Microbial Virulence Factors in Pulmonary Infections
OVERVIEW: MICROBIAL PATHOGENESIS
Beginning at birth, microbial organisms enter and leave the body, primarily on external or mucosal surfaces. Some of these predominantly commensal organisms become resident; others are transient; and still others establish latent foci in otherwise sterile spaces. Over a lifetime, a person is the reservoir for hundreds of strains of viruses, thousands of bacterial species, and a scattering of fungi and parasites. When these organisms violate their niche, invade, or produce toxic products, and at the same time overcome innate and adaptive host defenses, virulent interactions take place and occasionally lead to disease. Organisms can cause disease without entering or adhering to tissues by releasing toxic products. However, all infections caused by obligate intracellular parasites such as viruses require attachment of microbes onto cells and subsequent intracellular invasion as typified by the viral hemagglutinin molecule on the surface of the influenza virus, which determines species and tissue tropism, transmissibility, and replication.1,2
Binding to cells does not always occur with bacterial pathogens, particularly in the lung. In contrast to the GI tract where most bacterial pathogens must attach to surfaces and some, such as Shigella dysenteriae, do enter into cells, many studies with common respiratory bacterial pathogens such as Pseudomonas aeruginosa, Klebsiella pneumoniae, Streptococcus pyogenes, and others have found that avoidance of binding to respiratory epithelial cells promotes progression of infection and development of disease. Thus, the statement that one often encounters that bacterial binding to epithelial cells initiates infection in the respiratory tract is not correct for some prominent lung pathogens. This is likely due, in large part, to the role of bacterial cell binding to the respiratory epithelium in promoting clearance of most organisms entering the lung (see Chapter 121). This occurs by a variety of nonspecific innate immune mechanisms that are potently activated by microbial binding to epithelial surface or, following binding and internalization, binding to intracellular toll-like receptors (TLRs) and other conserved pattern-recognition molecules. Along with induction of cytokine synthesis and release there is a rapid activation of beneficial inflammation and clearance. In some situations, however, organisms are able to avoid such clearance and propagate and produce clinical symptoms. For example, in neutropenic mice, the infectious dose of P. aeruginosa applied to the nose of an anesthetized animal that produces a lethal pneumonia and sepsis can be in the range of 10 to 50 bacterial cells, whereas in a neutrophil-sufficient setting usually 107 to 108 bacterial cells are needed to initiate infection. Clearly, avoiding polymorphonuclear leukocyte (PMN)-mediated clearance initiated by innate immunity is essential for serious disease due to P. aeruginosa. This finding highlights how pathologic conditions in the host can predispose to entry and survival of microbes, ranging from breaks in mucosal surfaces to defects in the immune system (see Chapter 121). Organisms become parasites when they express the requisite virulence determinants to gain entry and overcome or evade host defenses.
An important step in establishing infection occurs when the potential pathogen encounters the immune system. There are numerous mechanisms whereby the immune system detects and tries to limit the extent of microbial challenge, including inflammation (acute and chronic), phagocytosis (neutrophils and macrophages), complement activation, and humoral and cellular immune responses. The immune system also maintains surveillance for organisms that invade phagocytes, propagate, and resist killing, and also for organisms that invade nonphagocytic cells. Means by which microbes can be controlled range from physical clearance mechanisms to phagocytosis (followed by oxidative or nonoxidative killing) to nutritional depletion (e.g., sequestration of iron, which is an essential nutrient for bacterial growth).
Microbes have evolved a variety of strategies to overcome host defenses, evade the immune system, scavenge for nutrients, eradicate competing bacteria, and survive to spread to other hosts. These processes can lead to tissue damage and even death of the host. However, ultimate survival of the microbe requires eventual spread to a new host. A new “generation” of microbes is established (by clonal division) approximately once an hour, whereas a new generation of humans occurs about once every 20 years. Thus, the microbes have a clear genetic advantage in selecting properties that enhance virulence and survival. In response, the human immune system has developed a variety of defenses against a broad range of pathogenic mechanisms. Infections can occur at specific sites on surfaces or within the body or involve local, distal (metastatic), or systemic spread. Infection can occur without damaging cells, through direct cellular damage by microorganisms or their toxins, or as a consequence of the immune response. When physiologic disruption or cellular damage occurs, the host needs to recover and repair the damage. In addition, the immune system attempts to recognize the pathogen and develop an effective adaptive immune response involving T cells and antibody to prevent reinfection. Pathogens have also evolved a series of mechanisms to avoid immune detection, including local interference with immune processes, antigenic variation, avoidance of inducing immune responses, or inducing ineffective immune responses.
Organisms are constantly evolving to meet the demands and opportunities of modern society. Just as the cities of the Middle Ages brought together humans and rats and caused outbreaks of bubonic plague, the use of antibiotics, chemotherapy, and various medical devices has led to a number of new pathogenic interactions between microbes and humans. A key to understanding the pathophysiology of infectious diseases and appreciating the complexity of both the immune system and the microbial world is knowledge of the facts and processes of each; this knowledge base is necessary for an appreciation of the ideas presented above and the conceptual basis of immunity and infection as related to respiratory tract infections.
GENERAL MECHANISMS OF INFECTIOUS PROCESSES IN THE RESPIRATORY TRACT
The pathogenesis of acute and chronic microbial infections of the lung entails complex interactions between the microorganisms and a variety of host defense mechanisms. The general steps and molecular factors involved in the pathogenesis of microbes causing lung infections are summarized in Table 124-1. Although the alveolar spaces are generally sterile, low levels of microorganisms are continually inhaled into the lungs. Inoculation of the lungs can occur from a variety of sources, including aspirated oropharyngeal secretions or bacteria in small aqueous droplets inhaled directly via the nose or mouth. Most commonly, inhaled organisms, either alone or in association with particles of mucus, gain access to the lower airways. They are generally either cleared by mucociliary flow or scavenged by phagocytes (see Chapter 121). Particulates greater than 10 μm in diameter are deposited in the larger airways. Particles of less than 5 μm that are not cleared in the larger airways can be deposited in the alveoli. Most particulate matter is not infectious, and only spores or organisms that remain viable can cause infection. Thus, the pathogenesis of microbial infection will initially depend on a microbe’s ability to enter the respiratory system and avoid clearance by mechanical and innate immune mechanisms.
As noted earlier, microorganisms can reach the lower airways from various sources. Organisms in ambient air can be inhaled as droplet nuclei—particularly in closed environments, where density is great and infected individuals can deposit organisms into the air. Perhaps the most important source of organisms causing pneumonia is the flora of the upper respiratory tract. A large ecosystem of microorganisms that includes both pathogenic and nonpathogenic bacteria and fungi normally resides in the upper airways (see Chapters 127 and 133). The quantity and species diversity of many of these organisms can be stable over long periods, but transient colonization with a variety of microbes also occurs with some frequency, and these changes are often correlated seasonally and/or with concomitant infections with respiratory viruses. For example, Streptococcus pneumoniae (pneumococcus) and Neisseria meningitidis are more frequently isolated from the nasopharynx or throat during the winter months. Bacteria colonizing the nasopharynx of healthy children are often associated with the concurrent presence of certain viruses and bacteria, for example, Staphylococcus aureus with influenza but not rhinoviruses or pneumococcus; pneumococcus with rhinoviruses, Haemophilus influenzae, and Moraxella catarrhalis.3 In addition, organisms that make up the normal microbial flora in other parts of the body can be transferred to the lung, where they can cause infection. In early-onset group B streptococcal pneumonia and sepsis in neonates, the organisms are transferred during birth from the vaginal canal of the mother to the respiratory tract of the infant.
Organisms causing infections at other body sites can spread to the lungs. Although not common, hematogenous spread from the bloodstream to the lungs can occur, perhaps most notably in Lemierre disease related to septic thrombophlebitis due to the anaerobe Fusobacterium necrophorum. The existence of heavy colonization or infection in the upper airways also increases the potential for infection in the lungs by a variety of mechanisms, mostly by a simple dose effect, whereby a large burden of potentially pathogenic organisms overwhelms the clearance mechanism of the lungs. Another mechanism for pneumonic infection can result from conditions that perturb the specific and nonspecific defenses, leading to respiratory infection as a sequela of another pathogenic process. A common example of this process is bacterial infections secondary to influenza virus. The neuraminidase of influenza virus has been shown to play a synergistic role in pneumococcal pneumonia models by cleaving the sialic acid residues on host glycoconjugates, thereby leading to increased adherence of the bacterium.4 Activation of the innate immune response to viral RNA, modeled by polyI:C-mediated stimulation of the TLR3 and RIG-I pathways, which induce Type I interferons, can also increase susceptibility of mice to pneumococcal and S. aureus pneumonia.5 During the adaptive immune response to influenza in mice, interferon-gamma can interfere with alveolar macrophage phagocytic function and heighten susceptibility to S. pneumoniae pneumonia.6 Finally, any process that disrupts the physiologic and physical barriers between the upper and lower airways can lead to infection—for example, the placement of an endotracheal tube or changes in normal clearance mechanisms associated with cystic fibrosis.
Once a microorganism gains access to the lower respiratory tract, it must be able to resist clearance from this space, remain viable, and multiply. Usually organisms will either multiply locally, resisting local defenses, or spread to other body sites by traversing epithelial barriers that normally inhibit microbial spread. In order for extracellular organisms to multiply, they must scavenge for nutrients. Of particular note is the universal requirement for iron, which must be extracted from iron-binding molecules such as transferrin. Many bacteria produce iron-binding substances known as siderophores that have an affinity for iron of greater than 1018 M and bind to high-affinity receptors on the bacterial surface. Organisms must also resist opsonophagocytosis or be able to survive and multiply within phagocytes. Subsequent to microbial growth and resistance of host defenses, damage to the host tissues occurs. This process is aided by a variety of pathogenic factors and results in invasion of tissues often with destruction of cells. Secreted toxins can act locally and/or systemically spread to cause clinical symptoms. Many Gram-negative bacilli possess a type III secretion system that can function like a hypodermic needle to inject toxins directly into host cells. The potential for inflammation to cause tissue destruction can be a devastating consequence of microbial growth in normally sterile lung sites. Finally, although not necessary for the pathologic process to take place, most organisms that successfully multiply will have mechanisms with which to leave the body and transmit disease, thereby propagating their species.
MOLECULAR FACTORS AND PROCESSES IN RESPIRATORY INFECTIONS
The study of pathogenic microorganisms has benefited greatly from the ability to identify microbial factors that are at work to elicit a particular pathologic process. Often these factors by themselves are responsible for a particular aspect of the infectious process, whereas at other times these factors act in consort to promote microbial colonization, growth, infection, and ultimately host responses and disease.
The success of the microorganism in establishing infection (the presence of a microorganism in a tissue where it is not normally found) and causing disease (the signs and symptoms of clinical illness) is predicated on the organism’s ability to elaborate specific molecular factors that allow it to progress from the colonization to the disease state. Factors that inhibit or neutralize the host’s response to eliminate the organism are also critical for pathogenesis. The ability of specific microorganisms to produce virulence or pathogenic factors, even among bacterial strains of the same species, is highly variable and is doubtless a major reason for the differences in pathogenicity among closely related strains of bacteria. Initially, most microbes that establish themselves in the respiratory tract will bind to host tissues including mucins and other factors in mucus, often in a specific manner. For example, P. aeruginosa places at the tip of the flagella that provide mobility to the organism, a protein called FliD that mediates adherence to respiratory mucin7 and H. influenzae similarly uses its pilus to bind to mucins8 and anchor the cells within the respiratory tract. Pathogens will produce specific molecules to promote this process. Some potentially pathogenic microorganisms, such as S. pneumoniae, S. aureus, and N. meningitidis, can establish colonization in the nasopharynx or throat without causing harmful effects. Almost everyone is colonized by these potential pathogens many times during life. Viruses and obligate intracellular parasites, such as Chlamydia
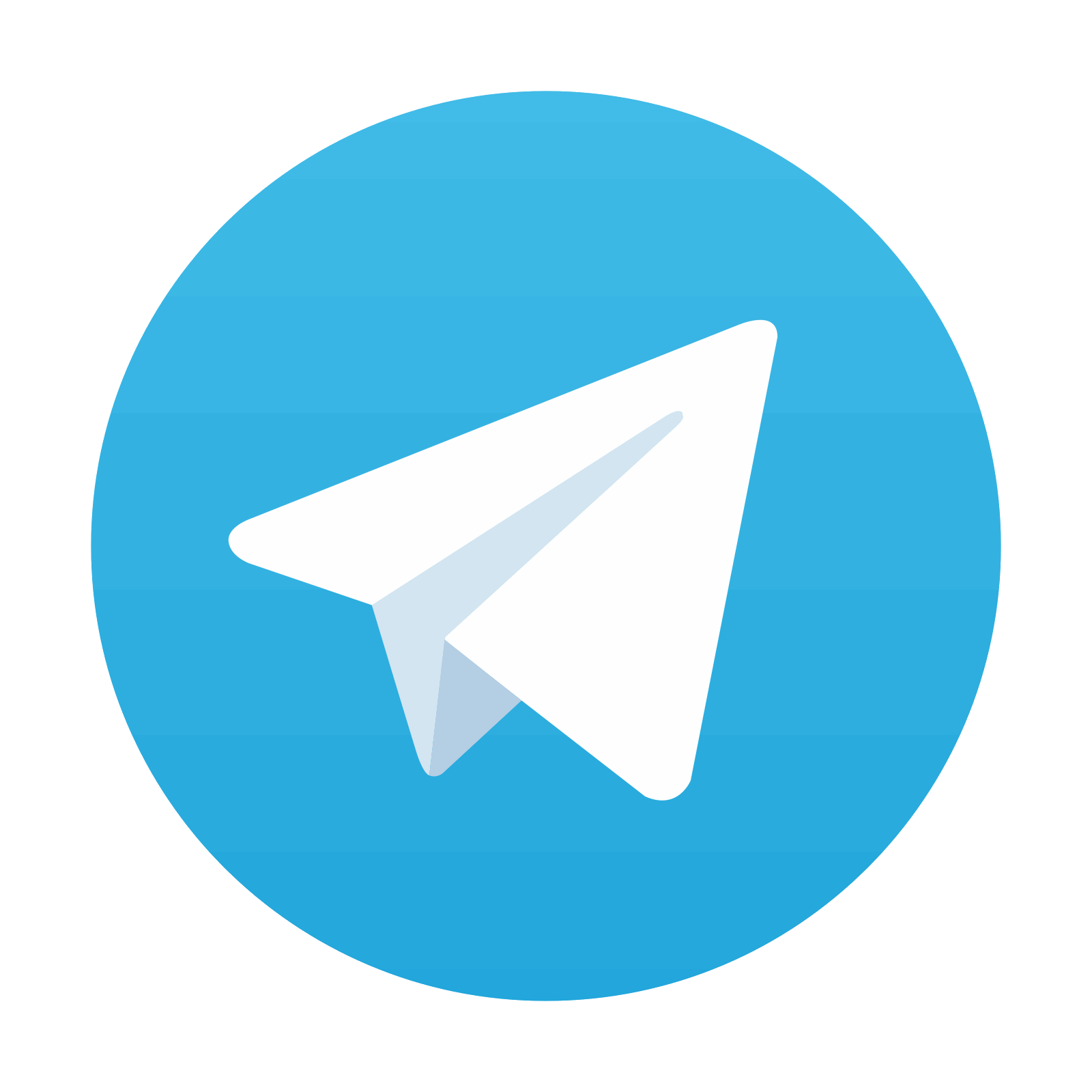
Stay updated, free articles. Join our Telegram channel

Full access? Get Clinical Tree
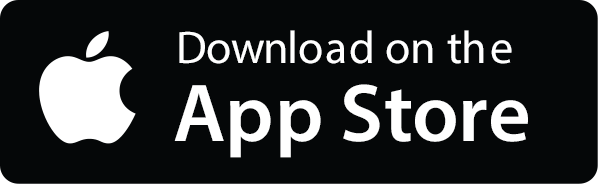
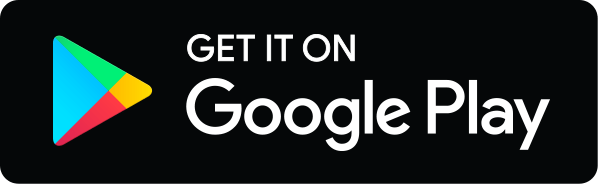