Mucociliary Clearance
Mucus forms an essential barrier that protects the lungs from inhaled particles, pathogens, and toxicants. However, excessive mucus accumulation contributes to the pathogenesis of all the common diseases of the airways. Therefore, understanding airway mucus function and dysfunction is important for pulmonary medicine. The airway mucus barrier is mobile, continually propelled in a proximal direction by ciliary beating. Ciliary dysfunction causes disease both because of the failure to clear xenobiotics from the lungs and because it results in mucus accumulation. Mucus and ciliary biology will be considered together in this chapter as they interact to achieve, or fail to achieve, airway clearance.
MUCOCILIARY CLEARANCE IN HEALTH
AIRWAY SURFACE LIQUID
Liquid in the airway lumen is distributed between two distinct layers—a mobile mucus layer and a stationary periciliary layer (Fig. 6-1). Secreted polymeric mucins are the principal macromolecular components of the mucus layer, whereas membrane-tethered mucins and nonmucin glycoconjugates are the principal macromolecular components of the periciliary layer.
Figure 6-1 Airway surface liquid layers. A. Mucus is continuously produced in the conducting airways of the lungs, and swept by ciliary action from distal to proximal airways. After passing through the larynx, mucus is swallowed. B. The mobile mucus layer (light blue) glides over a periciliary layer of higher osmotic modulus (dark blue). C. Airway secretory cells synthesize and secrete mucin polymers that interact with water to form the mobile mucus layer. Ciliated cells are covered by a dense glycocalyx containing glycosaminoglycans, membrane-tethered mucins, and other glycoconjugates that give the periciliary layer its high osmotic modulus. D. Mucin polymers are illustrated in the mucus layer with the protein core shown in black, sugar side chains in blue, and sites of end-to-end polymerization as black circles. Membrane-tethered mucins are shown densely coating cilia, while other glycoconjugates are not illustrated. (Reproduced with permission from Dickey BF: Biochemistry. Walking on solid ground, Science. 2012;337(6097):924–925.)
Mucus Layer
Normal mucus has physical characteristics on the border between a viscous fluid and a soft and elastic solid. Its physical state can vary with the extent of hydration and other conditions as described below in Mucociliary Dysfunction in Disease. Mucus is formed by a network of mucin polymers in water, with water accounting for ~98% of the mass, mucins 0.7%, and salts and nonmucin macromolecules the remainder. Mucins are exceedingly large glycoproteins (monomeric masses up to 3 × 106 Da) that link up to form long chains and branched networks. MUC5AC and MUC5B are the major secreted mucins in the airways.1–3 They exhibit similar molecular weights and primary structure (Fig. 6-2A), but differ in function (see Secretory Cells) and polymer structure. Whereas MUC5B forms end-to-end polymers (Fig. 6-2B), emerging data suggest that MUC5AC forms branched covalent networks. They have characteristic regions rich in serine and threonine residues linked by their hydroxyl side groups to sugar chains (O-glycosylation) that account for 50% to 90% of the mass of mucins.
Figure 6-2 The structure of MUC5B. A. Structural domain representation of the MUC5B monomer. MUC5B has Von Willebrand factor (VWF)-like domains at NH2– and COOH-terminal regions (D, B, C, and CK domains). The central region contains five heavily glycosylated, tandem repeat (TR), mucin domains and five small cysteine-rich (cys) regions. B. Electron microscopy image of a conformationally relaxed, linear MUC5B molecule. The MUC5B intact molecule in mucus is assembled from disulfide bond-mediated interactions between COOH-terminal domains of monomers to form dimers, and subsequent interactions between NH2-terminal domains to form higher polymer oligomers. The assignment of the structure as a dimer (dotted bar) is made on the basis of the length (850 nm), which is greater than that obtainable by a single MUC5B monomer. The N↔N terminal region and C↔C terminal region can be identified by their size. Scale bar, 100 nm. (Reproduced with permission from Kesimer M1, Makhov AM, Griffith JD, et al. Unpacking a gel-forming mucin: a view of MUC5B organization after granular release. Am J Physiol Lung Cell Mol Physiol. 2010;298(1):L15-L22.)
Mucins are packaged dehydrated in secretory granules. After secretion, they rapidly adsorb several hundred fold their mass of water, so it is critical there be sufficient airway surface liquid.4 Insufficient liquid results in formation of a gel that is too viscoelastic to be readily cleared by ciliary motion or cough. Once immobile mucus plaques are formed, they swell only very slowly if subsequently exposed to additional liquid because of the high degree of entanglement of the mucin polymers. Besides the need for sufficient surface liquid, adequate bicarbonate must be present in the liquid to allow proper mucus maturation by chelating calcium. In secretory granules, calcium organizes the folding of mucin polymers, and must dissociate to allow mucus expansion.5–7 Chloride and bicarbonate are both secreted into the airway lumen by the cystic fibrosis transmembrane regulator (CFTR). Since the airway epithelium is quite water-permeable, water follows chloride and bicarbonate into the airway lumen.8 Additional hydration can be provided by other chloride channels as described below in “Mucociliary Dysfunction Disease the sections “Secretory Cells” and “Asthma.”
Mucus is produced throughout the conducting airways down to the level of the smallest bronchi and the larger bronchioles, but not in terminal or respiratory bronchioles (Fig. 6-3A). Bronchioles lack submucosal glands, and overall it is estimated that two-thirds of airway mucus is produced by surface epithelial cells and one-third by submucosal glands in primates (Fig. 6-3B).9 In the most distal airways, the mucus layer is vanishingly thin, but it becomes increasingly thick as it travels proximally, both because additional production adds to its bulk and because the total cross-sectional area of the airways progressively narrows. In the trachea, the mucus gel layer is ~50 μm thick. Besides mucus acting as a simple physical barrier, the mucin sugar side chains act as a combinatorial library to bind particles and pathogens, and a thicker mucus layer has been shown to protect against infection.10
Figure 6-3 Mucin production in conducting airways. A. MUC5B is produced constitutively by surface epithelium down to the level of bronchioles proximal to terminal bronchioles, and by submucosal glands present in the trachea and bronchi but not bronchioles. MUC5AC is produced constitutively by surface epithelial cells with a goblet morphology in more proximal airways, and its production can be induced in nongoblet secretory cells that produce MUC5B down to the level of terminal bronchioles. Airway generation is listed numerically in the third column. B. The relative amount of mucin produced by surface epithelium and submucosal glands has been estimated by morphometric analysis of the airways of rhesus monkeys stained with Alcian blue/PAS, expressed as the ratio of volume of stained material (mm3 × 10–3) per unit area of basal lamina (mm2). (A: Modified with permission from Weibel ER: Morphometry of the Human Lung. Heidelberg: Springer-Verlag; 1963; B: Reproduced with permission from Plopper CG1, Heidsiek JG, Weir AJ, et al. Tracheobronchial epithelium in the adult rhesus monkey: a quantitative histochemical and ultrastructural study, Am J Anat. 1989;184(1):31–40.)
Periciliary Layer
It was widely believed until recently that cilia beat in a watery layer of lower density than the overlying mucus layer. However, recent data indicate that the periciliary layer has a higher density than the mucus layer due to a high concentration of membrane-tethered mucins (MUC 1, 4, and 16) and mucopolysaccharides (also called glycosaminoglycans).11–13 This finding has several important implications for airway function.14 First, it helps to explain how distinct layers form because dense packing of the grafted glycoconjugates of the periciliary layer tends to exclude the unattached polymeric mucins of the mucus layer (Fig. 6-1C). Second, charged polymers are highly effective lubricants in an aqueous environment, allowing low friction ciliary beating despite the high density of grafted glycoconjugates, and low friction between the periciliary and gel layers. Third, spatial impingement by grafted glycoconjugates may physically couple neighboring cilia to coordinate their beating (Fig. 6-1C). Fourth, glycoconjugates are grafted with increasing density from the top of the periciliary layer to the bottom, which should propel exogenous particles and pathogens out of the periciliary layer for removal by the mobile mucus layer. Fifth, the higher density of water-avid glycoconjugates in the periciliary layer and their grafting to the cell surface results in a nearly constant amount of liquid in this layer except under conditions of severe underhydration. In conditions of overhydration, liquid is transferred to the mucus layer, which is generally well tolerated.
AIRWAY EPITHELIAL CELLS
The surface airway epithelium forms a mosaic of two major cell types—secretory and ciliated (Figs. 6-1, 6-4, and 6-5). In addition, basal cells that do not contact the airway lumen serve as progenitors in the proximal airways, and neuroendocrine cells that secrete basolaterally toward sensory neurons are scattered throughout the airways. Secretory and ciliated cells are multilayered and have a tall columnar shape in proximal airways but become single layered and progressively shorter in distal airways until they have a cuboidal shape in bronchioles. Ciliated cells are more abundant than secretory cells in proximal airways, whereas secretory cells are more abundant in distal airways.
Figure 6-4 Cilia structure. A. Scanning electron micrograph of mouse tracheal epithelium showing both ciliated and nonciliated cells. Note the difference in size between the microvilli (on nonciliated cell surfaces) and the cilia. Scale bar, 5 μm. B. Transmission electron micrograph of an airway cilium in cross section showing the 9+2 configuration of the microtubules. Arrows point to the central pair of microtubules, and to the outer dynein arms (ODA) and inner dynein arms (IDA) that are critical for ciliary movement and common sites of mutation in PCD. (A: Photograph used with permission of Charles Daghlian, Dartmouth Electron Microscope Facility. Released to the public domain, via Wikimedia Commons.)
Figure 6-5 Airway epithelial mucus metaplasia. Light micrographs with AB-PAS staining (A, B), electron micrographs (EM) (C–F; low and high magnification), and immunohistochemical images using mucin-specific antibodies (G–J). On the left are images from the airway of a healthy mouse without airway inflammation (naïve), and on the right are images from the airway of a mouse with mucus metaplasia 3 days after the onset of allergic inflammation, as described by Evans et al.15 Cells in the top row on the left show alternating ciliated (arrow) and domed secretory (arrowhead) cells, and on the right show prominent mucin granules in secretory cells. Cells in the EM images on the left show small numbers of electron-dense secretory granules (SG) near the apical membrane, numerous mitochondria (Mito), and abundant smooth endoplasmic reticulum (sER). Cells in the EM images on the right show numerous electron-lucent SG containing mucins and an electron-dense core, and abundant rough ER (rER). Images in the bottom row show that Muc5b is present in naïve airways even though it is not apparent by insensitive AB-PAS staining, and that both Muc5ac and Muc5b are present in the thickened metaplastic epithelium. Scale bar in the right middle panel is 10 μm for top row, 1 μm for second row, 150 nm for third row, and 30 μm for bottom row.
Secretory Cells
Subsets of airway secretory cells have been given different names based upon differences in their microscopic appearances, including goblet and club (Clara) cells. However, molecular techniques reveal overlap between these subsets, such as the presence of CCSP (SCGB1A1) in cells with a goblet morphology that express mucin, and the presence of MUC5B in cells with a club morphology.15–17 Secretory cells are unified by the stable expression of an apical-regulated exocytic machinery,18,19 whereas the presence of secretory products such as mucins and secretoglobins varies with exposure to extracellular signals that acutely regulate their expression and secretion (Fig. 6-5). Therefore, it is simplest to refer to these cells generically as airway secretory cells, while further specifying their appearance, airway level, and gene expression as appropriate.
Secretory cells in bronchi and proximal bronchioles constitutively express MUC5B (Figs. 6-3 and 6-5), whereas secretory cells in terminal and distal bronchioles do not express MUC5B either constitutively or inducibly.1,15,20 The same bronchial and proximal bronchiolar secretory cells that express MUC5B can also express MUC5AC, with MUC5AC expressed constitutively in proximal airways but only inducibly in distal airways (Figs. 6-3 and 6-5). Mice with deletion of Muc5b die postnatally from upper and lower respiratory tract inflammation and infection.21 In contrast, mice with deletion of Muc5ac are healthy at baseline but fail to clear parasitic worms from their guts,22 and induced expression of Muc5ac in rat lungs prevents worms from transiting the lungs.23 Thus, Muc5b/MUC5B appears to be the principal mucin functioning in baseline clearance of the airways, while Muc5ac/MUC5AC appears to function principally in parasite defense.
The effector function of Muc5ac in parasite defense dovetails well with the central role of IL-13 in parasite defense and the ability of IL-13 to strongly induce expression of Muc5ac/MUC5AC (>100-fold in cultured human airway cells and 40-fold in mice in vivo).24–26 Other cytokines that promote mucin gene expression include IL-1β, 4, 6, 9, 23, and 25, but whether they do this directly or by amplifying the intensity of IL-13 signaling is not yet clear.1 Complement protein C3a, epinephrine, and γ-aminobutyric acid signaling interact with IL-13 signaling to augment Muc5ac expression.1 ClCa1 is a secreted protein that signals in an autocrine and paracrine fashion in response to IL-13 to increase Muc5ac/MUC5AC expression.24 A network of transcription factors has been identified that regulates Muc5ac/MUC5AC expression, including STAT6, SPDEF, Foxa2, Foxa3, Notch, β-catenin and XBP-1. While some components of the signaling pathways connecting extracellular ligands with transcriptional regulation are known, such as the key roles of STAT6 downstream of IL-13 and MAPK13 downstream of ClCa1,24,27 many details remain to be elucidated. The pathways and transcription factors regulating Muc5b/MUC5B expression have been less studied, probably reflecting the fact that expression of this mucin is relatively stable compared to Muc5ac/MUC5AC.28 However knowledge of the regulation of MUC5B expression during development is likely to give important insight into cell fate specification in the airway, and the recent discovery of aberrant MUC5B expression in interstitial lung diseases highlights its clinical importance (see the section “Interstitial Lung Diseases”). Along with production of the polymeric mucins themselves, specialized enzymes required for glycosylation (e.g., GalNAc-T),29 folding and polymerization (e.g., AGR2),30 and other aspects of mucin processing are produced by airway secretory cells.
Mucins are secreted into the airway lumen at a low basal rate and a high stimulated rate. A regulated exocytic mechanism mediates both rates as indicated by abnormal phenotypes in both basal and stimulated secretion when Munc13-2, a sensor of second messengers, is deleted in mice.16 Additional molecular components of the exocytic mechanism have been identified and their function studied (Fig. 6-6).18,19,31,32 The rate of mucin secretion is regulated by the second messengers calcium and diacylglycerol, which are generated by a signaling cascade downstream of G-protein–coupled receptors that include the P2Y2 purinergic and A3 adenosine receptors.18,33,34 ATP is released in autocrine and paracrine fashion and activates P2Y2 receptors and is metabolized to adenosine. It is possible that additional ligands such as histamine and acetylcholine may serve as secretagogues in inflammation, though they may act indirectly by causing smooth muscle contraction and nucleotide release.
Figure 6-6 Airway mucin secretion. Initially, mucin granules become tethered to the plasma membrane by Rab proteins and their effectors in the vicinity of the exocytic SNARE proteins (VAMP8, SNAP23, and an unknown Syntaxin, shown as black bars) (Left). Activation of heptahelical receptors such as those for ATP (P2Y2) and adenosine (A3R) leads to activation of the trimeric G-protein, Gq, and phospholipase C (PLC), resulting in generation of the second messengers diacylglycerol (DAG) and inositol trisphosphate (IP3). Diacylglycerol activates the priming protein Munc13-2, and IP3 induces the release of calcium from apical ER to activate Synaptotagmin-2 (Syt2) (Center). Activation of the regulatory Munc13 and Syt proteins leads to full coiling of the SNARE proteins to induce fusion of the granule and plasma membranes. The interactions of the SNARE proteins take place on a scaffold provided by Munc18b (Right). (Adapted with permission from Davis CW1, Dickey BF: Regulated airway goblet cell mucin secretion, Annu Rev Physiol. 2008;70:487–512.)
As secreted mucins must adsorb large volumes of water to yield mucus of normal viscoelasticity, it is important that adequate airway lumenal water be available. The autocrine/paracrine ligands ATP, adenosine, and ClCa1 regulate the expression and/or activity of CFTR, the calcium-activated chloride channel (CaCC) TMEM16 A, and solute carrier family 26, member 9 (SLC26A9) to control chloride secretion.24,33,35 As these same ligands regulate mucin production and/or secretion as described earlier, mucin release is thus coordinately regulated with water translocation.
Besides their role in mucin production and secretion, airway secretory cells serve as progenitors of both secretory and ciliated cells.36 They also express components of the cytochrome P450 system that inactivates toxic inhaled organic compounds by oxidation.37 They secrete antimicrobial peptides and reactive oxygen species constitutively, and they are capable of sensing pathogens and responding by augmenting their antimicrobial defenses and signaling to leukocytes.38
Ciliated Cells
Motile cilia are the defining structural and functional feature of airway ciliated cells (Fig. 6-4) (Video 6-1 Normal). They are evolutionary conserved and share homologous features with flagella of unicellular eukaryotes and mammalian sperm tails. Respiratory cilia have the “9+2” microtubular axonemal structure, which comprises nine peripheral doublets plus a central pair.
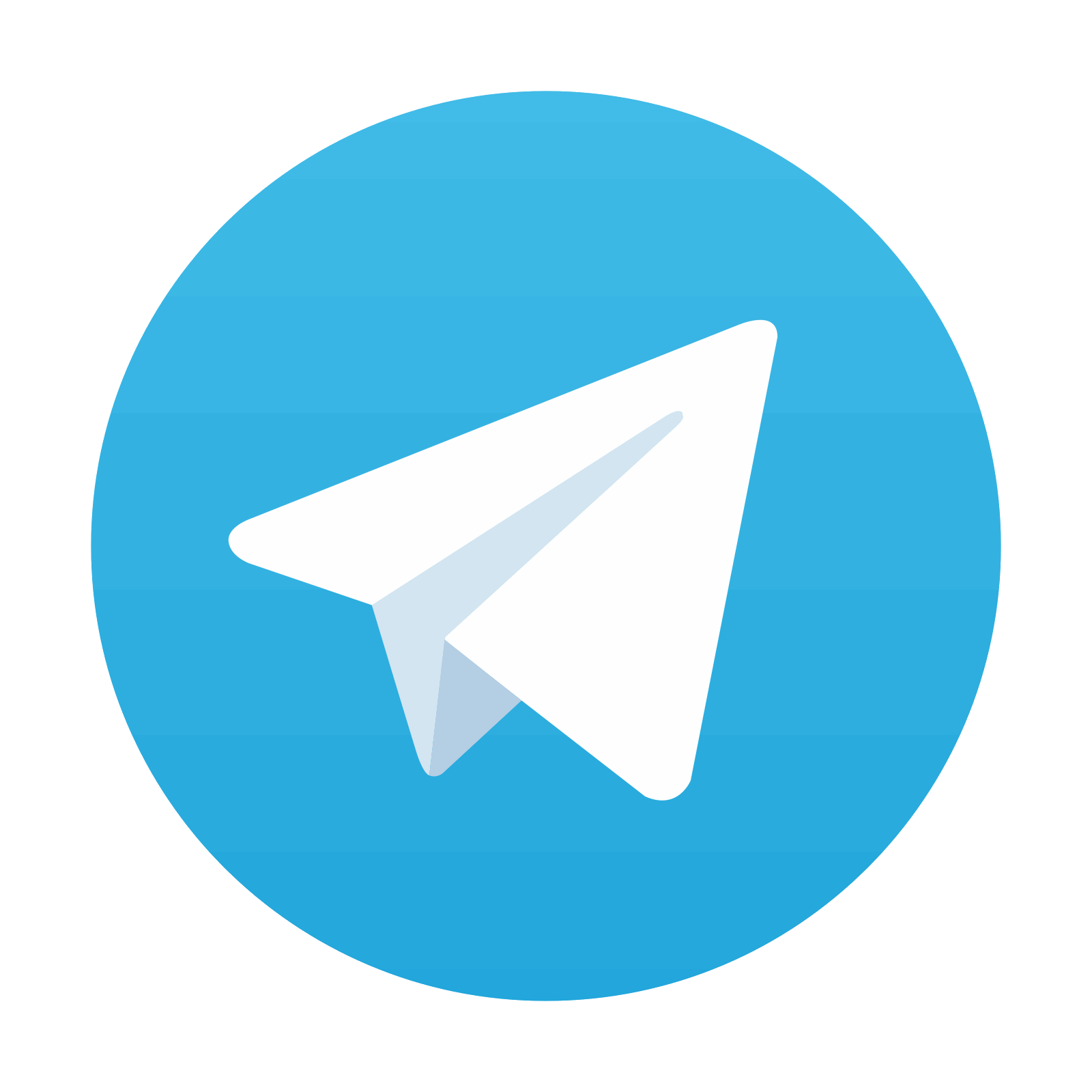
Stay updated, free articles. Join our Telegram channel

Full access? Get Clinical Tree
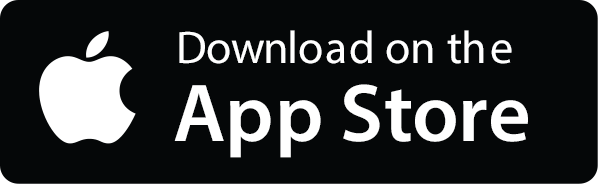
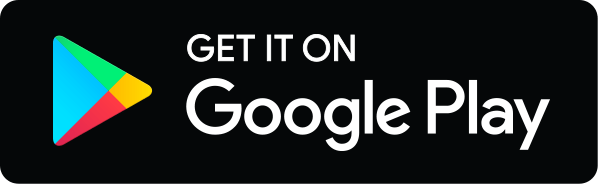