Magnetic Resonance Imaging and Computed Tomography of the Vascular System: Introduction
Imaging of the vasculature has greatly evolved in the past 15 years. Although the current gold standard remains invasive x-ray angiography, noninvasive modalities such as magnetic resonance imaging (MRI) and computed tomography (CT) are becoming routine for the evaluation of patients with vascular diseases. In many instances, either MRI or multidetector computed tomography (MDCT) has replaced x-ray angiography as the imaging modality of choice in the assessment of patients with suspected vascular disease due to the ever-increasing quality of the images, the noninvasive application of these modalities, the ease and comfort of the patients, and the clinical versatility of both CT and MRI. In addition to evaluating the degree of luminal stenosis, MRI and CT can now detect noninvasively the presence and composition of atherosclerotic plaques in the different arterial beds. This chapter provides a comprehensive and state-of-the-art overview of the clinical indications for MRI and CT in the evaluation of vascular diseases.
Angiography
Magnetic resonance angiography (MRA) can be divided into two categories: nonenhanced and contrast-enhanced angiography.1 Nonenhanced MRA can be obtained either by detecting the effect of blood flow on signal amplitude (time of flight [TOF]) or on phase (phase contrast [PC]) of moving protons. TOF angiography relies on the differences in signal amplitude between in-slice stationary protons and blood protons flowing into the slice. In-slice stationary protons become relatively saturated with repeated excitation pulses and produce low signal intensity, whereas inflow blood protons in arteries and veins have not experienced the excitation pulses, are not saturated, and therefore generate high signal intensity. Limitations of TOF imaging are long acquisition times, the need to position sections orthogonal to the direction of flow, and saturation of protons, particularly in case of three-dimensional (3D) acquisition. Therefore, clinical applications of TOF imaging are restricted to the evaluation of extra- and intracranial arteries. PC angiography derives image contrast from the differences in the phases accumulated by stationary and moving spins in a magnetic field gradient. PC-MRA uses pairs of bipolar or flow-compensated and uncompensated gradient pulses to generate flow-sensitive phase images. Phase data can be used either to reconstruct velocity-encoded flow-quantification images or MRA images. With velocity-encoded imaging, amplitude of the phase is directly proportional to the flow velocity, allowing for quantitative measurements of flow velocities and the identification of flow direction. In addition to morphologic MRA, velocity-encoded imaging can help for the evaluation of flow and pressure gradients across stenoses in the carotid arteries, peripheral arteries, and renal arteries, as well as for thoracic dissection and coarctation. However, clinical applications are hampered by long acquisition times required for velocity-encoded imaging.
In contrast to nonenhanced MRA, first-pass MRA with gadolinium-based contrast agents has gained widespread acceptance owing to shorter acquisition times. The strong increase of luminal signal intensity after intravenous injection of T1-shortening contrast agents such as gadolinium chelates allows for fast angiographic acquisitions with 3D gradient echo sequences.2 These techniques have been proven to give an accurate evaluation of intra- and extracranial, thoracic, abdominal, and peripheral vessels. In many centers, contrast-enhanced 3D MRA has widely replaced conventional x-ray angiography for the evaluation of peripheral arterial vessels.3 Technical advances, such as synchronization of the arrival of contrast agent with magnetic resonance (MR) acquisition,4,5 moving bed technology for multistation studies,6,7 parallel imaging,8 and k-space sharing methods9 have dramatically reduced acquisition times and paved the way for whole-body MRA. Whole-body MRA is well suited for repeated clinical examinations in patients with systemic diseases such as vasculitis or atherosclerosis. Whole-body MRA (Fig. 24–1) has been shown to be feasible and accurate for simultaneous evaluation from carotid to lower limb arteries.11,12 Compared with invasive angiography, sensitivity and specificity of whole-body MRA for the detection of significant arterial stenosis were determined to be 96% for both, close to values reached with single-station MRA.11,13 However, intracranial and coronary artery imaging still requires dedicated acquisitions.
Figure 24–1.
Coronal 3D, T1-weighted, gradient recalled echo, fast low-angle shot, whole-body magnetic resonance angiograms obtained with a rolling table platform and five contiguous stations in a 31-year-old volunteer. The arterial signal is improved with venous compression. Modified from Herborn et al.10
Recent descriptions of associations between injection of gadolinium-based contrast agents and nephrogenic systemic fibrosis have stimulated the use of nonenhanced sequences for angiography, particularly for patients with preexisting renal insufficiency. Angiographies based on balanced steady-state free precession (SSFP) sequences are gaining acceptance for evaluating the thoracic aorta and coronary arteries. Image contrast with SSFP sequences stems from T2/T1 ratio, which is high in blood compared with other tissues. SSFP sequences produce bright blood imaging without reliance on blood inflow. Thanks to technical advances in hardware, the signal-to-noise ratio of MRAs increased with SSFP sequences, allowing for acquisitions with parallel imaging and shorter acquisition times. Both arteries and veins have high signal intensities with balanced SSFP sequences. Therefore, additional preparations, such as saturation bands or spin labeling, are required to null venous signal for angiographies of renal or carotid arteries with balanced SSFP.
In summary, recent technical advances in MRI technology, such as shortened acquisition times with parallel imaging and k-space sharing methods, have dramatically reduced acquisition times and allowed for larger spatial coverage. MR scanners at high field strength (≥3 teslas [T]) are now available in many radiology departments but have both advantages and drawbacks for angiographic studies. On one hand, intrinsic higher signal-to-noise ratio of tissues at 3 T offers to shorten scan time and improve spatial resolution, which is particularly beneficial for lower limb and coronary angiographies. On the other hand, increased susceptibility artifacts at higher field strength could alter the accuracy of contrast-enhanced and nonenhanced angiographies. Angiographic sequences with 3-T MRI scanners need still to be optimized before becoming clinical routine.
Single-spiral (ie, helical) CT became part of routine diagnostic imaging in the early 1990s and has matured into a volume-scanning modality. 3D reconstructions were obtained from continuous volume data acquired with spiral scanning and postprocessed using multiplanar reconstructions or volume-rendering techniques.14 However, in practice, the spiral data sets suffered from considerable differences between the transverse (in-plane) and longitudinal (out-of-plane) spatial resolutions. The advent of MDCT systems15 in the late 1990s have expanded the capabilities of CT in several ways, including scanning anatomic volumes with standard techniques with faster scan times, scanning larger volumes in shorter scan time, and scanning anatomic volumes with high longitudinal resolutions (narrow collimations) to obtain small isotropic voxels (0.35-0.4 mm3 using 64-MDCT scanners). In addition, continuous 3D data sets of any 5% intervals of the cardiac cycle can be obtained due to the increase in gantry rotation times of new MDCT systems (<330 ms) and “partial scans“ reconstruction algorithms. Besides cardiac applications, advantages of MDCT systems for assessing the vasculature in general are substantial. MDCT allows for a reduction of the injected volume of iodinated contrast, expanded volume coverage, increased longitudinal spatial resolution, and reduced pulsation artefacts compared with single-spiral CT. MDCT technology has substantially improved CT angiography (CTA) of cerebral, thoracic, abdominal, pulmonary, and peripheral vasculature.
Stroke represents the third leading cause of death in the United States, accounting for 600,000 cases each year. The risk of stroke associated with carotid stenosis has been evaluated in several trials. The North American Symptomatic Carotid Endarterectomy Trial (NASCET),16 the European Carotid Surgery Trial (ECST),17 and the Veterans Affairs Trial (VAT)18 demonstrated that carotid endarterectomy reduced recurrent stroke in symptomatic patients with severe stenosis (70%-99%) of ipsilateral carotid artery (see Chap. 110). Therefore, carotid endarterectomy is recommended for these patients if the morbidity of surgery is reasonably low (<6%). Carotid angioplasty represents an alternative attractive approach for patients with a high surgical risk. Symptomatic patients with moderate stenosis (50%-69% in NASCET) still benefited from surgery, although the overall gains were more modest than in patients with severe stenosis. For asymptomatic patients with severe carotid stenosis, carotid endarterectomy significantly reduced the 5-year stroke rate in selected subgroups with the highest risk.
In most of these trials, intra-arterial digital subtraction angiography (DSA) has been used to measure the degree of stenosis and, as such, remains the gold standard method against which alternative imaging modalities need to be validated. Concerns about the small but potentially severe risk of thromboembolic complications of DSA have prompted the use of noninvasive imaging such as Doppler ultrasonography, MRA, and CTA for the evaluation of suspected carotid artery stenosis.
MRA and CTA acquisitions can be obtained with short acquisition times and reconstructed in two-dimensional (2D) and 3D views with a high spatial resolution. Angiographies similar to conventional x-ray angiograms can be reconstructed using maximum-intensity projection (MIP) and rotated around 360 degrees. In addition to luminal angiography, the composition of the underlying carotid plaques can be studied more precisely with both imaging techniques and might help to define indications for intervention.
MRA has emerged as one of the noninvasive methods of choice for carotid imaging because it offers significant advantages over DSA. MRA does not require the injection of iodinated contrast agents or the use of ionizing radiation and is less sensitive to calcifications while still offering reconstructions of carotid angiographies in a format analogous to DSA. Multiple projections of the carotid arteries can be generated by postprocessing techniques such as MIP.
Before the advent of contrast-enhanced MRA (CE-MRA), 2D and 3D TOF acquisitions were used to image carotid arteries and have shown good agreement with DSA.19 However, TOF acquisitions are sensitive to turbulent flow and can overestimate the degree of stenosis. TOF sequences are also limited by long imaging times of 10 to 15 minutes, which increase susceptibility to motion artifacts. Despite these limitations, TOF MRA remains a useful option for patients who have contraindication to MR contrast agents, such as patients with renal insufficiency, and image quality can be excellent, particularly at high field strength. CE-MRA has become the technique of choice for imaging carotid arteries relying on injection of a paramagnetic agent such as gadolinium chelates to reduce the T1 relaxation time of tissue and to generate contrast between the intravascular lumen and surrounding tissues. Image acquisition needs to be synchronized with the arterial phase of the bolus to prevent venous enhancement. Unlike TOF MRA, vascular contrast is less sensitive to turbulent flow, particularly in case of high-grade stenosis. A large volume from the aortic arch to the circle of Willis (Fig. 24–2) can be obtained in <1 minute.21 CE-MRA demonstrated high sensitivities and specificities for the evaluation of carotid artery stenosis (Table 24–1) and is currently the most accurate noninvasive imaging for carotid stenosis. CE-MRA offers two to three times lower spatial resolution than DSA or CTA, but spatial resolution of CE-MRA is set to improve with new technologic developments, such as high field strengths, parallel imaging techniques, and the use of blood-pool contrast agents that remain in the circulation for a longer time and enable high-resolution steady-state angiographic acquisitions.
Figure 24–2.
Severe stenosis (arrows) of left internal carotid artery in a 72-year-old patient evidenced with contrast-enhanced magnetic resonance angiography (B) and 3D time-of-flight magnetic resonance angiography (C). Stenosis was not detectable with conventional digital subtraction angiography in this projection (A). Modified from Anzalone et al.20
References | Technique | Carotids (No.) | Sensitivity (%) | Specificity (%) |
---|---|---|---|---|
Anzalone et al, 200520 | MRA | 98 | 100 | 90 |
Fellner et al, 200523 | MRA | 21 | 100 | 96.7 |
Johnson et al, 200024 | MRA | 76 | 94 | 95 |
Serfaty et al, 200025 | MRA | 63 | 94 | 85 |
Sardanelli et al, 200022 | MRA | 56 | 100 | 100 |
Barlett et al, 200626 | CTA | 268 | 88.2 | 92.4 |
Anderson et al, 200027 | CTA | 80 | 77 | 92 |
Leclerc et al, 199928 | CTA | 44 | 100 | 97 |
Marcus et al, 199929 | CTA | 46 | 93 | 97 |
Verhoek et al, 199930 | CTA | 38 | 100 | 100 |
CTA of the carotid arteries requires the intravenous injection of an iodinated contrast agent. With the advent of multislice technology, the whole length of the carotid artery from the aortic arch to the circle of Willis can now be imaged in a true arterial phase, which limits adjacent venous enhancement (Fig. 24–3). Multislice CT enables volumetric acquisitions of carotid arteries with a high spatial resolution (voxel size of 0.3 mm3). Images similar to DSA projections and precise quantification of the degree of stenosis can be obtained after postprocessing of volumetric acquisitions. CTA demonstrated higher sensitivities and specificities than TOF MRA but lower than CE-MRA for the evaluation of the degree of carotid stenosis (see Table 24–1). Although spatial resolution is higher than for MRA, lower specificity of CTA can be explained by the difficulty in evaluating the degree of luminal stenosis in presence of extensive calcifications of carotid arteries. Among the limitations of CTA are the need to inject potentially nephrotoxic iodinated contrast agents and the ionizing radiations. In the future, development of k-edge imaging technology, which offers to discriminate between the absorption properties of iodine and calcium, could help to improve the quality of CTA of the carotid arteries.
Figure 24–3.
Contrast-enhanced 16-detector row computed tomography of the supra-aorta and intracranial vessels acquired in 12 seconds with a caudocranial scan range of 30 cm and 0.75-mm-thick slices. Maximum-intensity projection (A) and volume-rendering technique (B) both reveal a complex ulcerated atherosclerotic plaque of the proximal right internal carotid artery associated with a high-grade stenosis (arrows). Courtesy of B. Ertl-Wagner, Ludwig-Maximilians-University, Munich, Germany.
In summary, although the accuracy of noninvasive imaging techniques remains slightly lower than DSA for the evaluation of carotid stenosis, MRA and CTA are more readily accessible, and their accuracy is now considered to be sufficient to outweigh the risks associated with DSA. A combination of screening with Doppler ultrasound and confirmation with MRA or CTA is now clinical routine, with a role for DSA in case of discordance between noninvasive tests. CE-MRA is the most accurate noninvasive technique for the evaluation of the degree of carotid stenosis, but multislice CTA represents an attractive alternative imaging technique because of its availability and high spatial resolution.
Aortic dissection is a life-threatening disease in which early diagnosis and treatment is critical for survival32 (see Chap. 106). The yearly incidence of aortic dissection is 10 to 20 cases per million population. Misdiagnosis can have fatal consequences because untreated patients have an early mortality rate as high as 25% in 24 hours and 75% by 2 weeks.33 Retrograde aortography was the first accurate method for the diagnosis of aortic dissection. Diagnosis of aortic dissection by aortography requires the presence of an intimal flap and two distinct lumina. Aortography has a sensitivity of 88% and specificity of 94% for the detection of aortic dissection.34,35 Major drawbacks of this technique are the time required for the procedure and complications associated with invasive procedures. Recent technical advances in noninvasive imaging techniques have greatly improved early diagnosis and management of aortic dissection.
CTA is a fast, accurate alternative to transesophageal echocardiography because it is more readily available on a 24-hour basis. CTA has a sensitivity of 83% to 95% and a specificity of 87% to 100% for the diagnosis of acute dissection.36 CTA can visualize the intimal flap, branch vessel involvement, extent of dissection, patency of false lumen, size of aorta, presence of pericardial fluid, and some evidence of end-organ ischemia. If aortic disease is suspected, electrocardiogram (ECG) gating should be considered to limit aortic motion artifacts that can be present on CTA, in particular in patients with low heart rates.37 Initially, nonenhanced CT may need to be performed to rule out an intramural hematoma because intravenous contrast may obscure the diagnosis. Intramural hematoma appears as a crescent-shaped, high-attenuation signal within the wall of the aorta or, less often, as localized thickening of the aortic wall with internal displacement of intimal calcifications. Limitations of CTA for the evaluation of aortic dissections are the absence of hemodynamic information in case of valvular regurgitation and the need to inject potentially nephrotoxic contrast agents.
MRI is a safe and reliable alternative imaging technique for the diagnosis of aortic dissection. Acquisition times have been significantly shortened by implementation of faster and stronger gradients in recent MRI systems. The thoracic aorta and major aortic branch arteries can be imaged in a few seconds with 3D CE-MRA38,39 (Fig. 24–4) or in a few minutes using bright blood sequences such as steady-state gradient echo techniques that do not require the injection of contrast agent. As with invasive angiography, the diagnosis of aortic dissection is based on the presence of an intimal flap and two distinct aortic lumens. Velocity-encoding sequences can help to differentiate the true and false lumen and determine whether aortic side branches are perfused by the true or the false lumen. Black blood sequences offer high spatial and contrast resolutions and are well suited for the analysis of the aortic wall. Intramural aortic hematoma can be identified with MRI using black blood sequences as a crescentic aortic wall thickening (≥5 mm) without intimal flap or tear. MRI offers both anatomic and functional imaging of aortic dissections. Of all imaging techniques, MRI has the highest sensitivity and specificity for detecting aortic dissection. However, the use of MRI for the detection of aortic dissection is limited by its lack of availability in urgent situations and the difficulty to monitor and manage patients with unstable hemodynamic status in MRI scanners.
Figure 24–4.
Contrast-enhanced magnetic resonance angiograms of a 32-year-old patient suffering from Marfan syndrome. The oblique multiplanar reconstruction (MPR) shows an aneurysm of the aorta, extending from the thoracic to the abdominal aorta (TA = thoracic aorta, AA = abdominal aorta) with extensive elongation and kinking in the course of the complete descending aorta. A dissecting membrane extending from the aortic arch to the distal abdominal aorta (arrows) can be seen. Courtesy of A. Huber, Ludwig-Maximilians-University, Munich, Germany.40
Transesophageal echocardiography, CTA, and MRA have all demonstrated high accuracies for the diagnosis of aortic dissection.41 Because there is a clear time-dependent mortality rate in patients with aortic dissection who do not receive treatment, the first choice of an imaging technique should take into account the availability of the technique in emergency. Transesophageal echocardiography may be the preferred imaging modality if an experienced cardiologist is available because it provides immediate and sufficient information to determine whether emergency surgery will be required.
Thoracic aortic aneurysms (TAAs) are typically discovered in asymptomatic patients (see Chap. 106), with symptoms occurring in the setting of either a complication of the disease (ie, rupture or dissection) or when these complications are imminent. The most frequent etiologies of TAAs include degenerative aneurysms, bicuspid aortic valve, and aneurysms associated with connective tissue disease (eg, Marfan syndrome). The risk of complications depends mainly on TAA diameter, location, and etiology. Thresholds for preventive surgical interventions have been defined accordingly. When the diameter of the ascending aorta reaches >55 mm for degenerative aneurysm and >50 mm for aneurysm associated with bicuspid aortic valve or Marfan disease, surgery is recommended. These thresholds can be lowered in case of rapid expansion of TAA (>5 mm/y). Expression of aortic diameters indexed to body surface area should be preferred, in particular when body surface area is out of the normal range.
CTA and 3D CE-MRA perform equally well to depict the thoracic vascular anatomy, particularly the relationship of aortic aneurysm to branch vessel origin and the extent of aneurysm.42 Acquisitions should be performed in diastole and with breath-holding to limit motion artifacts in the ascending aorta. MDCT can combine noninvasive evaluation of coronary arteries and imaging of the thoracic aorta. 3D CE-MRA has demonstrated a diagnostic accuracy of 100% for assessing the size and extent of the aneurysm and its relationship to aortic branches, compared with conventional angiography and surgical exploration.43 CE-MRA should be completed by cross-sections of the aortic wall with black blood sequences to detect the presence of an intraluminal thrombus. In addition, the presence of inflammation, such as in mycotic aneurysm or aortitis, might be suspected when delayed enhancement is detected in the aortic wall and surrounding soft tissues. The aortic valve is frequently involved in ascending aorta aneurysm. Cine SSFP sequences in a plane along the axis of the aortic outflow tract can help to determine whether aortic stenosis and/or regurgitation are associated to aneurysm. Before intervention involving the descending aorta, care should be taken to locate the origin of the artery of Adamkiewicz with MRA to avoid spinal complications after surgery.44
The abdominal aorta is the most frequent arterial location of aneurysms, with abdominal aortic aneurysms (AAA) usually being defined as a maximal aortic diameter ≥30 mm. The prevalence of asymptomatic AAA in men and women over the age of 60 years is evaluated at 4% to 8% and 0.5% to 1.5%, respectively, and increases with age. AAAs share common risk factors with atherosclerosis except diabetes.4 At least 15,000 deaths are caused each year by ruptured AAAs in the United States. Once an AAA ruptures, mortality is high (80%-90%). In contrast, elective repair is associated with a low rate of complications (2%-3%). Screening strategies have therefore been developed to identify patients with large AAAs (>55 mm in diameter) who might benefit from elective repair. Ultrasonography remains the most cost-effective imaging technique for morphologic detection and follow-up of abdominal AAA expansion. Main indications for CTA and MRI are preoperative evaluation of AAA and follow-up of patients who have had endovascular grafts. Preoperative assessment of AAAs before open or endovascular repair should include the maximum transverse diameter; the relation of the AAA to the renal arteries; the length, diameter, and angulation of normal-caliber aorta below the renal arteries before the aneurysm (ie, infrarenal neck); the presence of iliac or hypogastric aneurysm; and serious occlusive disease in the iliac or renal arteries.
Contrast-enhanced CT (CTA) offers 2D and 3D high-resolution imaging of AAAs and represents the preferred imaging modality for preoperative evaluation of AAAs before open or endovascular surgery. MDCT provides a volumetric acquisition that allows for multiplanar and 3D rendering reconstructions to be generated perpendicular to the long axis of the aneurysm, resulting in greater accuracy of the aneurismal size measurements.45 Parameters such as the maximal transverse diameter of AAA, relation of branching arteries to the AAA, the length of nonaneurysmal aorta below the renal arteries, intraluminal thrombus, and calcifications and tortuosity of iliac arteries can be assessed precisely with CTA and will help select between open or endovascular repair of aneurysms. Juxta- and suprarenal extension of an AAA can be missed with x-ray angiography because of a mural thrombus or atheroma filling the proximal neck. CTA can identify concomitant renal artery stenoses (>50%) in presence of AAAs46 with a 94% sensitivity and 96% specificity. In addition, 3D image reconstruction allows for identification of the ostia of the mesenteric arteries. As with other indications, the limitations of CTA are the need for ionizing radiation and injection of iodinated contrast agent, which might be of added concern in cases of repeated imaging or renal insufficiency. In addition, extensive calcifications of the arterial wall can preclude measurements of the diameters of iliofemoral arteries before endovascular repair.
MRI represents an alternative to CTA for preoperative evaluation of AAA, particularly in cases of renal insufficiency or massive calcifications of arterial walls, although attention should be paid to the consideration of gadolinium agents in patients with severe renal dysfunction. Similar to CT, MRI provides 2D and 3D high-resolution anatomic imaging of AAA (Fig. 24–5). Angiography can be obtained either without injection of contrast agent with TOF sequences that require long acquisition times or, more rapidly, with fast gradient echo sequences associated with the injection of gadolinium chelates. Exact dimensions of the aneurysm can be determined with multiplanar reconstructions prior to intervention with a high accuracy48,49; extension of AAA into the iliac and renal arteries and the presence of concomitant stenosis of renal arteries can also be determined (see Fig. 24–5). 3D CE-MRA is usually completed by delayed axial acquisitions to evaluate the size of intraluminal thrombus.50
Figure 24–5.
A 79-year-old patient with infrarenal aortic abdominal aneurysm. Contrast-enhanced, three-dimensional, maximum-intensity projection, magnetic resonance angiographic image shows the abdominal aorta and its major branches. Modified from Atar et al.47
MRA is indicated for the preoperative evaluation of patients with lower limb ischemia. Similar to invasive angiography, MRA can depict the location and degree of stenosis of peripheral arteries (see Chap. 109 and 110). TOF angiography was the first technique used to evaluate peripheral arteries.51,52 Limitations of TOF imaging are long acquisition times, the need to position sections orthogonal to the direction of flow, and underestimation of retrograde collateral flow in occluded arteries. CE-MRA has supplanted TOF-MRA for lower extremity arterial imaging owing to shorter acquisition times (a few seconds). For CE-MRA, the beginning of the acquisition should be perfectly synchronized with the arrival of the bolus of contrast agent to avoid venous enhancement. Meta-analyses consistently demonstrated greater accuracy of CE-MRA compared with TOF-MRA, with a sensitivity of 96% to 98% and a specificity of 96% for the detection of significant stenoses with CE-MRA.53 CE-MRA was found to be superior to duplex ultrasound and highly accurate, with sensitivities of 81% to 100% and specificities of 83% to 99% compared with conventional angiography (Table 24–2). The high degree of agreement between MRA and conventional angiography has led to surgical planning based on MRA alone62 (Fig. 24–6). CE-MRA is also suitable for the evaluation of lower extremity bypass grafts and has been found to be 100% sensitive and specific in this setting.64 Technical advances in MRI such as continuously moving tables and parallel imaging54,65,66 increase substantially the field of view that can be imaged during the arterial phase of the bolus of contrast agent.
Figure 24–6.
A 67-year-old man suffering from intermittent claudication. Both contrast-enhanced magnetic resonance angiography (A) and digital subtraction angiography (B) show significant stenosis in the right common iliac artery (arrow) and in the right external iliac artery (arrowhead). Modified from De Vries et al.63
References | Technique | Patients (No.) | Sensitivity (%) | Specificity (%) |
---|---|---|---|---|
Hentsch et al, 200354 | MRA | 203 | 93 | 90 |
Ruehm et al, 200056 | MRA | 61 | 92 | 98 |
Huber et al, 200057 | MRA | 24 | 100 | 98 |
Sueyoshi et al, 199958 | MRA | 23 | 97 | 99 |
Willmann et al, 200559 | MDCT (16) | 39 | 96 | 97 |
Martin et al, 200355 | MDCTA (4) | 41 | 98 | 97 |
Ofer et al, 200360 | MDCTA (4) | 18 | 91 | 92 |
Riecker et al, 199761 | SCTA | 30 | 93 | 99 |
Early detection of renovascular disease has been previously hampered by the lack of an adequate noninvasive diagnostic modality. CTA and CE-MRA were found to be significantly more accurate than ultrasound scanning or captopril renal scintigraphy for diagnosis of renal artery stenosis.67 Sensitivity and specificity values for the diagnosis of renal artery stenosis with CE-MRA range from 82% to 100% and 64% to 100%, respectively. In addition, PC-MRA also provides functional information such as velocity profile of renal arterial flow.68 TOF-MRA has been validated for imaging of the proximal portions of splanchnic vessels,69 but it is technically limited by long scan times and flow-dependent signal loss from in-plane and turbulent flow. CE-MRA provides greater spatial resolution with higher signal-to-noise ratio. Sensitivity and specificity rates for the visualization of the celiac and superior mesenteric arteries and their major branches have been determined to be between 94% and 100%.49,70
The diagnostic accuracy of CTA for the evaluation of peripheral vessels compares well with MRA, although studies directly comparing these two imaging modalities are lacking. Shorter acquisition times, thinner slices, higher spatial resolution, and MDCT scanners have enabled scanning of the whole vascular tree in a limited period with injection of small volumes of contrast agent. High-quality multiplanar reformations and 3D renderings can be processed easily and quickly from CTA acquisitions. Main clinical indications of CTA for imaging the lower extremities include the evaluation of peripheral, arterial, and aneurismal diseases; patency and integrity of bypass grafts; and traumatic arterial injury.71,72 Recent studies using CTA report sensitivity and specificity rates of approximately 98% for the detection of stenosis and >50% for the detection of peripheral artery disease73,74 (see Table 42–2). CTA seems even to be superior to conventional angiography for the evaluation of calf vessels of patients with proximal occlusions.55 Several clinical studies demonstrated a high reproducibility of CTA measurements. However, CTA is less accurate in presence of severe and diffuse calcifications of the arterial wall.75 In addition, care should be taken to limit patient exposure to ionizing radiation. In the first clinical study on peripheral CTA, the calculated radiation dose was however 3.9 times lower than that with x-ray angiography. Increased number of detectors in recent systems should continue to help to lower the radiation exposure. For the evaluation of renal and mesenteric arterial stenoses, diagnostic accuracy of CTA is similar to the results obtained with MRA (Fig. 24–7).67
Figure 24–7.
Computed tomography angiography of the peripheral vasculature of a 74-year-old man was acquired in <40 seconds with 0.75-mm-thick slices using a 16-detector multidetector computed tomography scanner. For optimal visualization of the peripheral vessels with maximum-intensity projections (A), bone structures have to be excluded manually. Although significant calcifications are present in the abdominal aorta (arrow), patent arteries can be depicted until the ankle joints on both sides. 3D reconstructions with volume rendering (B, C) can be easily obtained and rotated in different views for analysis of peripheral vessels without the need to exclude bone structures. Courtesy of B. J. Wintersperger, Ludwig-Maximilians-University, Munich, Germany.76
Pulmonary x-ray angiography remains the gold standard for the diagnosis of pulmonary embolism (PE) but is invasive, can give false-positive results, and is not readily available in many centers.77 In addition, conventional x-ray angiography is associated with a 1% risk of major complications from the procedure and a mortality of 0.5%.78 To reduce the need for pulmonary angiography, different tests are available for diagnosis of PE, including clinical probability scores,79 plasma D-dimer concentration, lung scintigraphy (ventilation/perfusion), and venous compression ultrasonography of the legs (see Ch. 109). In the last decade, MRA and especially spiral CTA have become attractive alternatives to conventional angiography in the diagnosis of both acute and chronic PE.80 Nevertheless, x-ray angiography can still be required in patients for whom the diagnosis of PE remains uncertain after different noninvasive diagnostic tests.77
Conventional x-ray angiography has, in most institutions, been replaced by spiral CT, which is more readily available and yields lower procedure-associated risks. The sensitivity of spiral CT is on the order of 90% for central, lobar, or segmental PE (Table 24–3). The advent of MDCT provides the ability to analyze subsegmental pulmonary arteries, even though subsegmental PE is of uncertain clinical significance.91 CTA (Fig. 24–8) has been proven to be an accurate, safe, noninvasive, easily and rapidly performed, widely accepted, and cost-effective technique for direct detection and demonstration of intraluminal PE.92 Follow-up studies have demonstrated that CT pulmonary angiography can be used in combination with investigation for deep vein thrombosis to exclude PE.93 CT venography of the pelvis and lower extremities can be incorporated into the CT protocol to identify or exclude concurrent deep venous thrombosis at the expense of an increased radiation burden94 and minimal clinical benefits.95 Now, spiral CT pulmonary angiography should be considered as the first choice of imaging technique for the diagnosis of PE, particularly in patients with high rates of nondiagnostic ventilation/perfusion scintigraphy scans, such as hospitalized patients, patients with a history of cardiopulmonary disease, or patients with abnormal chest x-rays. A diagnostic strategy based on D-dimer testing and MDCT may be able to rule out PE without the need of lower-limb ultrasonography.96
Figure 24–8.
Pulmonary computed tomography angiography (CTA) acquired with a 16-detector CT scanner in a 61-year-old woman with a high clinical probability of pulmonary embolism. CTA reveals large thromboembolic clots (arrows) on both sides confirming a massive pulmonary embolism. Courtesy of G. Le Gal, University Hospital, Brest, France.
References | Technique | Patients (No.) | Sensitivity (%) | Specificity (%) |
---|---|---|---|---|
Pleszewski et al, 200681 | MRA | 48 | 82 | 100 |
Ohno et al, 200482 | MRA | 48 | 83 | 97 |
Oudkerk et al, 200283 | MRA | 118 | 77 | 98 |
Gupta et al, 199984 | MRA | 36 | 85 | 96 |
Meaney et al, 199785 | MRA | 30 | 100 | 95 |
Stein et al, 200686 | CTA | 824 | 83 | 96 |
Stone et al, 200387 | CTA | 25 | 57 | 94 |
Perrier et al, 200188 | CTA | 299 | 70 | 91 |
Blachere et al, 200089 | CTA | 179 | 94 | 95 |
Remy-Jardin et al, 200090 | CTA | 370 | 96 | 100 |
MRA of pulmonary arteries can be acquired with several techniques such as TOF angiography and 3D MRA with injection of gadolinium chelates or blood-pool contrast agents.97 Large pulmonary arteries can be imaged using TOF sequences,98,99 but TOF-MRA is limited by a lack of spatial resolution, artifacts related to slow flow, motion, and field distortion. 3D CE-MRA can now be acquired in a single breath-hold (Fig. 24–9) due to the development of short time-resolved 3D gradient echo sequences and parallel acquisition techniques. In addition, MR sequences have been developed to allow for the evaluation of lung ventilation in addition to perfusion sequences.100,101 A number of clinical studies have reported high sensitivities and specificities for the detection of PE compared with conventional pulmonary angiography (see Table 24–3). A combined “one-stop shopping“ MRI approach for PE and deep venous thrombosis seems to be feasible and accurate.102 Pulmonary MRA can also be of interest to differentiate chronic thromboembolic pulmonary arterial hypertension from primary pulmonary hypertension.103,104 Pulmonary MRA represents an alternative imaging technique to CTA but, due to the accuracy and availability of CTA, clinical indications for pulmonary MRA remain infrequent.
Figure 24–9.
3D, contrast-enhanced, high-resolution magnetic resonance angiography (MRA) of the pulmonary vasculature in a 55-year-old patient (A, B) revealing large thromboembolic clots in the central pulmonary artery tree on both sides (arrows). Using time-resolved MRA perfusion techniques and acquiring one data set every 1.1 seconds, significant perfusion defects in the upper left and right lower lobe become visible (C,arrowheads). Courtesy of K. Nikolaou, Ludwig-Maximilians-University, Munich, Germany.
The small size and constant cardiac motion of coronary arteries offer a challenge to their noninvasive diagnostic imaging. So far, no cross-sectional imaging modality has proven to be capable of depicting the coronary arteries with a sufficiently high temporal and spatial resolution for a consistent and adequate image quality as good as that of x-ray angiography. Spatial and temporal resolutions of noninvasive imaging modalities such as MRI and MDCT systems are constantly improving thanks to recent technical innovations. In most of the early reports, results were quite impressive in a limited number of patients with optimal imaging conditions, but often, only proximal segments of the vessels were analyzed, and many patients (ranging from 5% to 30%) were excluded from final analysis because of poor image quality.105,106 The newer generation of CT scanners (64 or more detector rows) represents a significant breakthrough for coronary imaging with temporal resolutions of <80 ms and 0.35-mm isotropic voxel spatial resolution and has outperformed both electron-beam CT and MRI. CT systems with 64 or more detector rows seem able to produce consistent, high-quality coronary angiographies in most patients. Concerns remain about radiation burden, especially the cumulative exposure, using these systems.
Despite the impressive image quality that can be achieved in some patients with optimal imaging conditions, CT is not yet ready to routinely replace invasive coronary angiography for the diagnosis of coronary artery stenoses in the majority of patients. Only the recent substantial developments in scanner technology have made it possible to perform adequate coronary CTA.107
Increasing the number of detectors in new CT systems results in the ability to acquire a larger volume during a gantry rotation and to shorten the acquisition time. Modern MDCT systems have an x-ray gantry rotation time of as fast as 370 ms. In addition, reconstruction algorithms have been developed that permit the use of data acquired during either little more than one half of one rotation (ie, half-scan algorithms) or smaller sections of several subsequent rotations (ie, multiphase algorithms) for image reconstruction.108 Using half-scan reconstruction algorithms, image acquisition windows of approximately 185 to 250 ms can be achieved without the necessity of averaging data acquired over more than one heartbeat. In addition, CT acquisitions are gated to the ECG and reconstructed usually during end-diastole (phases 70%-80% of R-R) when the heart motion is the lowest. The heart can now be depicted in a breath-hold time of <5 seconds with a 128-detector CT scanner compared with a 35- to 40-second breath-hold with previous 4-detector CT scanners. Oral or intravenous β-blockade is recommended for patients with heart rates >65 beats/min with 64-detector CT or less to ensure motion-free images. In addition to reducing motion artifacts, shortening acquisition times also limits the volume of contrast agent required for optimal enhancement of coronary arteries109 (from 120 to 160 mL for 4-detector CT to 70 mL for 64-detector CT). Coronary MDCT acquisitions with a 64-detector CT scanner allows for volumetric reconstructions with a spatial resolution of 0.33 mm. New CT systems are based either on larger simultaneous acquisition volume by increasing the number of detectors or faster temporal resolutions with so-called dual-source CT that combines two x-ray tubes and detectors arranged at an angle of 90 degrees in a single gantry. Increasing the number of detectors up to 256 allows the CT to cover the entire heart in one single rotation, while spatial and temporal resolution remain unchanged.110 This approach makes cardiac CT imaging less susceptible to arrhythmias, requires less contrast, and will potentially reduce radiation dose.111 However, dual-source CT112 can collect the x-ray data necessary for 3D reconstructions in only one-quarter rotation (temporal resolution of 83 ms). This technology helps to reduce motion artifacts and diagnostic accuracy of coronary CTA in patients with heart rates >65 beats/min.113 A strong concern for MDCT is radiation burden, with an estimated dose of up to 11 to 21 mSv with the first MDCT systems.114-116 Zanzonico et al117
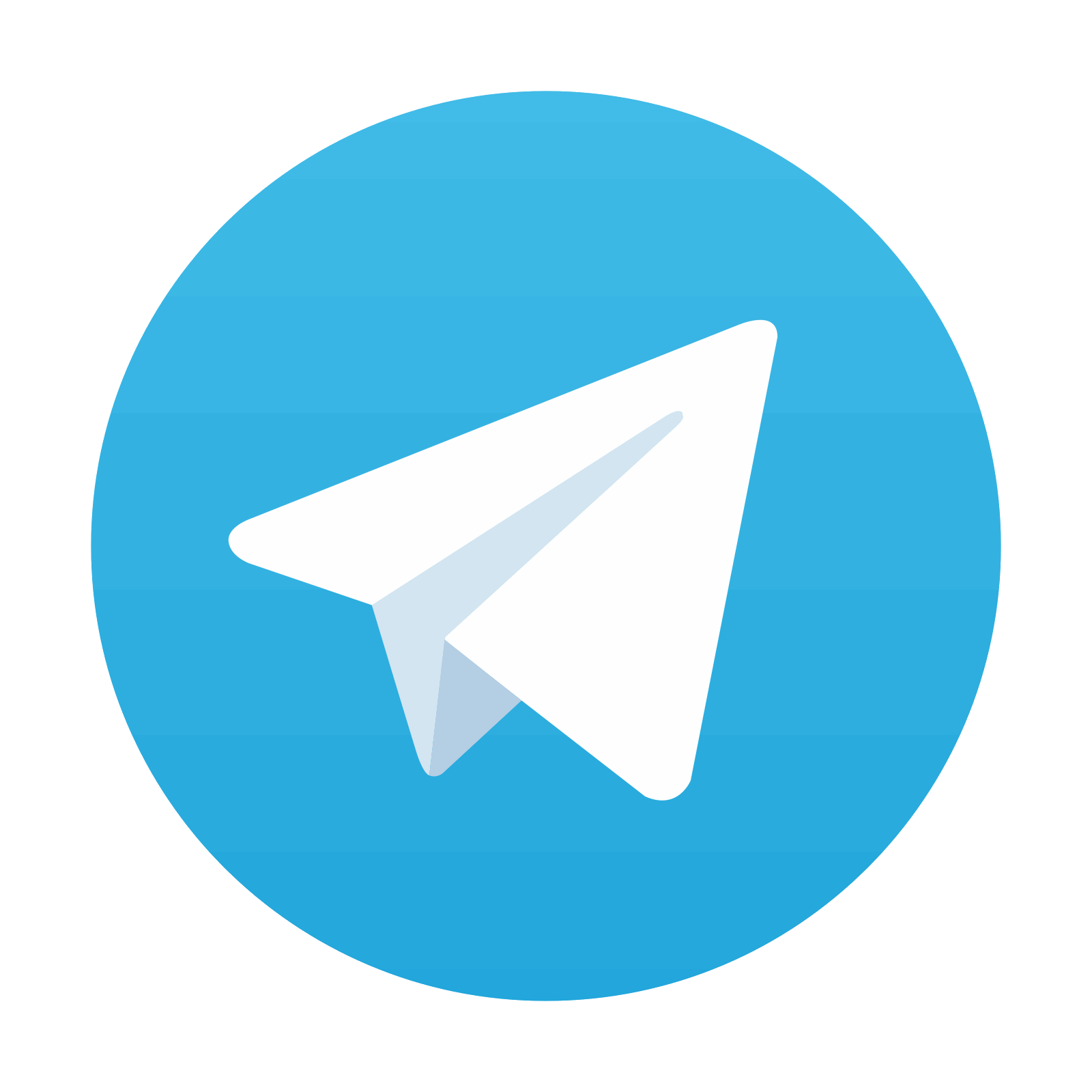
Stay updated, free articles. Join our Telegram channel

Full access? Get Clinical Tree
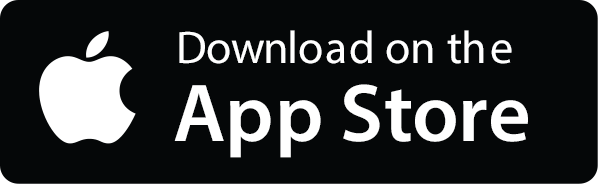
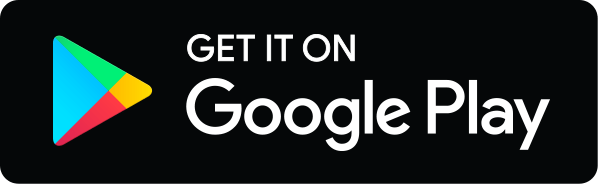
