Chapter 39 Myocardial Viability
Comparison with Other Techniques
INTRODUCTION
Coronary artery disease (CAD) is the most common cause of chronic heart failure.1 Over the last decades, significant progress in the treatment of patients with chronic ischemic heart failure has been made. Advances in pharmacologic therapy, the introduction of device therapy, and developments in cardiac surgery have led to improved prognosis in these patients (Table 39-1). Pharmacologic therapy in ischemic left ventricular (LV) dysfunction typically consists of angiotensin-converting enzyme (ACE) inhibitors, β-blockers, and spironolactone and may improve symptoms and clinical outcome. However, pharmacologic therapy is a partial success. In general, patients with chronic ischemic heart failure have a history of myocardial infarction, multivessel CAD, and a variable degree of LV dysfunction. The primary question in these patients is whether they will benefit from surgical revascularization. In patients with a substantial amount of viable myocardium, coronary revascularization may improve heart failure symptoms, left ventricular ejection fraction (LVEF), and long-term prognosis. On the other hand, patients without viable myocardium are unlikely to benefit from surgical revascularization and are at increased risk for perioperative complications.2,3
Table 39-1 Therapeutic Options in Ischemic Cardiomyopathy
Medical Therapy |
ACE inhibitors |
Amiodarone |
AT-II receptor blockers |
β-blockers |
Diuretics |
Digoxin |
Spironolactone |
Despite new drugs, survival on medical therapy is poor |
Device Therapy |
Biventricular pacing |
Internal cardiac defibrillator (ICD) |
Left ventricular assist device (as bridge to transplantation) |
Heart Transplantation |
Limited number of donor hearts does not meet large demand |
Excessive comorbidity in potential recipients |
Good long-term survival |
Surgery |
Revascularization if viable myocardium present, but associated risk is high |
Additional Surgery |
Mitral valve repair |
Left ventricular aneurysmectomy |
Left ventricular restoration |
DEFINITION OF MYOCARDIAL VIABILITY (See Chapter 36)
Patients with ischemic cardiomyopathy often have a variable degree of coexisting hibernating and stunned myocardium and nonviable scar tissue.4 The term myocardial hibernation was first described by Rahimtoola5 to explain the condition of chronic LV dysfunction attributable to chronically reduced blood flow in patients with CAD in whom coronary revascularization may result in recovery of LV contractility. Myocardial stunning refers to reversible myocardial contractile dysfunction in the presence of normal myocardial blood flow at rest.6 Repetitive stunning is a condition that may be caused by repeated ischemic periods inducing chronic dysfunction in the presence of normal or mildly reduced myocardial blood flow at rest; coronary flow reserve, however, is reduced. It has been suggested that a temporal progression exists from stunning, characterized by normal blood flow at rest, to repetitive stunning with normal or mildly reduced blood flow at rest (but reduced flow reserve), to hibernation with reduced blood flow at rest.
Biopsy samples obtained in patients with ischemic LV dysfunction undergoing coronary revascularization revealed that myocardial stunning and hibernation are not just pathophysiologic concepts. Hibernating myocardium shows signs of energy depletion and down-regulation of energy turnover, which may cause and maintain contractile dysfunction, tissue degeneration, and loss of cardiomyocytes.7 Structural dedifferentiation of cardiomyocytes has been observed in hibernating myocardium, characterized by a loss of contractile filaments, small mitochondria, nuclei with uniformly distributed chromatin, and a nearly absent sarcoplasmic reticulum.8 It appears that cardiomyocytes adapt their activity level to prevailing circumstances. This may explain why in hibernating myocardium some characteristics (e.g., contractile reserve) can be lost, whereas more basal characteristics such as cell membrane integrity and glucose metabolism are still intact. Several noninvasive imaging techniques have been developed to probe the different characteristics of dysfunctional but viable myocardium. Some techniques address rather basic characteristics such as cell membrane integrity and glucose metabolism, and other techniques rely on assessment of contractile reserve; this (in part) explains the differences in diagnostic accuracy of the various techniques (Table 39-2). The general term viability includes both hibernation and repetitive stunning but also includes segments with subendocardial scar tissue. These segments are indeed viable, but they contain a mixture of subendocardial necrosis and normal, viable myocardium in the epicardial layers. Whereas both hibernation and repetitive stunning benefit from revascularization (with functional recovery, and potentially improve long-term outcome), dysfunctional segments with subendocardial scar tissue and normal, viable myocardium do not benefit from revascularization.
Table 39-2 Characteristics of Dysfunctional but Viable Myocardium in Relation to Imaging Modalities
Imaging Modality | Characteristics of Viability |
---|---|
Echocardiography at rest | Wall thickness |
Echocardiography with dobutamine | Contractile reserve |
Echocardiography using contrast | Perfusion |
Magnetic resonance imaging at rest | Wall thickness |
Magnetic resonance imaging with dobutamine | Contractile reserve |
Magnetic resonance with intravenous contrast | Scar tissue |
PET or SPECT with FDG | Glucose utilization |
SPECT with thallium-201 | Perfusion and cell membrane integrity |
SPECT with technetium-99m | Perfusion and cell membrane/mitochondrial integrity |
FDG, fluorine-18 2-fluorodeoxyglucose; PET, positron emission tomography; SPECT, single-photon emission computed tomography.
NONINVASIVE IMAGING TECHNIQUES (See Chapters 37 and 38)
Echocardiography
Echocardiography at Rest
A crude differentiation between viable and nonviable myocardium can be made using measurement of end-diastolic wall thickness with echocardiography at rest. Schinkel et al.9 evaluated 150 consecutive patients with ischemic LV dysfunction and demonstrated that the end-diastolic wall thickness was reduced (<6 mm) in 2% of the dysfunctional region and preserved (≥6 mm) in 309 dysfunctional regions. Regions with a reduced end-diastolic wall thickness virtually never exhibited contractile reserve during dobutamine stress echocardiography. In regions with a wall thickness equal to 6 mm, the response to dobutamine varied; approximately 60% of these segments were viable on dobutamine stress echocardiography, and the remaining 40% were nonviable. Conceivably, the regions with an end-diastolic wall thickness less than 6 mm consist of scarred and fibrotic tissue and do not improve in function following revascularization. Assessment of LV end-diastolic wall thickness can be used as an initial evaluation for myocardial viability: Dysfunctional segments with an end-diastolic thickness less than 6 mm are nonviable and may not need further evaluation; segments with a preserved end-diastolic wall thickness (≥6 mm) can be further classified as viable and nonviable myocardium based on the presence/absence of contractile reserve during dobutamine challenge.
Dobutamine Stress Echocardiography
Methodology. Extensive clinical experience has been obtained with dobutamine stress echocardiography to assess myocardial viability.2,3 Dobutamine is a β1-specific agonist that increases myocardial oxygen demand by increasing heart rate, contractility, and arterial blood pressure. The safety of dobutamine stress echocardiography was evaluated by Secknus et al.,10 reporting an incidence of 7.6% side effects in 3011 patients undergoing dobutamine stress echocardiography. β-blockers can be used as antidotes. Infusion of low-dose dobutamine (5 to 10 mcg/kg/min) has been demonstrated to increase contractility (without a substantial increase in heart rate) in dysfunctional but viable myocardium, which has been referred to as contractile reserve. Segments without viable myocardium do not show contractile reserve. The protocol can be extended with high-dose dobutamine infusion (with infusions up to 40 mcg/kg/min with addition of atropine if needed), which allows assessment of myocardial ischemia.12 With the low-high dobutamine dose stress protocol, four response patterns can be observed:
Pattern 1 represents transmural scar, whereas pattern 2 represents severe ischemia in a region subtended by a critically stenosed artery. Pattern 3, on the other hand, is probably related to subendocardial scar tissue, whereas pattern 4 represents viability with superimposed ischemia. An example of a patient with myocardial ischemia during dobutamine stress echocardiography is shown in Figure 39-1.
Accuracy. Stress echocardiography has been evaluated extensively for the detection of viable myocardium in patients with chronic ischemic LV dysfunction. Forty-one studies with dobutamine stress echocardiography have reported on the sensitivity and specificity for detection of myocardial viability and prediction of recovery of regional function following revascularization.11–51 A total of 1849 patients were included in these 41 studies. The weighted mean sensitivity and specificity respectively were 80% and 78%, with a positive and negative predictive value of 75% and 83% (Fig. 39-2; Table 39-3).
Table 39-3 Pooled Data from Viability Studies Using Different Techniques to Predict Improvement of Regional Contractility After Revascularization*
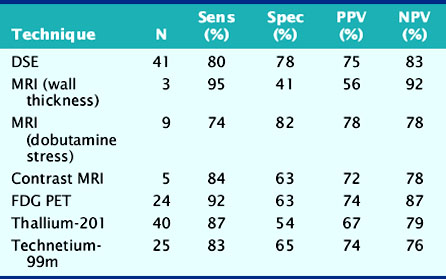
For the prediction of recovery of global LV function, 6 studies including 287 patients are available, using dobutamine stress echocardiography.45–47,50,52,53 Pooled analysis of these six studies revealed a weighted mean sensitivity and specificity of 57% and 73%, respectively with a positive and negative predictive value of 63% and 68% (Fig. 39-3; Table 39-4).
Table 39-4 Pooled Data from Viability Studies Using Different Techniques Predicting Improvement of Global Left Ventricular Function After Revascularization*
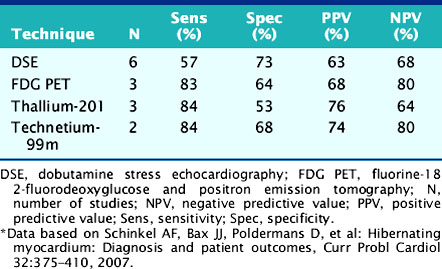
The prognostic value of viability assessment using dobutamine stress echocardiography was addressed in 11 studies that included 1753 patients (5 studies used high-dose dobutamine, 5 low-dose dobutamine, 1 low-high dose dobutamine).54–63 The event rates in relation to viability and therapy are presented in four groups:
Patients with viable myocardium undergoing revascularization had the best survival, while the highest annualized mortality rates were noted in patients receiving medical therapy, either with or without viable myocardium. An intermediate mortality rate was observed in patients without viable myocardium who underwent revascularization (Fig. 39-4).
Novel Technical Developments in Echocardiography and Viability Assessment
Echocardiography is a widespread imaging modality that has a number of advantages, including bedside availability, real-time imaging, low costs, and absence of radiation. Besides information on systolic and diastolic LV function, information on LV volumes, pericardial pathology, and valvular function can be obtained. However, especially in obese patients and those with chronic obstructive pulmonary disease, the technique may be limited by a poor acoustic window, making precise endocardial border delineation and assessment of regional wall motion difficult. Second harmonic imaging has significantly improved endocardial border delineation.64 Intravenous administration of contrast agents can further improve visualization of the endocardium (Figs. 39-5, 39-6 and 39-7).65 Reilly et al.66 determined the value of contrast echocardiography for assessment of LV wall motion in 70 patients in the intensive care unit, who had a poor acoustic window. Wall motion was evaluated on standard echocardiography, harmonic echocardiography, and after intravenous contrast administration. Uninterpretable wall motion was present in 5.4 segments per patient on standard echocardiography, 4.4 on harmonic echocardiography (P = 0.2), and 1.1 on contrast echocardiography (P < 0.0001). An average of 7.8 segments were read with surety on standard echocardiography, 9.2 on harmonic echocardiography (P = 0.1), and 13.7 on contrast echocardiography (P < 0.0001). The safety of the use of intravenous contrast has recently been questioned, but various studies have clearly demonstrated the safety, both in patients with stable CAD and patients with recent myocardial infarction.67,68 Aggeli et al.67 evaluated 5250 individuals with dobutamine stress echocardiography and intravenous contrast and reported very low adverse event rate, without deaths or infarction and serious cardiac arrhythmias in 0.04%. Therefore, particularly in patients with a poor acoustic window, contrast echocardiography appears to have incremental value for assessment of LV function and wall motion.
In addition, the combination of intravenous contrast and three-dimensional (3D) echocardiography may even further increase accuracy. This technique has resulted in superior visualization of the endocardial walls and excellent assessment of LV volumes and LVEF (see Figs. 39-6 and 39-7). Traditionally, analysis of the dobutamine stress echo data was performed visually, whereas nuclear imaging permitted quantitative (objective) analysis. Tissue Doppler imaging, however, allows quantification of systolic (and diastolic) velocities of the myocardium (Fig. 39-8).69 Rambaldi and colleagues70 have evaluated 40 patients with chronic CAD and LV dysfunction (mean ejection fraction 33% ± 11%); all underwent metabolic imaging with fluorine-18-fluorodeoxyglucose (FDG) SPECT (as the gold standard for viability) and dobutamine stress echocardiography. The authors used tissue Doppler imaging to quantify the peak systolic velocity of dysfunctional segments, both at rest and during dobutamine infusion. The peak systolic velocity at rest was not different between viable and nonviable segments (according to findings on metabolic imaging), but the velocities during low-dose dobutamine (8.6 ± 2.9 cm/sec versus 6.0 ± 1.8 cm/sec, P < 0.05) and high-dose dobutamine (9.3 ± 3.1 cm/sec versus 6.2 ± 2.1 cm/sec, P < 0.05) were significantly higher in viable segments compared to nonviable segments.
A further improvement in quantification of data is the use of strain. This approach has the advantage over velocity imaging in that it can differentiate between passive motion and active deformation. In theory, strain imaging may therefore provide perfect information on viability and may prevent the well-known problem of tethering in stress echocardiography. Recently, speckle-tracking strain imaging was introduced as a novel echocardiographic technique that enables the assessment of multidirectional myocardial strain. Based on the detection of natural acoustic markers (so-called speckles) equally distributed in the myocardial wall, speckle-tracking imaging quantifies myocardial deformation in the radial, circumferential, and longitudinal directions, with independence of the angle insonation of the ultrasound beam (which is not the case with Doppler-derived strain imaging).69 An example of these different strains in a normal individual is shown in Figure 39-9. Even the resting strain of dysfunctional myocardium is already an indicator for viability. Becker and coworkers71 evaluated 47 patients with chronic ischemic LV dysfunction and reported that radial strain and strain rate decreased in parallel to an increasing extent of scar tissue as assessed with contrast-enhanced MRI. Radial strain allowed differentiation between nontransmural and transmural infarction with a sensitivity of 70.0% and a specificity of 71.2%, when a cutoff value for radial strain of 16.5% was used. In a subsequent study, 53 patients underwent two-dimensional (2D) radial strain imaging to assess viability; these patients underwent revascularization with assessment of functional recovery at 9 ± 2 months of follow-up.72 Segments with recovery of function had more preserved radial strain (22.6% ± 6.3% versus 15.2% ± 7.5%, P < 0.001); using a cutoff of 17.2% for peak systolic radial strain, functional recovery could be predicted with a sensitivity of 70.2% and a specificity of 85.1%). The quantification of strain during low-dose dobutamine stress to assess viability has also been explored. In 55 patients undergoing revascularization, the quantification of strain during low-dose dobutamine stress echocardiography yielded a sensitivity ranging from 74% to 80%, with a specificity around 77%, to predict improvement of function after revascularization.73 Finally, postsystolic thickening can be derived from tissue Doppler imaging and has been proposed as a marker for viability.74 Some groups argued that postsystolic thickening is an accurate marker of viability and demonstrated good agreement with viability on metabolic imaging with FDG,75 whereas others suggested that postsystolic shortening represents scar tissue. Lim et al.76 evaluated 25 patients with chronic LV dysfunction (mean ejection fraction 32% ± 10%) with tissue Doppler imaging (to assess postsystolic thickening) and contrast-enhanced MRI. The authors demonstrated that postsystolic thickening was most often observed in segments with transmural scar formation on MRI.
Echocardiography with Intravenous Contrast
Methodology. The improved technical properties of the myocardial contrast agents now allow for assessment of myocardial perfusion (Fig. 39-10). In early studies, intracoronary injection of contrast was still needed, but with the newer generation of contrast agents, intravenous administration is possible. The recent contrast agents are composed of high-molecular-weight inert gases. The microbubbles stay in the vascular space and do not enter the extravascular space. Within the vascular space, microbubbles behave like red cells in terms of rheology and can be used in combination with echocardiography to directly visualize the myocardial perfusion. Since myocardial perfusion is a prerequisite for myocardial viability, myocardial contrast echocardiography has been used to assess myocardial viability. It has been shown that echo contrast parameters of myocardial perfusion correlate positively with the microvascular density and the capillary area and inversely with the extent of fibrosis.77 In the clinical setting, myocardial perfusion by myocardial contrast echocardiography is evaluated qualitatively, and segments are visually classified as being viable with normal or patchy perfusion, and nonviable when perfusion is absent (see Fig. 39-10).
