Fig. 5.1.
India ink stain of C. neoformans var. grubii strain H99, revealing the characteristic polysaccharide capsule surrounding the fungal cells as a white halo. Note also that a number of the cells in the image are in the process of budding to produce new daughter cells
C. neoformans is primarily an environmental organism, which is widespread in soil, bird guano and rotting wood (Kronstad et al. 2011). C. neoformans can reproduce asexually, via budding, or sexually via a teleomorph form. The sexual (teleomorph) state is called Filobasidiella neoformans and forms in response to certain environmental and nutritional conditions. Sexual reproduction occurs between two distinct mating types, a and α, producing basidiospores, which may be the main infective vectors in cryptococcosis (Giles et al. 2009; Hull and Heitman 2002).
II. The Pathogenesis of Cryptococcosis
Cryptococcal infection in the lungs begins following inhalation of infectious cells or spores (Giles et al. 2009). There are no documented cases of human-to-human spread of cryptococcosis and thus infections are thought to result almost exclusively from environmental exposure. Following inhalation, C. neoformans initially colonises the extracellular alveolar space, where it comes into contact with the host innate immune system. As part of the innate immune response, alveolar macrophages migrate to the site of infection and attempt to clear the infection by engulfing fungal cells via phagocytosis (see Fig. 5.2). Following phagocytosis, however, C. neoformans is able to survive and grow within the intracellular niche provided by the macrophage. Initial respiratory infection occurs in both healthy and immunocompromised individuals, but subsequent disseminated infection is far more likely if an immune deficiency is present.
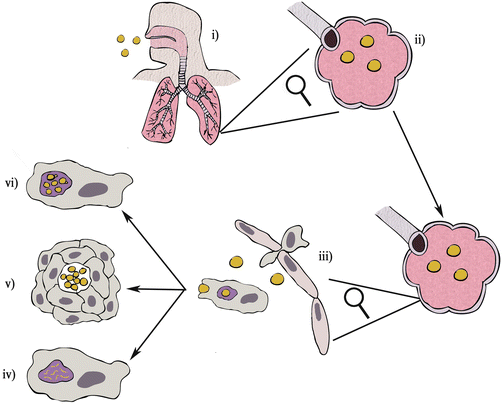
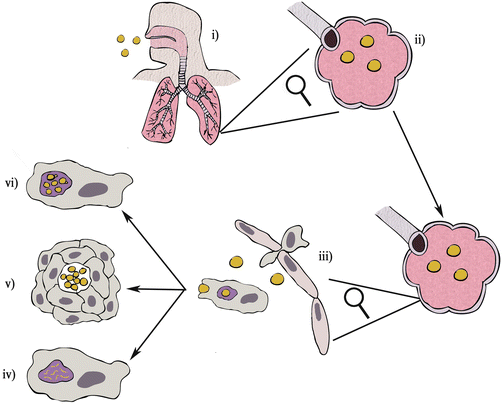
Fig. 5.2.
Cryptococcosis pathogenesis. (i) Desiccated C. neoformans cells or infectious spores are breathed into the respiratory tract following environmental contact. (ii) The fungi move down the respiratory tract until they reach the alveoli where they initially grow extracellulary. (iii) Immune phagocytes such as macrophages are attracted to the site of infection and proceed to engulf extracellular fungi via phagocytosis. Following phagocytosis there are three possible outcomes for fungi in the macrophage: (iv) the macrophages succeeds in killing the invader and the fungus is destroyed; (v) the macrophage cannot kill the fungus but is able to keep it contained within a granuloma in a dormant state; or (vi) the macrophage cannot control fungal growth and the fungi begin to replicate within the macrophage, escape from the macrophage and may disseminate to the central nervous system
Disease outcome following C. neoformans infection depends on the strength of the host immune response. Broadly there are three possible outcomes:
1.
Fungal cells in the alveolar space are phagocytosed by alveolar macrophages and infiltrating neutrophils; following phagocytosis the fungi are killed, resulting in total clearance of infection.
2.
Fungal cells are phagocytosed, but not destroyed, by alveolar macrophages. The fungi reproduce in alveolar macrophages, eventually disseminating to the CNS where they cause fatal meningoencephalitis.
3.
Fungal cells in the alveolar space are phagocytosed by alveolar macrophages; the immune system is unable to fully clear infection but manages to contain the fungi within the lungs in a latent state within granulomatous structures.
For C. neoformans, fatal disseminative disease (ii) occurs almost exclusively in individuals with pre-existing immune deficiencies whereas total clearance (i) and latent disease (iii) can manifest in individuals who are immune competent.
Epidemiological studies can never truly determine the extent of C. neoformans infection within immune competent populations because the majority of infections are asymptomatic and go unreported. To address this issue, studies have used anti-cryptococcal immunoglobulin titres within immune competent populations as a sign of past infection. These studies conclude that cryptococcal infection is common in the normal adult population (Deshaw and Pirofski 1995; Houpt et al. 1994) and most individuals are probably exposed to the fungus during childhood (Abadi and Pirofski 1999; Houpt et al. 1994). These conclusions seem reasonable considering the ubiquity of C. neoformans within the natural environment, thus it is likely that individuals encounter C. neoformans many times during their lifetime but are protected by a healthy immune system.
Some studies show that latent infection contained by the immune system may re-emerge if an immune deficiency later develops. Evidence for this phenomenon comes from a study by Garcia-Hermoso et al., which determined the genetic origin of clinical C. neoformans isolates taken from a cohort of cryptococcosis sufferers in France. This analysis found that a number of immunocompromised African immigrants in the study, who had lived in France for many years before developing immune deficiency, were infected with C. neoformans strains originating in Africa. Garcia-Hermoso et al. concluded that these patients may have developed a latent form of cryptococcosis before moving to France and that the infection re-emerged when immune deficiency later developed (Garcia-Hermoso et al. 1999).
III. The Macrophage
Macrophages are innate immune phagocytic cells that patrol the body’s tissues searching for signs of infection. When a macrophage detects a foreign cell, such as a pathogenic microorganism, it becomes activated and will attempt to engulf the invader via a process called phagocytosis (see Fig. 5.3). Phagocytosis begins when phagocytic receptors on the cell surface of the macrophage encounter ligands that are only found on foreign cells. Following receptor ligation, signalling pathways are activated inside the macrophage, which trigger cytoskeletal actin rearrangements around the receptor, leading to internalisation of the particle into a membrane-bound compartment termed the phagosome. Over time, conditions within the phagosome are altered by the macrophage to create an environment that is destructive to microorganisms, a process called phagosomal maturation. Via a series of vesicle fusion events, membrane proteins such as NADPH oxidase, V-ATPase and NOS are delivered to the phagosome. Together, these create a highly antimicrobial environment, for instance via the production of reactive oxygen species (ROS) by the NADPH oxidase, or lowering of the phagosomal pH by the V-ATPase. Finally, the phagosome fuses with a specialised cytosolic vesicle called the lysosome, which contains an array of digestive enzymes. These digestive enzymes, which work best at low pH, kill any remaining organisms in the phagosome and then break up the dead cell into its constituent parts.
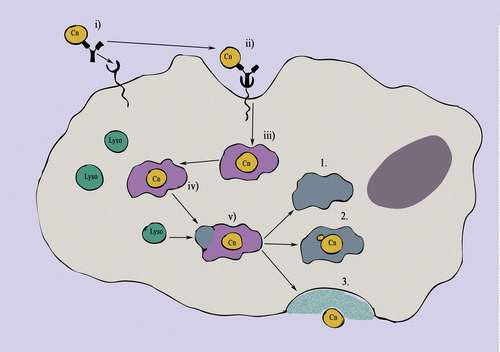
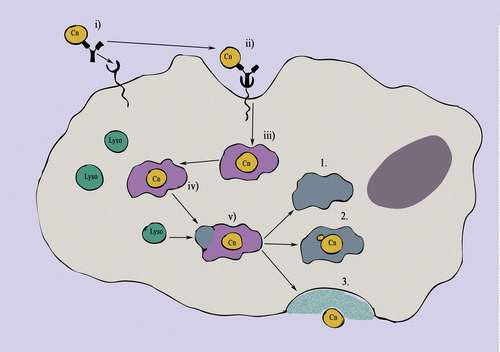
Fig. 5.3.
Possible outcomes following C. neoformans phagocytosis. (i) Opsonised C. neoformans (in this case opsonised with antibody) binds to phagocytic receptors on the cell surface of the macrophage. (ii) Ligation of phagocytic receptors induces cytoskeletal rearrangements within the macrophage resulting in invagination of the membrane and the formation of phagocytic pseudopodia around the bound cell. (iii) C. neoformans is contained inside the macrophage within a membrane-bound compartment called the phagosome. (iv) Over time the phagosome matures, beginning with the recruitment of v-ATPase, NADPH oxidase and NOS to the phagosome membrane, resulting in the lowering of pH and the production of reactive oxygen species within the phagosome. (v) Phagosomal maturation culminates when lysosomes fuse with the phagosome; this releases digestive proteolytic enzymes into the phagosome (which is now termed the phagolysosome). There are three possible outcomes for C. neoformans following formation of the phagosome: (1) Biocidal conditions within the phagosome destroy the fungus, which is then digested; (2) C. neoformans resists the conditions within the phagolysosome and begins to replicate; or (3) C. neoformans escapes from the macrophage via a process called vomocytosis (either following, or independently of, replication)
Phagocytosis can clear infection directly but it also triggers signals that enhance the immune response. Upon sensing infection, macrophages can release proinflammatory cytokines such as tumour necrosis factor (TNF)-α and interleukin (IL)-1β; the inflammation that these cytokines produce attracts other immune cells to the site of infection, ultimately engaging the adaptive immune system if the infection is not cleared rapidly.
IV. Phagocytosis of C. neoformans by Macrophages
Macrophages must detect the presence of foreign organisms before they can initiate phagocytosis. To facilitate detection, macrophages express an array of cell surface phagocytic receptors that bind to ligands present on foreign cells but not host cells. Phagocytic receptors can be categorised according to the nature of their ligands. Non-opsonic phagocytic receptors or pathogen recognition receptors (PRRs) bind to molecular ligands (pathogen-associated molecular patterns or PAMPs) such as polysaccharide residues or lipid species found on the surface of foreign cells. In contrast, opsonic receptors bind to opsonising proteins, such as antibodies or complement proteins, which coat foreign cells following activation of the humoral immune system.
Capsule expression has long been implicated in cryptococcal virulence since it was observed that Cryptococcus acapsular mutant strains are avirulent (Fromtling et al. 1982; Chang and Kwon-Chung 1994). The capsule has a number of properties that contribute to virulence during infection, but the most important seems to be its anti-phagocytic quality, which was first realised when acapsular strains were found to be phagocytosed by macrophages more readily than were capsular strains (Kozel and Gotschlich 1982; Cross and Bancroft 1995). Capsule growth can be stimulated by CO2 levels and pH conditions similar to those found during host infection (Granger et al. 1985) and has also been observed to occur in vivo (Feldmesser et al. 2001). Synthesis of the capsule is controlled by at least four genes, designated CAP64, CAP59, CAP10 and CAP60 (Buchanan and Murphy 1998; Ma and May 2009). In a series of experiments, Chang et al. found that deletion of each CAP gene produced an acapsular mutant that lacked virulence until the gene was reconstituted (Chang et al. 1996; Chang and Kwon-Chung 1994, 1999, 1998). The capsule is composed of two key polysaccharide components: glucuronoxylomannan (GXM) and galactoxymannan (GalXM) (Bose et al. 2003), with a ratio of approximately 9:1 by mass in favour of GXM (Idnurm et al. 2005).
One of the main ways that the capsule protects C. neoformans against phagocytosis is by providing a shroud around the cell, which interferes with macrophage detection. This shrouding effect blocks uptake via both opsonic and non-opsonic phagocytic receptors although it appears to have a greater inhibitory effect on non-opsonic uptake because non-opsonised C. neoformans cells are almost completely protected from phagocytosis (Mukherjee et al. 1996).
A. Non-opsonic Uptake
Phagocytosis of yeast-like fungi that do not produce a polysaccharide capsule, such as Candida albicans and Saccharomyces cerevisiae, often occurs via non-opsonic phagocytic receptors such as Dectin-1 and the Mannose receptor (MR) (Gantner et al. 2005; Brown and Gordon 2001; Porcaro et al. 2003; Giaimis et al. 1993). Both phagocytic receptors bind to cell wall constituents found in the fungal cell wall; Dectin-1 binds to β-1,3- and β-1,6-linked glucan residues (Brown and Gordon 2003) whereas MR binds to mannoproteins. Macrophages can easily phagocytose acapsular C. neoformans mutants via Dectin-1 or MR ligation, but they struggle to phagocytose encapsulated cells (Cross and Bancroft 1995). In wild-type capsular strains, the ligands for each receptor are obscured by the overlying capsule, thus it is thought that non-opsonic phagocytic receptors such as Dectin-1 and MR may contribute to the phagocytosis of recently inhaled cryptococcal cells (which have a minimal capsule), but once capsule growth is stimulated non-opsonic uptake becomes redundant.
B. Opsonic Uptake
Under most conditions, the cryptococcal capsule conceals cell wall ligands detected by non-opsonic receptors so the main route of phagocytosis by macrophages is via opsonic phagocytic receptors (Mukherjee et al. 1996). Opsonisation is more effective at facilitating the detection and phagocytosis of C. neoformans because opsonins bind to capsular components and thus are more accessible to macrophage phagocytic receptors than the non-opsonic receptor ligands. Two main classes of opsonic phagocytic receptors are expressed by macrophages: complement receptors, which recognise activated complement proteins, and Fc receptors, which recognise the Fc regions of immunoglobulin (antibody) molecules.
Complement can be activated via two pathways – the classical pathway and the alternative pathway. Classical activation requires the presence of antigen-specific immunoglobulin bound to the surface of the foreign cell, whereas alternative activation does not require antigen specificity. Antibody produced during cryptococcal infection is thought to be ineffective at activating classical complement cascades (Houpt et al. 1994) and thus the alternative complement cascade is considered more important to opsonisation during infection. Regardless of the route of activation, the end result of the complement cascade is the deposition of the complement fragment C3b, which can be recognised and phagocytosed by the macrophage complement receptor CR3.
Although complement deposition facilitates macrophage detection of C. neoformans, the pathogen is still able to reduce the efficiency of complement-mediated uptake by modifying the makeup of its capsule. Consequently, cryptococcal cells producing a capsule that is thick (Zaragoza et al. 2003) or has a low density (Gates and Kozel 2006) are harder for macrophages to detect, since both of these properties increase the likelihood that complement proteins will bind within the capsule, where they are obscured, as opposed to at the surface.
The production of antigen-specific antibody against a newly encountered pathogen requires activation of the adaptive immune response, although secondary exposure to an antigen evokes a quicker response due to the persistence of immunological memory. Antibodies can improve the phagocytic uptake of foreign cells in two ways once they have bound to the cell. They can amplify complement opsonisation by activating the classical complement pathway, but they can also directly stimulate phagocytosis via ligation of macrophage Fc receptors, which bind to the non-variable region of antigen-bound antibodies. During C. neoformans infection, most antibody produced is against capsular components such as GXM. Anti-capsular GXM antibody titres are often used as markers of acquired immunity to C. neoformans in healthy individuals (Deshaw and Pirofski 1995; Houpt et al. 1994); however, the protective role of anti-GXM antibodies during infection is debateable. For example, a 1994 study by Houpt et al. found that the sera of healthy subjects contained IgG and IgM antibodies reactive against cryptotoccal GXM, but that these antibodies were ineffective at activating the classical complement cascade (Houpt et al. 1994).
C. Capsule-Independent Antiphagocytic Factors
The capsule is a major antiphagocytic mechanism deployed by C. neoformans, but capsule-independent antiphagocytic mechanisms also exist. A study by Liu et al. identified a GATA family transcription factor called Gat201, which appears to control such a mechanism (Liu et al. 2008). A recent study of Gat201 revealed that knockout of its gene affected the transcription of ~1,100 genes in C. neoformans, although only 62 of these genes are thought to be regulated by direct binding of Gat201. Phenotypic analysis of the GAT201 knockout strain showed reduced capsule size and increased uptake by macrophages. The susceptibility to phagocytosis observed was greater than that of acapsular mutants alone, suggesting that capsule-independent antiphagocytic mechanisms are also controlled by Gat201 potentially via two downstream genes – BLP1 and GAT204 (Chun et al. 2011).
One capsule-independent antiphagocytic mechanism is the secreted protein APP1. This protein is expressed by C. neoformans during infection. APP1 can block complement-dependent phagocytosis by binding to and subsequently blocking macrophage CR3 receptors (Luberto et al. 2003).
D. Titan Cell Formation
Several studies have reported the presence of abnormally large cryptococcal cells in the lungs during in vivo infection (Zaragoza et al. 2010; Cruickshank et al. 1973; Feldmesser et al. 2001; Love et al. 1985) that appear to be resistant to phagocytosis (Okagaki et al. 2010). These giant cells are now recognised as a distinct cell morphology during infection and are now often called “Titan cells” in the literature. The size of reported Titan cells differs between studies and ranges from around 25 μm to 100 μm, making their volume up to 900 times greater than that of normal cryptococci (Zaragoza et al. 2010). The underlying mechanism or stimuli behind Titan cell development is not known, although the presence of macrophages, or spent media used to grow macrophages, can induce the morphology (Okagaki et al. 2010) suggesting that interactions with the macrophage play at least some role in the process.
Titan cells appear to be more resistant to phagocytosis than normally sized cells. The most obvious defence a Titan cell has against a macrophage is its physical size. However, recent work has suggested that the presence of Titan cells during infection may also protect neighbouring wild-type cells from phagocytosis, suggesting the presence of a secreted inhibitory factor (Okagaki et al. 2010; Okagaki and Nielsen 2012).
In summary, the detection and phagocytosis of C. neoformans cells is an important part of the immune response against the fungus. To protect itself from phagocytosis, C. neoformans relies heavily on its polysaccharide capsule but also deploys a range of other antiphagocytic strategies. Such mechanisms appear effective at helping some cells evade phagocytosis, but nonetheless the infection of macrophages in vitro and in vivo is often observed, indicating that phagocytosis of C. neoformans is far from rare.
V. Life Within the Phagosome
To grow inside a macrophage following phagocytosis, an intracellular pathogen must successfully avoid the killing mechanisms that develop in the phagosome during phagosomal maturation. A number of mechanisms are employed by pathogens to do this, including inhibition of V-ATPase to prevent phagosomal acidification as seen with Mycobacterium tuberculosis infection (Huynh and Grinstein 2007) and escape from the phagosome into the cytoplasm as seen with Listeria monocytogenes infection (Hamon et al. 2006). In contrast to such pathogens, C. neoformans is able to survive within the phagosome seemingly without modifying the maturation process. Instead, it is able to resist and overcome the destructive conditions it encounters in the phagosome and can even grow within this harsh environment.
The first barrier to cryptococcal growth in mammalian macrophages is the higher temperature within the host than in the environment. The complete molecular mechanisms that allow C. neoformans to grow at 37 °C are not fully known, but a number of potential pathways have been implicated. For instance, higher temperatures induce upregulation of TSP1 and TSP2, which together lead to higher levels of the polysaccharide trehalose, protecting cellular proteins from denaturation (Petzold et al. 2006).
Inside the phagosome, the largest barrier to cryptococcal growth is phagosomal maturation, i.e. the production of oxygen free radicals accompanied by the acidification of the phagosome. During infection most C. neoformans strains synthesise melanin, which is a dark-coloured pigment and an antioxidant (Wang et al. 1995) that is thought to protect the fungi from reactive oxygen species. In addition to melanin, capsule enlargement during macrophage infection may also be protective against oxygen free radicals by creating a barrier between the cell body and phagosomal contents (Zaragoza et al. 2008); furthermore, GXM within the capsule may act as an antioxidant (Monari et al. 2006; Vecchiarelli et al. 1996). Interestingly, however, the phagosomal acidification that occurs during maturation may actually be beneficial to C. neoformans because neutralising phagosomal pH can block intracellular replication (Levitz et al. 1999).
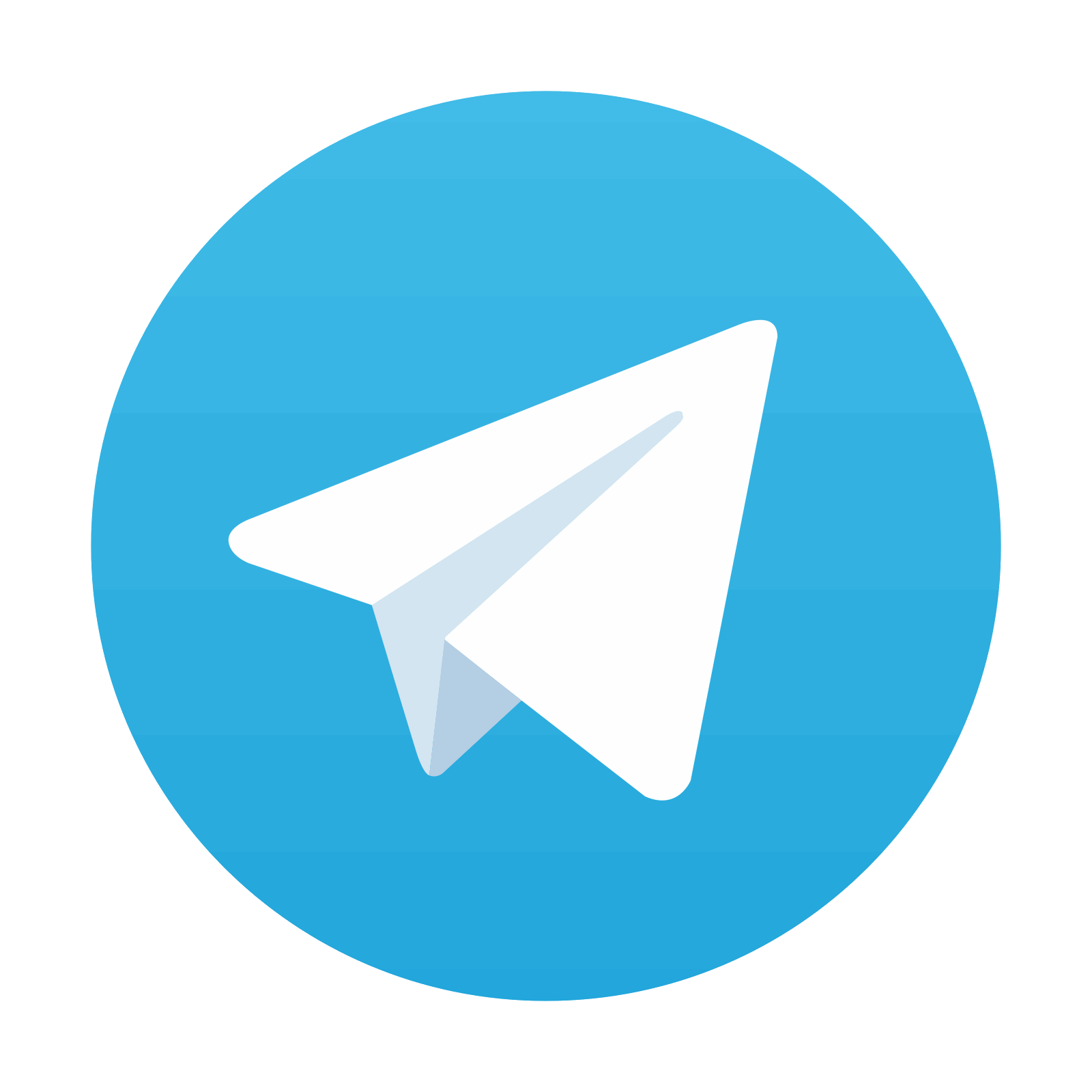
Stay updated, free articles. Join our Telegram channel

Full access? Get Clinical Tree
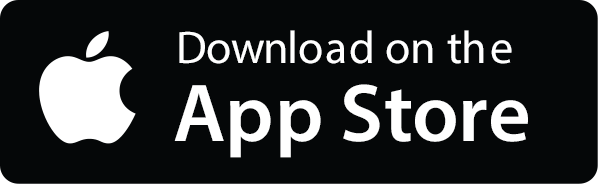
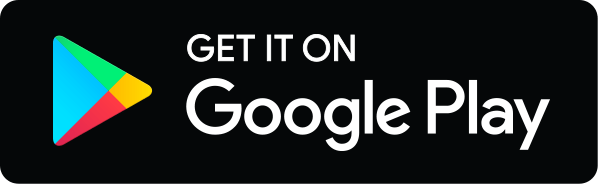