Fig. 20.1
Colored arteriogram of arteries of healthy heart ((c) SPL/Science Source Images, used with permission)
Heart failure (HF) is a physiologic state in which the heart either cannot pump enough blood to meet the metabolic needs of the body or requires an elevated filling pressure to meet these needs. The American College of Cardiology/American Heart Association (ACC/AHA) Task Force on Practice Guidelines defines HF as “a complex clinical syndrome that can result from any structural or functional cardiac disorder that impairs the ability of the ventricle to fill with or eject blood” [1, 2].
Heart failure can be caused by myocardial failure, valvular heart disease, or pericardial disease. Myocardial failure denotes dysfunction of the myocardial cells due to apoptosis, necrosis, myocyte hypertrophy, or fibrosis of the extracellular matrix [3]. Most commonly, ischemia or infarction causes myocardial failure, which subsequently progresses to HF. Heart failure was formerly referred to as congestive heart failure, denoting a frequent clinical symptom of dyspnea from pulmonary edema. However, the clinical symptoms of HF can vary from congestive symptoms, such as dyspnea and peripheral edema, to low-output symptoms, including fatigue and the cardiorenal syndrome. Today, heart failure is the preferred term, to be inclusive of those patients without symptoms of congestion. European guidelines also define acute heart failure further and draw a distinction between de novo heart failure and acute decompensated heart failure. De novo HF describes the initial presentation of HF. In contrast, acute decompensated HF refers to cases of HF in which symptoms exacerbate in a patient with a previous history of heart failure [4].
Most clinical trials for HF have focused attention on the subset of patients with left ventricular (LV) systolic dysfunction. The results of these trials form the basis of our current approach to patients with HF. Left ventricular systolic dysfunction is defined as a reduction in ejection fraction (EF), reflecting impaired contraction of the left ventricle. However, numerous epidemiologic studies have revealed that nearly half of patients with HF symptoms have a preserved EF [5–9]. Initial conclusions were based on the assumption that these patients’ disorder is mainly one of diastolic function, thereby generating the terms diastolic dysfunction and diastolic heart failure [10–12]. The term diastolic dysfunction has been supplanted by HF with preserved EF because of controversy regarding the exact pathophysiology of HF in these patients.
Patients with preserved EF are more likely to be women and to have hypertension and are less likely to have coronary artery disease (CAD) than patients with a reduced EF [13–15]. Overall, compared with patients suffering from systolic heart failure, patients with preserved EF appear to have a lower inpatient mortality but similar morbidity in terms of need for days of hospitalization [8, 13, 14].
In 2005, the ACC/AHA Task Force defined the stages of HF to emphasize this disease’s progressive nature, thereby encouraging healthcare providers to identify high-risk patients and implement preventative strategies (Fig. 20.2) [1]. Patients with CAD are well represented in stages A and B, at which aggressive medical strategies can prevent deleterious ventricular remodeling and the development of HF symptoms, as well as promote reverse remodeling of the left ventricle.
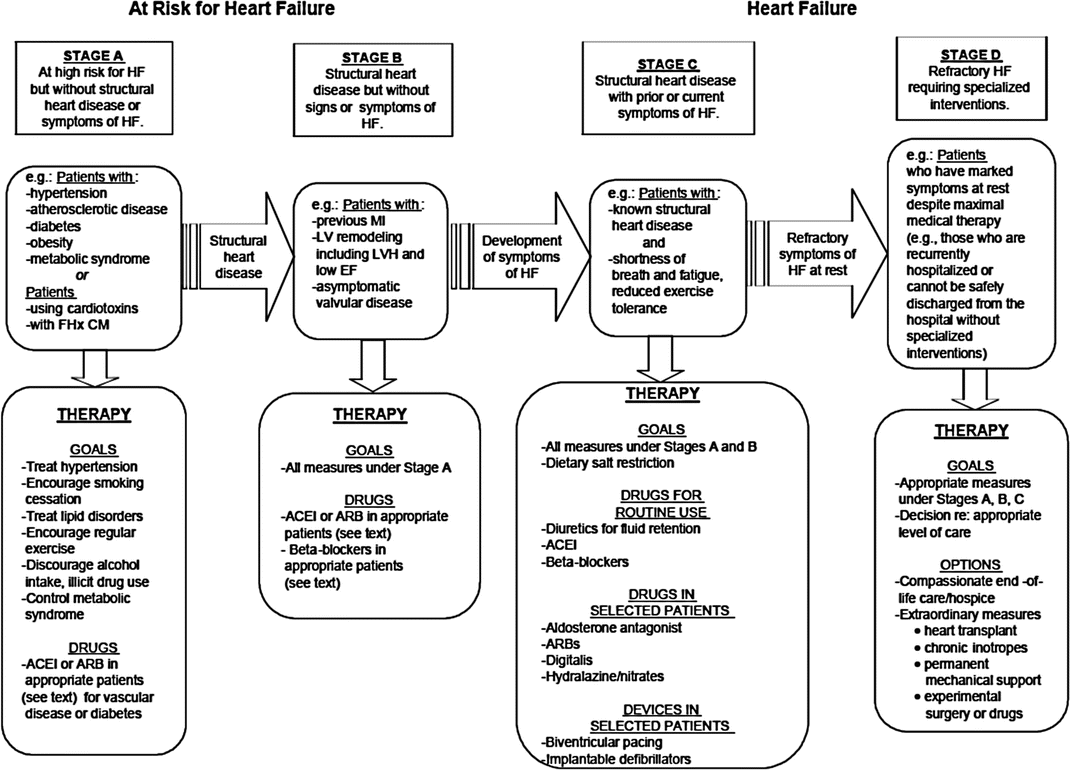
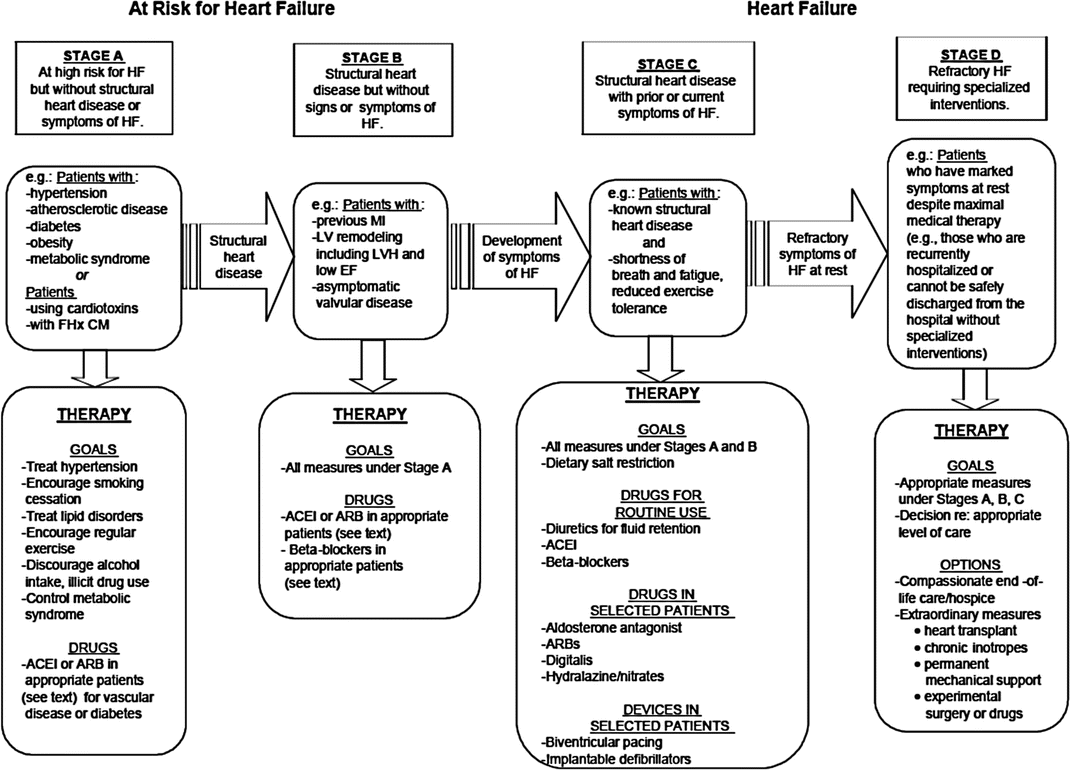
Fig. 20.2
Stages in the development of heart failure and recommendations. FHx CM family history of cardiomyopathy, ACEI angiotensin converting enzyme inhibitors, ARB angiotensin receptor blocker (From Hunt et al. [1]. Reprinted with permission from Wolters Kluwer Health)
The New York Heart Association (NYHA) classification for HF reflects a patient’s exercise capacity at a given point in time and complements the ACC/AHA staging system. When mechanical circulatory support was introduced for patients with advanced HF, neither the NYHA classification nor the ACC/AHA staging system could discern which patients with advanced HF would benefit from a surgically implanted ventricular assist device. The Interagency Registry for Mechanically Assisted Circulatory Support (INTERMACS) Coordinator’s Council developed seven clinical profiles to better discriminate among the various stages of clinically decompensated advanced heart failure that previously were all grouped under the broad heading of Stage D Heart Failure (Table 20.1) [16].
Table 20.1
INTERMACS profiles for advanced heart failure
INTERMACS profile descriptions | Time frame for intervention |
---|---|
Profile 1: Critical cardiogenic shock | |
Patients with life-threatening hypotension despite rapidly escalating inotropic support, critical organ hypoperfusion, often confirmed by worsening acidosis and/or lactate levels. “Crash and burn.” | Definitive intervention needed within hours. |
Profile 2: Progressive decline | |
Patient with declining function despite intravenous inotropic support, may be manifest by worsening renal function, nutritional depletion, inability to restore volume balance “Sliding on inotropes.” Also describes declining status in patients unable to tolerate inotropic therapy. | Definitive intervention needed within few days. |
Profile 3: Stable but inotrope dependent | |
Patient with stable blood pressure, organ function, nutrition, and symptoms on continuous intravenous inotropic support (or a temporary circulatory support device or both), but demonstrating repeated failure to wean from support due to recurrent symptomatic hypotension or renal dysfunction “Dependent stability.” | Definitive intervention elective over a period of weeks to few months. |
Profile 4: Resting symptoms | |
Patient can be stabilized close to normal volume status but experiences daily symptoms of congestion at rest or during ADL. Doses of diuretics generally fluctuate at very high levels. More intensive management and surveillance strategies should be considered, which may in some cases reveal poor compliance that would compromise outcomes with any therapy. Some patients may shuttle between 4 and 5. | Definitive intervention elective over period of weeks to few months. |
Profile 5: Exertion intolerant | |
Comfortable at rest and with ADL but unable to engage in any other activity, living predominantly within the house. Patients are comfortable at rest without congestive symptoms, but may have underlying refractory elevated volume status, often with renal dysfunction. If underlying nutritional status and organ function are marginal, patient may be more at risk than INTERMACS 4, and require definitive intervention. | Variable urgency, depends upon maintenance of nutrition, organ function, and activity. |
Profile 6: Exertion limited | |
Patient without evidence of fluid overload is comfortable at rest, and with activities of daily living and minor activities outside the home but fatigues after the first few minutes of any meaningful activity. Attribution to cardiac limitation requires careful measurement of peak oxygen consumption, in some cases with hemodynamic monitoring to confirm severity of cardiac impairment. “Walking wounded.” | Variable, depends upon maintenance of nutrition, organ function, and activity level. |
Profile 7: Advanced NYHA III | |
A placeholder for more precise specification in future, this level includes patients who are without current or recent episodes of unstable fluid balance, living comfortably with meaningful activity limited to mild physical exertion. | Transplantation or circulatory support may not currently be indicated. |
Modifiers for profiles | Possible profiles to modify |
TCS-temporary circulatory support – can modify only patients in hospital (other devices would be INTERMACS devices). Includes IABP, ECMO, TandemHeart, Levitronix, BVS 5000 or AB5000, Impella. | 1,2,3 in hospital. |
A-arrhythmia – can modify any profile. Recurrent ventricular tachyarrhythmias that have recently contributed substantially to clinical compromise. This includes frequent ICD shock or requirement for external defibrillator, usually more than twice weekly. | Any profile. |
FF-frequent flyer – can modify only outpatients, designating a patient requiring frequent emergency visits or hospitalizations for diuretics, ultrafiltration, or temporary intravenous vasoactive therapy. | 3 if at home, 4,5,6. A frequent flyer would rarely be profile 7. |
Prevalence of Coronary Artery Disease in Heart Failure
On the basis of 2007–2010 data from the National Health and Nutrition Examination Survey, it is estimated that 5.1 million Americans greater than 20 years old have heart failure (HF) [17]. In the NHLBI-sponsored Framingham Heart Study, hypertension was a common risk factor for HF, followed closely by antecedent MI [18]. In the Atherosclerosis Risk in Communities (ARIC) study, elevated glycohemoglobin A1C levels of 5.5–6.0 % in the middle-aged population were also significantly associated with incident heart failure [19].
Coronary heart disease is common in the population and is the leading cause of morbidity and mortality in the United States, affecting over 16 million Americans and accounting for 1 out of every 6 deaths in the United States in 2008 [20]. The ADHERE Registry was formed to study the characteristics, management, and outcomes of a broad sample of patients hospitalized in North America for acute decompensated heart failure. Of the first 104,362 patients studied in the Registry, it was found that 73 % had hypertension, 57 % had CHD, and 44 % had diabetes mellitus [21]. Heart failure can be an acute complication of acute MI, but it is also a common long-term sequela of myocardial infarction (MI); its incidence varies with patient age and sex and ranges from 8 to 23 % within 5 years after MI [20]. By improving survival after acute MI (AMI), the advent of reperfusion therapy has generated a population of patients who are at high risk of developing HF [22]. Despite the wealth of data demonstrating the benefits of early institution of angiotensin-converting enzyme (ACE) inhibitors and β-blockers after AMI, a significant number of patients have adverse ventricular remodeling and progress to overt clinical HF. In addition to traditional risk factors (i.e., hypertension and diabetes), obesity, physical inactivity, dyslipidemia, and sleep apnea have been shown to increase the risk of HF in these patients [23–26]. Recent studies have identified biomarkers—N-terminal pro-brain natriuretic peptide and urinary albumin-to-creatinine ratio—that reflect ventricular remodeling and endothelial dysfunction, respectively, thereby enhancing the health care provider’s ability to predict which patients will develop progressive HF [27, 28]. More accurate identification of CAD patients who are at high risk of developing HF provides an opportunity for aggressive risk factor modification and preventative strategies.
Pathogenesis of Acute Heart Failure in Patients with Coronary Artery Disease
The progression of CAD to HF can vary from abrupt cardiogenic shock after AMI to the insidious development of LV failure in patients with chronic coronary heart disease. Patients with CAD develop acute HF either as a complication of an acute coronary syndrome (ACS) or in the absence of classic myocardial ischemia. An elevated plasma level of cardiac troponin, a biomarker of myocardial injury, is generally considered to confirm the diagnosis of ACS. However, cardiac troponin levels can also be elevated in patients with acute decompensated HF in the absence of ACS; these elevated levels correlate with a worse prognosis [29–32]. Acute heart failure syndrome (AHFS) has a heterogeneous pathophysiology due to overlapping mechanisms from precipitating factors other than ischemia. Table 20.2 lists causes and precipitating factors of AHFS, which can perturb the hemodynamics of patients with chronic coronary heart disease, thereby prompting hospital admission for HF [33].
Table 20.2
Causes and precipitating factors of acute heart failure
Ischaemic heart disease | Circulatory failure |
Acute coronary syndromes | Septicaemia |
Mechanical complications of acute MI | Thyrotoxicosis |
Right ventricular infarction | Anaemia |
Valvular | Shunts |
Valve stenosis | Tamponade |
Valvular regurgitation | Pulmonary embolism |
Endocarditis | Decompensation of pre–existing chronic HF |
Aortic dissection | Lack of adherence |
Myopathies | Volume overload |
Postpartum cardiomyopathy | Infections, especially pneumonia |
Acute myocarditis | Cerebrovascular insult |
Hypertension/arrhythmia | Surgery |
Hypertension | Renal dysfunction |
Acute arrhythmia | Asthma, COPD |
Drug abuse | |
Alcohol abuse |
Cardiogenic shock occurs in 5–10 % of patients admitted with AMI and remains the leading cause of death after AMI [34–38]. In the SHOCK (SHould we emergently revascularize Occluded Coronaries for cardiogenic shocK?) trial and registry, nearly half of the patients with cardiogenic shock complicating their AMI developed shock after admission to the hospital [39]. Cardiogenic shock has been defined by four clinical criteria: inability to maintain a systolic blood pressure of more than 90 mmHg without inotropic or vasoconstrictor agents, a heart rate greater than 60 beats per minute, the need for vasopressors or intra-aortic balloon counterpulsation to keep systolic blood pressure above 90 mmHg, and evidence of end-organ hypoperfusion (i.e., cool extremities or reduced urine output). The hemodynamic diagnostic criteria for shock include a cardiac index of less than or equal to 2.2 L/min/m2 and a pulmonary capillary wedge pressure of greater than or equal to 15 mmHg [39]. Cardiogenic shock occurs as a sequela in both ST-segment elevation MI (STEMI) and non-STEMI, but with notable differences. Patients with STEMI who develop shock on admission or soon thereafter are more likely to have an occluded infarct artery, whereas patients with non-STEMI develop shock later in their hospital course, are older, and are more likely to have diabetes mellitus, three-vessel disease, or both [40].
Pathogenesis of Chronic Heart Failure in Patients with Coronary Artery Disease
In patients with CAD, chronic HF can evolve from various processes that contribute to structural changes in the heart and may include multiple concomitant events. Left ventricular remodeling after an MI results from myocyte ischemia, necrosis, and death, thereby increasing LV cavity wall stress and activating the neurohormonal cascade. This process results in myocardial fibrosis and myocyte hypertrophy, which ultimately leads to LV cavity dilatation and LV dysfunction [41, 42]. The extent of myocardial damage can be ameliorated with reperfusion therapy and the use of ACE inhibitors, β-blockers, and eplerenone [43]. Many patients who suffer an MI have a significant burden of atherosclerotic disease in their remaining coronary arteries [44]. Myocardial ischemia, stunning, and hibernation can occur outside the infarct zone and exacerbate the initial LV dysfunction caused by a myocardial infarct. Furthermore, endothelial dysfunction inherent in patients with CAD directly affects ventricular function by decreasing the production of vasodilators, nitric oxide, and prostacyclin and increasing the production of the potent vasoconstrictors endothelin and angiotensin II [45]. Sudden death is a great concern in patients with CAD and HF because of the risk of ventricular arrhythmias due to myocardial ischemia and reentry in the infarct scar [46].
Treatment of Acute Heart Failure in Patients with Coronary Artery Disease
Heart failure is a growing epidemic because of the aging of the population, success in treating patients with acute CAD, and the use of implantable defibrillators to prevent sudden death. The economic burden in the United States is enormous, with more than 1 million hospitalizations annually and a projected cost of over $44 billion annually by 2015 [20]. The largest expense is hospital care for patients with AHFS.
A hospital stay for AHFS mandates finding the cause of the patient’s HF and provides an opportunity to identify reversible causes, the correction of which can mitigate the progressive course of HF. The majority of patients who present with AHFS have CAD. It is paramount to initially determine whether the patient has ACS complicated by HF or AHFS with underlying CAD. To date, practice guidelines do not address the most appropriate method or timing for detecting or reassessing CAD in patients with AHFS. Patients with CAD often have other comorbidities, including hypertension, atrial fibrillation, valvular disease, thyroid disease, anemia, and sleep disordered breathing, that can accelerate the underlying ischemia, leading to myocyte dysfunction and AHFS.
The existing guidelines from the ACC/AHA, Heart Failure Society of America, and European Society of Cardiology recommend common laboratory tests, chest radiography, electrocardiography, and echocardiography (if not recently performed) to assess the patient with AHFS [2, 33, 47]. Patients with LV systolic dysfunction and electrocardiographic Q waves often have significant CAD. Although segmental wall motion abnormalities on echocardiography are predictive of CAD, they do not correlate with the severity of disease. Furthermore, most patients with HF and CAD do not have Q waves, and patients with nonischemic cardiomyopathy can have Q waves [48]. Hence, coronary angiography remains the gold standard for new diagnosis of CAD, as well as the reassessment of CAD extent in those with chronic coronary heart disease. It is disconcerting that three large AHFS registries and observational studies of community-based practice have shown that angiography is not commonly performed in patients with newly diagnosed AHFS [49]. Furthermore, ACS patients with HF are less likely to undergo coronary angiography and revascularization than ACS patients without HF [49].
Patients with AHFS who have demonstrable ischemia and obstructive CAD on angiography should be referred for early myocardial revascularization in addition to treatment with evidence-based medical therapy, including antiplatelet agents, statins, β-blockers, and ACE inhibitors or angiotensin receptor blockers (ARBs). In the absence of clinical signs of ischemia, nuclear perfusion imaging, dobutamine stress echocardiography, or positron emission tomography can be used to test for ischemia. Up to 50 % of all patients with CAD and LV dysfunction have viable myocardium that is dysfunctional because of stunning or hibernation [50]. Viable myocardium can be identified with single-photon emission computed tomography imaging, positron emission tomography, low-dose dobutamine echocardiography, or cardiac magnetic resonance imaging [51–53]. Patients who have viable myocardium before revascularization have better outcomes afterward than patients with no viable myocardium [54, 55].
Three phases of in-hospital management of patients with AHFS have been described. Phase I focuses on the initial stabilization and urgent treatment of the patient, most often in the emergency department. Phase II consists of in-hospital management to identify and correct any reversible causes and implement evidence-based therapy. Phase III focuses on the close management of the patient after discharge from the hospital [56]. The presence of CAD in patients with AHFS will affect their treatment strategy (Fig. 20.3) [49].
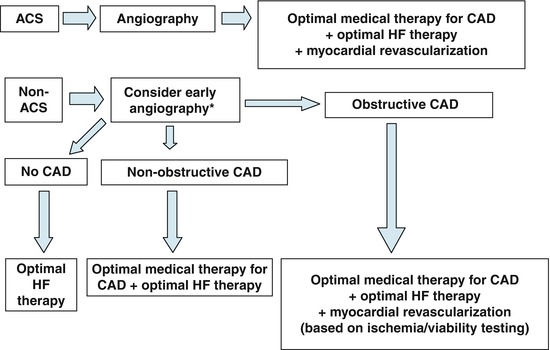
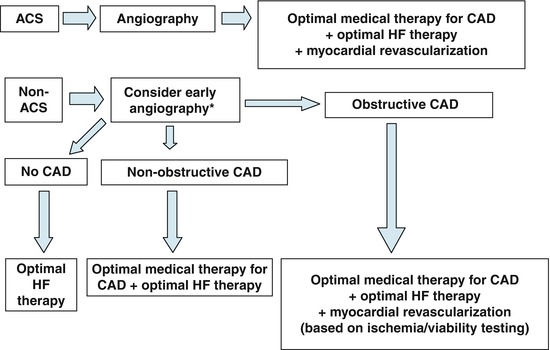
Fig. 20.3
Therapeutic strategies in patients with AHFS. *For those patients with remote or no history of coronary angiography. ACS acute coronary syndrome, CAD coronary artery disease, HF heart failure (From Flaherty et al. [49]. Reprinted with permission from Elsevier Limited)
The initial management of AHFS usually occurs in the emergency department or on arrival at the coronary care unit. The SHOCK trial showed that patients younger than 75 years of age with AMI complicated by cardiogenic shock have higher 1-year survival rates when treated with early revascularization, either by percutaneous coronary intervention (PCI) or coronary artery bypass grafting (CABG), rather than initial medical stabilization [39, 57, 58]. Furthermore, patients with AMI complicated by cardiogenic shock who present at centers without PCI capability benefit from hospital transfer despite the longer treatment time [59, 60]. When shock complicates MI, early use of intra-aortic balloon counterpulsation is recommended [61, 62]. In shock patients whose hemodynamics remain tenuous, pulmonary artery catheterization can be beneficial but should not delay transfer to the cardiac catheterization laboratory [62]. Pharmacologic therapy with intravenous fluids, inotropic agents, and vasodilators or vasoconstrictors can be tailored to a patient’s needs by the measurement of cardiac output, systemic vascular resistance, and filling pressures. The most common cause of cardiogenic shock is LV failure, but right ventricular (RV) failure due to a right coronary artery occlusion or mechanical complication of MI can also be responsible. Two-dimensional echocardiography with Doppler imaging is recommended to evaluate RV function and exclude the presence of LV free wall rupture, ventricular septum rupture, or papillary muscle dysfunction causing severe mitral regurgitation [62–64]. Prompt cardiac surgical consultation is imperative for patients who have mechanical complications or may require mechanical circulatory support to reverse their cardiogenic shock.
Vasodilators
In AHFS patients with underlying CAD who are not hypotensive, nitrates are the ideal initial therapy to improve coronary perfusion and alleviate chest pain. For those AHFS patients with severe pulmonary edema, a combination of high-dose nitrates and low-dose diuretics decreases the need for mechanical ventilation and lowers the risk of MI [65].
Intravenous nitroglycerin is primarily used to reduce preload, relieve symptoms of congestion, and mitigate coronary ischemia. This drug may be useful in treating symptomatic mitral regurgitation. However, nitroglycerin is associated with tachyphylaxis and should generally be used for less than 24 h.
Nesiritide (human BNP) is a vasodilator that reduces LV filling pressure but has inconsistent effects on cardiac output and sodium excretion. It appears to be effective at reducing dyspnea, but renal function may be compromised and should be carefully monitored [66–68].
In the Acute Decompensated Heart Failure National Registry (ADHERE), the use of intravenous nitroglycerin or nesiritide, a recombinant form of human BNP, was associated with lower in-hospital mortality than the use of intravenous dobutamine or milrinone [69]. However, intravenous nitroglycerin causes less renal deterioration and may better promote survival at 30 days than intravenous nesiritide [70, 71].
Sodium nitroprusside, a balanced preload and afterload vasodilator, has nitric oxide as an active metabolite, which promotes vasodilatation in the pulmonary vasculature. It is a useful drug for treating hypertensive crisis or acute mitral regurgitation because this drug can rapidly lower preload and afterload. Nitroprusside has the potential to cause severe hypotension, so an arterial line should be placed when this agent is used, especially in patients with renal insufficiency. Patients must also be monitored closely for possible thiocyanate toxicity [2, 33]. This drug produces hypotension, so it is best suited for congested and hypertensive patients and possibly those with mitral regurgitation.
Inotropes
Inotropes with vasodilatory properties can benefit AHFS patients by decreasing pulmonary capillary wedge pressure and increasing cardiac output but may be deleterious in patients with CAD. A decrease in blood pressure may reduce coronary perfusion and exacerbate any underlying myocardial ischemia. In addition, inotropes increase myocardial oxygen demand by enhancing the contraction of myocytes and increasing the heart rate, further exacerbating ischemia and potentially promoting myocyte necrosis [72]. In AHFS patients with elevated troponin levels, in-hospital mortality is significantly higher when inotropes are used [30].
Dobutamine, a nonselective β-agonist that has dose-dependent activity on the α-receptor, is the most commonly used inotrope in the United States [69]. At low doses (2–3 μg/kg/min), vasorelaxation predominates; at higher doses, the α1 receptor is more activated, causing venous and arterial vasoconstriction.
Milrinone, a phosphodiesterase inhibitor, is also used as an inotrope, but the OPTIME-CHF (Outcomes of a Prospective Trial of Intravenous Milrinone for Exacerbations of Chronic Heart Failure) trial associated the use of milrinone in CAD patients with increased mortality after discharge [73]. Therefore, among AHFS patients with CAD, the use of milrinone should be reserved for those with a low cardiac output state refractory to non-inotropic therapy. Moreover, a loading dose should be avoided to prevent hypotension and to avoid potentially exacerbating underlying ischemia.
Dopamine is an inotrope that causes dose-dependent activation of various receptors. At low doses (≤2 μg/kg/min), dopamine binds to vascular D1 receptors in the coronary, renal, and mesenteric beds, causing vasodilation and natriuresis. At intermediate doses (2–5 μg/kg/min), dopamine activates β1 receptors, resulting in a positive inotropic effect. At high doses (5–15 μg/kg/min), dopamine also binds to α1 receptors, thereby causing vasoconstriction. Low-dose dopamine is often used with dobutamine or milrinone to enhance renal perfusion.
Epinephrine, an endogenous catecholamine, binds to β1, β2, and α1-receptors. At low doses, β-adrenergic effects predominate, resulting in a powerful inotropic response. At higher doses, epinephrine primarily stimulates α1-receptors, resulting in vasoconstriction. Prolonged high-dose epinephrine administration can cause cardiac toxicity and limb ischemia by damaging arterial walls [74].
Isoproterenol, a nonselective synthetic β-adrenergic agonist with a very low affinity for α-receptors, has a powerful chronotropic and inotropic effect. Isoproterenol is an ideal agent to treat RV failure and is often used in the early postoperative management of heart transplant recipients.
Levosimendan, a calcium sensitizer and adenosine triphosphate–dependent potassium channel agonist, is a positive inotrope and a vasodilator. In patients with AHFS, levosimendan has been found to have no effect on survival when compared to placebo or dobutamine. However, levosimendan was associated with more hypotension, hypokalemia, ventricular tachycardia (VT), and atrial fibrillation but fewer recurrent hospitalizations for HF when compared to dobutamine [75].
Vasopressors
Vasopressor agents (Table 20.3) are occasionally used as rescue agents in patients with AHFS when the hypotension is due to systemic vasodilatation rather than a decreased cardiac output. These agents improve coronary and end-organ perfusion until other therapies can be implemented to improve systemic resistance. Vasopressors are best given through a central line and titrated with the guidance of invasive hemodynamic monitoring.
Table 20.3
Inotropic and vasopressor drug names, clinical indications for therapeutic use, standard dose ranges, receptor binding (catecholamines), and major clinical side effects
Drug | Clinical indication | Dose range | Receptor binding | Major side effects | |||
---|---|---|---|---|---|---|---|
α1 | β1 | β2 | DA | ||||
Catecholamines | |||||||
Dopamine | Shocka | 2.0–20 μg · kg−1 · min−1 (max 50 μg · kg−1 · min−1) | +++ | ++++ | ++ | +++++ | Severe hypertensionb |
HF | Ventricular arrhythmias | ||||||
Symptomatic bradycardia | Cardiac ischemia | ||||||
Unresponsive to atropine or pacing | Tissue ischemia/gangrene (high doses or due to tissue extravasation) | ||||||
Dobutamine | Low CO (decompensated HF, cardiogenic shock, sepsis-induced myocardial dysfunction) | 2.0–20 μg · kg−1 · min−1 (max 40 μg · kg−1 · min−1) | + | +++++ | +++ | N/A | Tachycardia |
Symptomatic bradycardia unresponsive to atropine or pacing | Increased ventricular response rate in patients with atrial fibrillation | ||||||
Ventricular arrhythmias | |||||||
Cardiac ischemia | |||||||
Hypertensionb | |||||||
Hypotension | |||||||
Norepinephrine | Shocka | 0.01–3 μg · kg−1 · min−1 | +++++ | +++ | ++ | N/A | Arrhythmias |
Bradycardia | |||||||
Peripheral (digital) ischemia | |||||||
Hypertensionb | |||||||
Epinephrine | Shocka | Infusion: 0.01–0.10 μg · kg−1 · min−1 | +++++ | ++++ | +++ | N/A | Ventricular arrhythmias |
Cardiac arrest | Bolus: 1 mg IV every 3–5 min (max 0.2 mg/kg) | Severe hypertension resulting in cerebrovascular hemorrhage | |||||
Bronchospasm/anaphylaxis | IM: (1:1,000): 0.1–0.5 mg (max 1 mg) | Cardiac ischemia | |||||
Symptomatic bradycardia or heart block unresponsive to atropine or pacing | Sudden cardiac death | ||||||
Isoproterenol | Bradyarrhythmias (especially torsade des pointes) | 2–10 μg/min | 0 | +++++ | +++++ | N/A | Ventricular arrhythmias |
Brugada syndrome | Cardiac ischemia | ||||||
Hypertension | |||||||
Hypotension | |||||||
Phenylephrine | Hypotension (vagally mediated, medication-induced) | Bolus: 0.1–0.5 mg IV every 10–15 min | +++++ | 0 | 0 | N/A | Reflex bradycardia |
Increase MAP with AS and hypotension | Infusion: 0.4–9.1 μg · kg−1 · min−1 | Hypertensionb | |||||
Decrease LVOT gradient in HCM | Severe peripheral and visceral vasoconstriction | ||||||
Tissue necrosis with extravasation | |||||||
PDIs | |||||||
Milrinone | Low CO (decompensated HF, after cardiotomy) | Bolus: 50 μg/kg bolus over 10–30 min | N/A | Ventricular arrhythmias | |||
Infusion: 0.375–0.75 μg · kg−1 · min−1 (dose adjustment necessary for renal impairment) | Hypotension | ||||||
Cardiac ischemia | |||||||
Torsade des pointes | |||||||
Amrinone | Low CO (refractory HF) | Bolus: 0.75 mg/kg over 2–3 min | N/A | Arrhythmias, enhanced AV conduction (increased ventricular response rate in atrial fibrillation) | |||
Infusion: 5–10 μg · kg−1 · min−1 | Hypotension | ||||||
Thrombocytopenia | |||||||
Hepatotoxicity | |||||||
Vasopressin | Shocka | Infusion: 0.01–0.1 U/min (common fixed dose 0.04 U/min) | V1 receptors (vascular smooth muscle) | Arrhythmias | |||
Cardiac arrest | Bolus: 40-U IV | V2 receptors (renal collecting duct system) | Hypertension | ||||
Decreased CO (at doses >0.4 U/min) | |||||||
Cardiac ischemia | |||||||
Severe peripheral vasoconstriction causing ischemia (especially skin) | |||||||
Splanchnic vasoconstriction | |||||||
Levosimendan | Decompensated HF | Loading dose: 12–24 μg/kg over 10 min | N/A | Tachycardia, enhanced AV conduction | |||
Infusion: 0.05–0.2 μg · kg−1 · min−1 | Hypotension |
Norepinephrine, an endogenous neurotransmitter, is a potent α1-adrenergic receptor agonist with modest β-agonist activity, which makes it a powerful vasoconstrictor with only mild inotropic effects. Norepinephrine increases systolic, diastolic, and pulse pressure with a minimal chronotropic effect, making it ideal when a heart rate increase is undesirable. Prolonged use can have direct toxicity to cardiac myocytes [74].
Phenylephrine is a potent synthetic α-adrenergic agonist with no affinity for β-receptors. It is an ideal agent for rapidly correcting sudden, severe hypotension. Phenylephrine is the preferred agent for relieving severe hypotension in patients with aortic stenosis, correcting hypotension caused by the co-administration of sildenafil and nitrates, increasing cardiac output in patients with obstructive hypertrophic cardiomyopathy, and correcting vagally mediated hypotension.
Vasopressin, or “antidiuretic hormone,” exerts its actions by binding to three types of receptors: V1a, V1b, and V2. The V1a receptors, located in vascular smooth muscle, mediate vasoconstriction; V1b receptors, found in the anterior pituitary and brain, regulate neural pathways; and V2 receptors, predominately located in the renal collecting duct system, mediate antidiuretic hormone effects on water reabsorption [74, 76]. Vasopressin causes less direct coronary and cerebral vasoconstriction than catecholamines do. It also has either a neutral or an inhibitory effect on cardiac output, depending on the dose, which determines how much the drug increases systemic vascular resistance and vagal tone [74].
Vasopressin Antagonists
Vasopressin antagonists have been approved for use in AHFS patients with hypervolemic or euvolemic hyponatremia. Vasopressin levels are inappropriately high in both acute and chronic HF. Tolvaptan, an oral selective V2 receptor antagonist, can improve serum sodium levels in patients with hyponatremia related to chronic HF, cirrhosis, or the syndrome of inappropriate antidiuretic hormone secretion [77]. The Efficacy of Vasopressin Antagonism in Heart Failure Outcome Study with Tolvaptan (EVEREST) was an international trial that enrolled 4,133 patients admitted with AHFS and reduced EF. Although tolvaptan had no obvious mortality benefit, the drug improved signs and symptoms of HF during hospitalization and reduced body weight without adversely affecting heart rate or blood pressure [78, 79]. The addition of conivaptan, a V1a and V2 antagonist for intravenous use, has been shown in a pilot study to increase urine output in AHFS patients but without significantly improving their signs or symptoms of HF or reducing body weight [80]. These two vasopressin antagonists have been approved for use in treating AHFS, but their exact role in the management of HF has yet to be fully elucidated.
Ultrafiltration
Peripheral ultrafiltration is a therapeutic option for patients with HF that is refractory to pharmacologic therapy. The Ultrafiltration Versus Intravenous Diuretics for Patients Hospitalized for Acute Decompensated Heart Failure (UNLOAD) trial enrolled 200 patients with AHFS and reduced or preserved EF. The patients randomly assigned to ultrafiltration showed a greater reduction of body weight at 48 h, a reduced need for vasoactive drug use, and a reduced rate of hospital readmission rate at 90 days. However, dyspnea scores and renal function were similar in both arms [81]. Ultrafiltration should be considered in patients who have severe volume overload that remains refractory to pharmacologic therapy.
Statin Therapy
Statin therapy is strongly recommended for patients with CAD [82, 83]. In addition to their cholesterol-lowering effects, statin drugs have pleiotropic effects, such as plaque stabilization, improvement of endothelin function, increased nitric oxide bioavailability, antioxidant properties, and anti-inflammatory effects [84]. The Cholesterol and Recurrent Events (CARE) trial showed that pravastatin reduces cardiac risk in patients with MI, mild elevation in serum cholesterol, and reduced EF [85]. In the Scandinavian Simvastatin Survival Study (4S), simvastatin decreased the incidence of new-onset HF in patients after MI. Furthermore, simvastatin reduced mortality in the patients who developed HF [86]. Statin therapy also has been found to be useful for the prevention and treatment of HF in large observational studies, small prospective studies, and post hoc analyses of cardiovascular databases. However, two large, prospective, controlled trials showed that rosuvastatin had no effect on the survival of patients with chronic HF. The benefit of statin therapy appears to be due to its ability to halt the progression of HF in patients with CAD. Therefore, statin therapy should be started or continued in CAD patients admitted with AHFS unless contraindicated [87].
Antiplatelet Therapy
The use of antiplatelet therapy—aspirin or clopidogrel—is strongly recommended for the secondary prevention of cardiovascular events in patients with CAD [83]. Dual therapy with a P2Y12 receptor antagonist (clopidogrel, prasugrel, or ticagrelor) and aspirin is indicated after ACS or PCI with stent placement [83]. The combination of clopidogrel and aspirin reduces the incidence of HF by 18 % in patients with ACS [88]. Antiplatelet therapy can limit future ischemic events in patients with CAD, which probably contributes to its protective effect against HF.
Anticoagulant Therapy
The use of warfarin in addition to low-dose aspirin therapy is indicated for patients who have a concomitant indication for anticoagulation, such as atrial fibrillation, LV thrombus, mechanical prosthetic heart valve, or venous thromboembolic disease [83]. For patients who require warfarin therapy, doses should be adjusted to meet the recommended international normalized ratio for their concomitant condition. The use of warfarin with antiplatelet therapy increases the risk of bleeding complications, warranting close monitoring during hospitalization and outpatient treatment. Furthermore, HF patients require closer monitoring while on anticoagulant therapy because of the potential for malnutrition, hepatic congestion, and drug-drug interactions that could alter the therapeutic effect of warfarin.
Myocardial Revascularization
Surgical revascularization reduces mortality risk in patients with CAD and HF when noninvasive testing reveals dysfunctional but viable myocardium. This reduction increases with the severity of LV dysfunction. In contrast, in patients with CAD and no viable myocardium, revascularization has no apparent benefit and might increase mortality risk. Therefore, the higher surgical risk of patients with more severe LV dysfunction can be potentially offset by myocardial viability [51]. The Surgical Treatment for Ischemic Heart Failure (STICH) trial randomly assigned 2,800 patients with CAD and LV dysfunction amenable to CABG from 100 centers to combinations of three different treatment strategies: CABG, surgical ventricular reconstruction (SVR), and intensive medical therapy. The trial was powered to address two primary hypotheses: (1) CABG combined with medical therapy improves long-term survival when compared to medical therapy alone, and (2) SVR provides an additional long-term survival benefit when combined with CABG and medical therapy. Although SVR reduced the end-systolic volume index, there was no significant difference in a composite end point of death from any cause and hospitalization for cardiac causes when compared to the CABG and medical therapy arm [89]. In the Global Registry of Acute Coronary Events (GRACE), the rate of in-hospital revascularization was low in patients with NSTE-ACS complicated by HF. The HF patients who underwent revascularization had a higher survival rate 6 months after discharge than the HF patients who did not undergo revascularization [90]. The performance of in-hospital myocardial revascularization on patients with AHFS and CAD is associated with increased use of aspirin, statins, β-blockers, and ACE inhibitors at the time of discharge and corresponds with significantly lower rates of mortality and re-hospitalization [91]. These data strongly suggest that patients with AHFS and CAD would benefit from angiography during their index hospitalization and that patients with angiographically detected obstructive CAD should undergo myocardial viability assessment.
Cardiac Device Therapy
Cardiac Resynchronization Therapy/Automatic Implantable Cardioverter-Defibrillators
The efficacy of placing an automatic implantable cardioverter-defibrillator (AICD) device at the time of admission for AHFS has not been studied. Implantation of an AICD should be seriously considered for patients with HF and an EF of 35 % or less, but only after a 3–6-month trial of evidence-based medical therapy for HF [2]. Similarly, for AHFS patients with reduced EF and electrical dyssynchrony (QRS complex >120 ms), cardiac resynchronization therapy (CRT) should be considered after discharge if the patient remains symptomatic despite optimal medical therapy.
Percutaneous Mechanical Support
The ability to deploy mechanical support rapidly is desirable in patients with AHFS due to myocardial stunning who are expected to recover. In AHFS patients with refractory cardiogenic shock, percutaneous mechanical support serves as a bridge, supporting them until their condition permits surgical revascularization or placement of an implantable LV assist device (LVAD).
Intra-aortic balloon (counterpulsation) pump (IABP) is the most widely used mechanical circulatory support system in the world today. The 40–60-mL polyurethane balloon is attached to the tip of a catheter, which is inserted percutaneously into the common femoral artery and then advanced into the descending thoracic aorta (Fig. 20.4) [92]. At the start of diastole, the balloon inflates, augmenting coronary perfusion. At the beginning of systole, the balloon deflates as the blood is ejected from the left ventricle, which increases the cardiac output and decreases LV stroke work and myocardial oxygen requirements. The IABP cannot be used in patients with significant atherosclerotic or aneurysmal disease of the thoracic aorta or significant aortic regurgitation. Furthermore, adequate IABP function depends on appropriate timing of the balloon cycle; therefore, it is suboptimal in patients with arrhythmias. The IABP cannot completely unload the left ventricle and may not provide enough support in patients with severe cardiogenic shock. Potential complications of IABP use include peripheral vascular hemorrhage and thromboembolic events [93, 94]. Further questions have recently arisen about which patients may benefit from placement of an IABP with the publication of a randomized multicenter study that found that IABP support did not significantly reduce 30-day mortality in patients with cardiogenic shock complicating acute MI for whom an early revascularization strategy was planned [95].
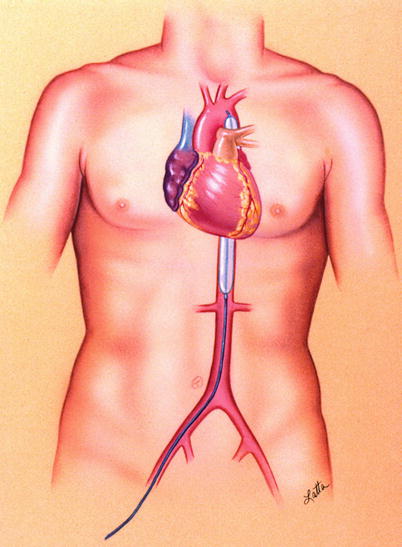
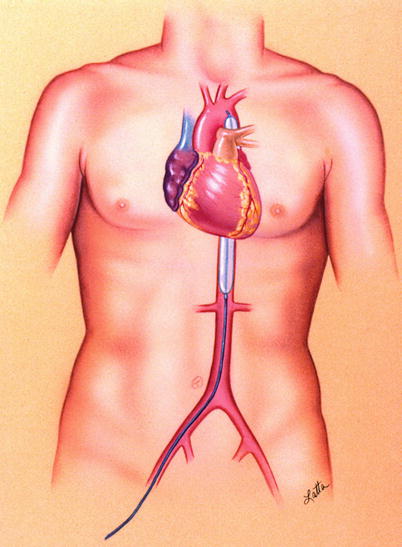
Fig. 20.4
Illustration of intra-aortic balloon pump in the body (©1997 Texas Heart Institute)
The TandemHeart Percutaneous Transseptal Ventricular Assist (PTVA) System (CardiacAssist, Inc., Pittsburgh, Pennsylvania) is a device approved by the US Food and Drug Administration (FDA) to provide temporary LV support. It can be implanted in the catheterization laboratory or the operating room. This continuous centrifugal pump can deliver up to 4 L/min of flow by diverting blood from the left atrium to the systemic circulation. A 21-F inflow cannula is inserted into the left atrium via a standard transseptal puncture through a femoral venous sheath, and a 15- or 17-F outflow cannula is placed in the femoral artery (Fig. 20.5). Results from a trial performed in 18 patients with cardiogenic shock revealed a mortality rate of 41 % [96], which is substantially lower than the 60 % rate seen in the SHOCK registry. The TandemHeart PTVA system has been used as temporary support during high-risk PCI, as a bridge to recovery, and as a bridge to long-term mechanical support [97].
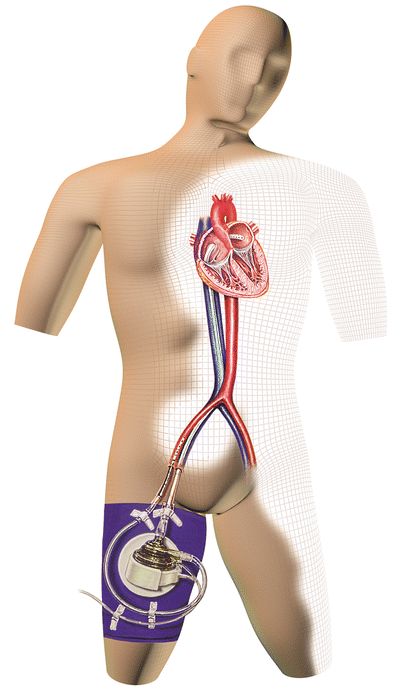
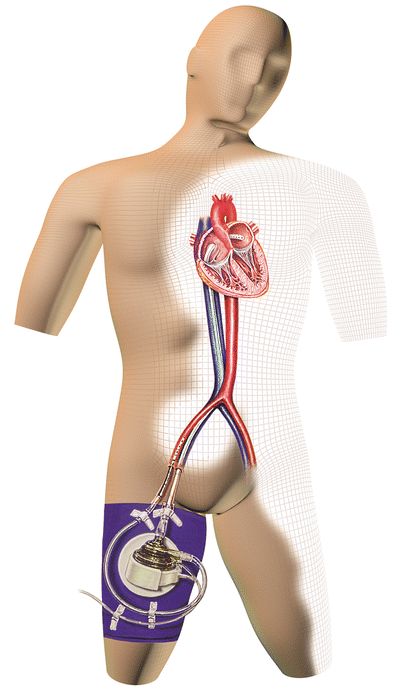
Fig. 20.5
The TandemHeart system is a left atrial-to-femoral bypass system that can be placed in the cardiac catheterization laboratory. A transseptal puncture is required, and the blood flow is delivered via a cannula that is placed in the femoral artery and whose tip is advanced to the aortic bifurcation (Courtesy of CardiacAssist, Inc.)
The Impella 2.5 device (Abiomed Cardiovascular, Inc.) received FDA approval in 2008 to provide temporary LV support. The Impella 2.5 is inserted via the femoral artery, is advanced into the left ventricle in the catheterization laboratory, and provides up to 2.5 L/min of blood flow (Fig. 20.6). The Prospective Feasibility Trial Investigating the Use of the Impella 2.5 System in Patients Undergoing High-Risk Percutaneous Coronary Intervention (PROTECT I) showed the safety and feasibility of the Impella 2.5 in supporting patients with poor LV function who underwent PCI on an unprotected left main coronary artery or last patent coronary conduit [98]. In the PROTECT II trial, researchers compared the Impella 2.5 with the IABP for hemodynamic support while patients underwent high-risk PCI. The 30-day incidence of major adverse events, including death, MI, stroke, and repeat revascularization, was not different for patients supported with IABP or Impella 2.5. A trend toward improved outcomes was seen for Impella 2.5 patients at 90 days [99]. The usefulness of the Impella 2.5 in treating patients with AHFS and CAD remains unclear. Although there is a definite need for percutaneous mechanical support, selection of these devices depends on institutional availability, physician experience, and the specific clinical needs of the individual patient.
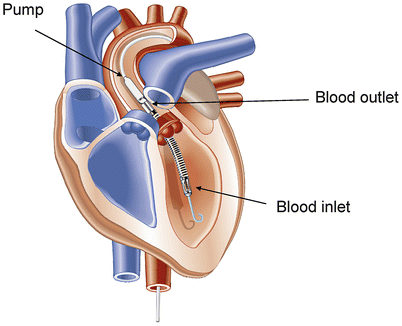
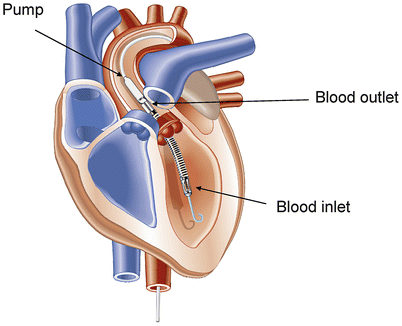
Fig. 20.6
The Impella 2.5 pumps blood from the left ventricle through an inlet area near the tip and expels blood from the catheter into the ascending aorta. The pump can be inserted by a standard catheterization procedure through the femoral artery to the ascending aorta and advanced across the aortic valve into the left ventricle (Courtesy of Abiomed, Inc.)
Treatment of Chronic Heart Failure
Heart failure is associated with a poor quality of life for patients because exercise intolerance and congestion are among its hallmark features. Heart failure is also highly fatal; its lethality exceeds those of all but the most aggressive malignancies. The goal of treatment, when HF is present, is relieving symptoms and prolonging life. It is important to note that HF is a progressive chronic disease and that if asymptomatic LV dysfunction is found, then treatment should begin when ventricular dysfunction is revealed, before symptoms develop.
Medical Therapy
Modern medical pharmacotherapy is aimed at establishing euvolemia and inhibiting the neurohormonal activation associated with the progression of HF. In some cases, “reverse remodeling” can occur with medical therapy, leading to reduced ventricular size, lessened valvular regurgitation, an improved EF, and stabilization of disease, which is associated with reduced readmissions to hospital.
Detailed guidelines for the medical management of HF have been produced by multiple North American, European, and other international committees [33, 100]. Generally, there is good agreement among the professional societies, and a polypharmacy approach has been endorsed by all professional societies.
Loop Diuretics
If signs or symptoms of congestion due to HF are present, then loop diuretics (Table 20.4) are an effective and often mandatory medication. Loop diuretics confer no mortality benefit but are highly effective in reducing elevated filling pressures and relieving pulmonary congestion and peripheral edema [101, 102]. Although loop diuretics are essential for the initial treatment of congestion, loop diuretics should be administered at the lowest dose that will achieve euvolemia. Higher doses of these diuretics are associated with increased mortality.
Table 20.4
Diuretics
Diuretic | Dose (mg) | Frequency | Duration (h) |
---|---|---|---|
Loop | |||
Furosemide | 2–200 | qd, bid, continuous | 6–8 |
Bumetanide | 1–4 | qd, bid, continuous | 2–4 |
Torsemide | 20–200 | qd | 6–8 |
Potassium–sparing | |||
Aldactone | 25–200 | qd, bid | 24 |
Eplerenone | 25–50 | qd, bid | 24 |
Other | |||
Metolazone | 2.5–10 | qd, bid | 24 |
Hydrochlorothiazide | 25–100 | qd | 6–12 |
Potassium-sparing diuretics should be considered a separate class of diuretic, because spironolactone has been associated with a survival benefit in NYHA class III or greater HF patients [103], and eplerenone [104] has been shown to exert a survival benefit in patients with ventricular dysfunction after MI. Patients who take these medications should be monitored at frequent intervals to ensure that they do not become hyperkalemic, and generally, these medications are contraindicated for or used with caution in patients with significant renal insufficiency.
Vasodilators
Vasodilator therapy is central to the treatment of HF patients with systolic dysfunction. Vasodilators are used to reduce arterial and venous filling pressures and to improve hemodynamics by reducing wedge pressure, reducing LV end-diastolic pressure, increasing cardiac output, and improving peripheral circulation. These hemodynamic improvements are often accompanied by improved symptomatology. Intravenous vasodilators are usually considered for patients with adequate blood pressure and ongoing congestion that is not sufficiently responsive to diuretics and standard oral therapy. Several agents are commonly used to treat acute heart failure and acute exacerbations of chronic heart failure [100]. The goal of such treatment is to relieve congestion and coronary ischemia and to improve hemodynamic variables until definitive therapy can be instituted.
Oral Vasodilators
Both ACE inhibitors and ARBs (Table 20.5) are used to inhibit the renin-angiotensin-aldosterone system, which plays an important role in vasoconstriction. Inhibiting this system promotes the excretion of sodium and water. The ACE inhibitors are vasodilating and act to reduce preload and afterload. More importantly, they are potent in averting adverse LV remodeling by preventing dilatation and hypertrophy. Long-term therapy with these agents improves symptomatology and exercise tolerance and reduces mortality risk [105–108]. These drugs are a cornerstone therapy that is recommended in all contemporary guidelines for chronic systolic HF therapy.
Table 20.5
Angiotensin-converting enzyme (ACE) inhibitors and angiotensin receptor blockers
Drug | Initial dosage | Target dosage | ||
---|---|---|---|---|
Dose (mg) | Frequency | Dose (mg) | Frequency | |
ACE inhibitors | ||||
Captopril | 12.5 | tid | 50–100 | tid |
Enalapril | 2.5 | bid | 10 | bid |
Lisinopril | 2.5–5 | qd | 10–20 | bid |
Quinapril | 5 | bid | 20 | bid |
Fosinopril | 10 | qd | 40 | qd |
Ramipril | 2.5 | bid | 5 | bid |
Trandolapril | 1 | qd | 2 | qd |
Angiotensin receptor blockers | ||||
Candesartan | 8 | qd | 32 | qd |
Losartan | 25 | qd | 50 | bid |
Valsartan | 80 | bid | 160 | bid |
The ARBs act as an alternative or supplementary approach to inhibiting the renin-angiotensin-aldosterone system by targeting the angiotensin I receptor, which mediates the physiologic effects of angiotensin, including vasoconstriction, cardiovascular tissue growth, and aldosterone stimulation. Recent studies have shown that these drugs are as effective as ACE inhibitors in retarding HF disease progression and improving exercise tolerance and mortality [109, 110]. Both ACE inhibitors and ARBs have potent renal effects and have the potential to produce hypotension, especially if patients are volume contracted. As target doses of these medications are approached, usually by up-titration over days or weeks, it is usually advisable to reduce diuretics as long as a stable volume can be achieved.
β-Blocker Therapy
Contrary to previous teaching that β-blocker therapy (Table 20.6) is potentially deleterious to patients with HF because it reduces heart rate and increases filling pressures, there is now clear and convincing evidence that long-term β-blockade has a substantial mortality benefit for patients with HF [111–118]. This benefit is mediated by a reduction in ischemia and cardiac death, as well as the inhibition of the adverse remodeling that is a hallmark of disease progression. Long-term β-blockade is associated with an improvement in EF and a reduction in end-diastolic and end-systolic LV volumes. Generally, β-blocker therapy is started at low doses, and the dose is up-titrated over several weeks. Patients should be counseled that they may experience fatigue and dyspnea for a few days after doses are increased, but that these symptoms are generally transient and that the medication is well tolerated by most patients, even those with pulmonary disease. The favorable long-term effects of β-blocker therapy extend across all HF patients regardless of whether their HF has an ischemic or nonischemic cause.
Table 20.6
β-blockers used to treat heart failure
Drug | Initial dosage | Target dosage | ||
---|---|---|---|---|
Dose (mg) | Frequency | Dose (mg) | Frequency | |
Carvedilol | 3.125 | bid | 25–50 | bid |
Metoprolol succinate | 12.5 | qd | 100–200 | qd |
Bisoprolol | 1.25 | qd | 10 | qd |
All patients with impaired ventricular dysfunction should be treated with both a β-blocker and an ACE inhibitor. If hypotension makes it impossible to reach target doses of both agents, then moderate doses of both agents should be used in combination, rather than employing the approach of forgoing one drug in favor of the other.
Other Agents
Isosorbide Dinitrate/Hydralazine
The combination of isosorbide dinitrate (ISDN) and hydralazine has a special place in the history of HF therapy. This was the first combination of medications that was shown in clinical trials to reduce mortality in HF patients [119]. The rationale for the trials was the improvement in hemodynamics (increased cardiac output and lowered filling pressures) that was previously demonstrated with this class of medications. Later trials showed the superior mortality benefits of ACE inhibitor therapy in contrast to ISDN/hydralazine, so the use of these drugs diminished for a period of time in favor of ACE inhibitors [105, 120]. When the results of the original Val-HeFT studies were subjected to subgroup analysis, it appeared that many of the favorable effects of ISDN and hydralazine were strongest in African Americans [121]. On the basis of findings from more recent studies [122, 123], some authors have speculated that nitrates function as nitric oxide donors and that hydralazine may act as a potent antioxidant that reduces NO consumption by reactive oxygen species. This would explain some of the short-term hemodynamic effects that were associated with nitrate administration in these studies, as well as the longer-term mortality benefits, which appeared to be independent of the hemodynamic effects. A confirmatory study of a fixed-dose combination of ISDN and hydralazine (Bidil; 20–37.5 mg) was conducted in African Americans already being treated with β-blockers and ACE inhibitors. This trial found a 43 % reduction in mortality in the group treated with ISDN and hydralazine. For this reason, the ACC/AHA [100] guidelines now recommend that all African-American HF patients receive this therapy [124].
Digitalis
The use of digitalis dates back to 1785, when the use of a medicinal preparation derived from the foxglove plant was first described in the English-language literature by Dr William Withering [125]. Since that time, digitalis, a plant-derived cardiac glycoside, has continued to be used, but not in the toxic doses that were used historically. The classical teaching is that digitalis’ main mechanism of action is positive inotropy, but there is also evidence that inhibition of neurohormones may be involved [126]. The slowing of the resting heart rate in patients with atrial fibrillation is another advantage of the drug; a significant number of patients with HF also have atrial fibrillation. Small trials showed that withdrawing digitalis therapy in stable HF patients could exacerbate their disease and worsen their HF symptoms [127, 128]. The largest trial of digitalis conducted in HF patients was the Digitalis Intervention Trial, which showed that digitalis, used in combination with contemporary HF therapy, did not confer a mortality benefit but stabilized disease to the extent that readmissions were reduced; however, there was a slight increase in sudden deaths in the digitalis-treated groups [129].
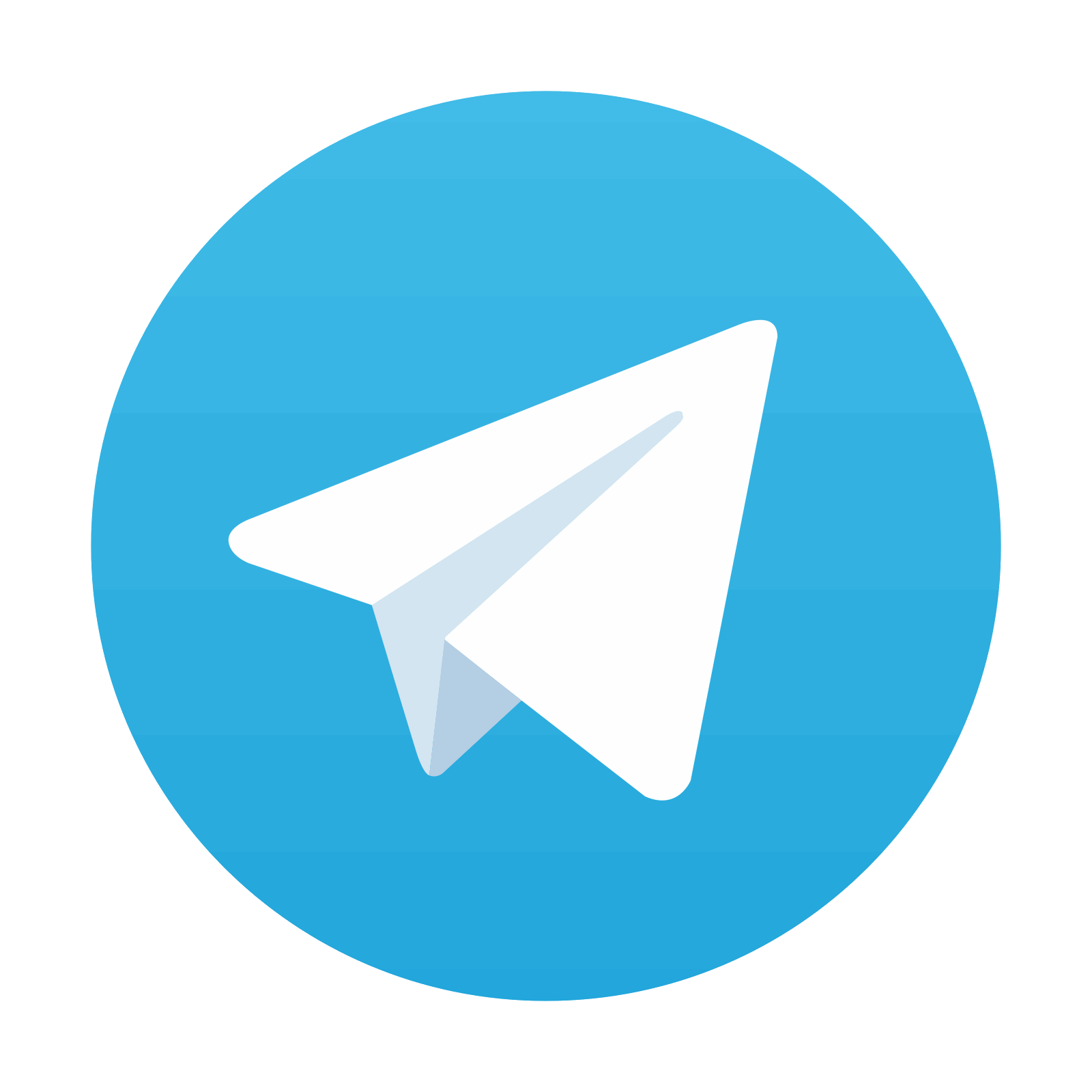
Stay updated, free articles. Join our Telegram channel

Full access? Get Clinical Tree
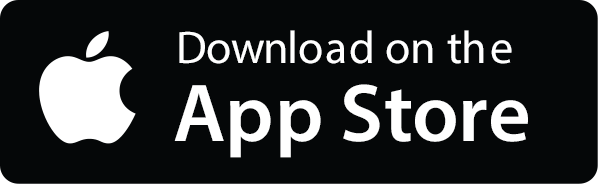
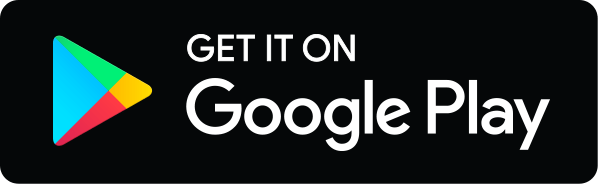
