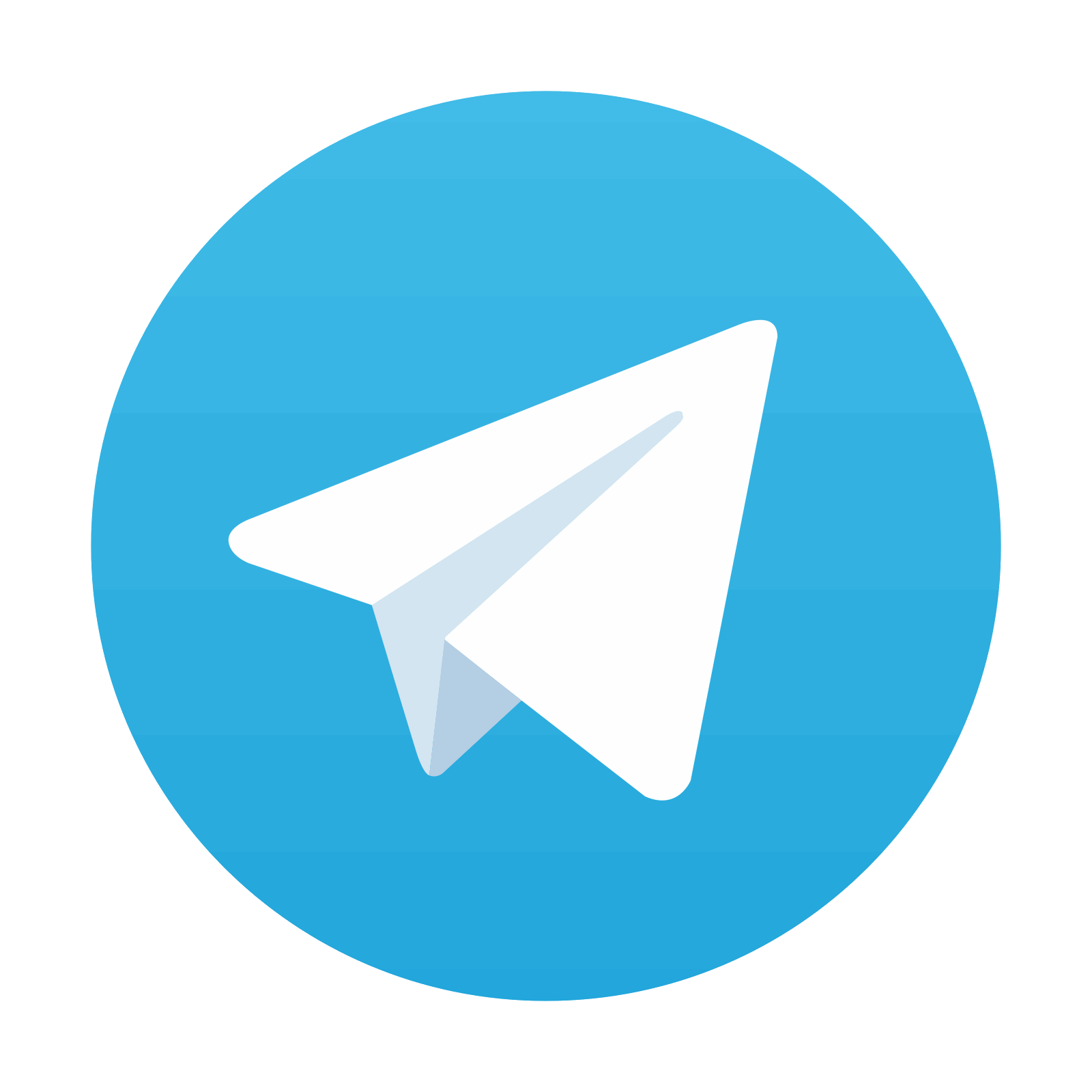
Stay updated, free articles. Join our Telegram channel

Full access? Get Clinical Tree
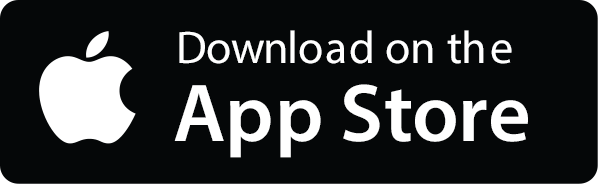
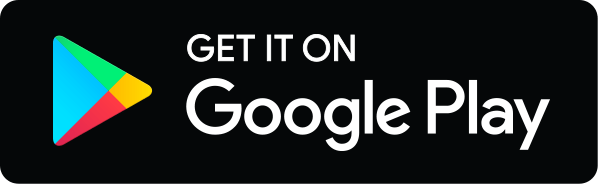