Lipid Disorders
Daniel J. Rader
Overview
Lipoprotein disorders are common and are an important risk factor for coronary artery disease (CAD) and all types of atherosclerotic vascular disease (ASCVD). Intervention with diet and drugs to reduce low-density-lipoprotein (LDL) cholesterol has been proven to decrease the risk of subsequent cardiovascular events, including total mortality. In patients with established ASCVD or at high risk for developing it, treatment to reduce LDL-C is effective not only when the LDL-C level is elevated, but also when the cholesterol level is average. Based on the wealth of clinical trial data, it is widely agreed that virtually all patients with CAD should be treated aggressively with lipid-modifying drug therapy to reduce their risk of subsequent cardiovascular events. Unfortunately, sudden cardiac death at the time of the first myocardial infarction (MI) is common, and therefore waiting to initiate lipid-modifying therapy until symptoms of CAD have developed is not an acceptable strategy. Therefore, it is also generally agreed that patients who have a level of risk that is roughly equivalent to that of patients with established CAD should also be treated aggressively with lipid-modifying therapy. This category includes patients who have other forms of ASCVD (carotid disease, peripheral vascular disease), patients with diabetes mellitus, and patients who have an absolute risk of having a cardiovascular event over the next 10 years of greater than 20%. Finally, lipid-modifying drug therapy has been shown to reduce cardiovascular events even in less-high-risk patients. Therefore, a major challenge in the use of lipid-modifying drug therapy in the primary prevention setting is performing an accurate risk assessment so that patients can be treated with an appropriate level of lipid-modifying drugtherapy. In addition to standard risk assessment based on traditional risk factors, the diagnosis of the metabolic syndrome may identify patients at higher risk. There may be a clinical role for additional tests to assess cardiovascular risk, such as laboratory tests and noninvasive imaging of subclinical atherosclerosis. LDL-C is not the only lipid parameter of interest, and triglycerides, non–high-density-lipoprotein (HDL) cholesterol, and HDL cholesterol are also of tremendous interest. Lipid-modifying therapy is a cornerstone of cardiovascular risk reduction. Strategies for achieving appropriate modification of plasma lipids and lipoproteins must include both broad-based public health measures oriented around diet and lifestyle and
highly focused, aggressive intervention targeted toward those at highest risk for developing ASCVD.
highly focused, aggressive intervention targeted toward those at highest risk for developing ASCVD.
Glossary
Apolipoprotein
Major protein component of lipoproteins; there are multiple apolipoproteins with functions.
Cholesteryl ester transfer protein (CETP)
Protein that transfers lipids among lipoproteins, especially cholesteryl ester from HDL to very low density lipoprotein (VLDL) in exchange for triglycerides.
Chylomicron
Intestinal triglyceride-rich lipoprotein; elevated when triglycerides are greater than 1,000 mg/dL.
Hepatic lipase (HL)
Endothelial-anchored enzyme in liver primarily responsible for hydrolysis of triglycerides and phospholipids in intermediate-density lipoprotein (IDL) and HDL.
High-density lipoprotein (HDL)
Small lipoprotein thought to participate in “reverse cholesterol transport;” levels are inversely associated with CAD risk.
Hydroxy-3-methylglutaryl coenzyme A (HMG-CoA) reductase
Rate-limiting enzyme in cholesterol biosynthesis; inhibition by “statins” results in reduction of plasma VLDL-cholesterol levels.
Intermediate-density lipoprotein (IDL)
Lipoprotein formed by hydrolysis of triglycerides in VLDL; elevated in type III hyperlipoproteinemia.
Lecithin:cholesterol acyltransferase (LCAT)
Enzyme that converts free cholesterol to cholesteryl ester on HDL.
Lipoprotein
Complex responsible for transporting lipids (cholesterol, triglyceride, phospholipids) within the blood.
Lipoprotein lipase (LPL)
Endothelial-anchored enzyme primarily responsible for hydrolysis of chylomicron and VLDL triglycerides, especially in muscle and adipose tissue.
Low-density lipoprotein (LDL)
Major cholesterol-containing lipoprotein and major atherogenic lipoprotein.
Primary prevention
Treatment for preventing atherosclerosis and CAD events in persons who do not have evidence of CAD.
Secondary prevention
Treatment for preventing recurrent CAD events in persons who have documented CAD.
Very low density lipoprotein (VLDL)
Major triglyceride-containing lipoprotein when fasting triglycerides are less than 1,000 mg/dL; made by the liver.
Historical Perspective
The association between elevated serum cholesterol and coronary heart disease (CHD) was first reported in the 1930s, and subsequent large epidemiologic studies confirmed the strong relationship between serum cholesterol and CHD. These studies formed the basis of the “cholesterol hypothesis” that the relationship between serum cholesterol and atherosclerosis is causal and that reduction of serum cholesterol would reduce atherosclerotic disease.
The Subfractionation of serum cholesterol into cholesterol contained within specific lipoproteins such as LDLs and HDLs was a major clinical advance. Cardiovascular risk was found to be positively associated with LDL-cholesterol (LDL-C) levels and inversely with HDL-C levels. The relationship between fasting serum triglycerides and cardiovascular risk has been confounded by the inverse association between triglycerides and HDL-C, as well as by the association of triglycerides with other risk factors such as diabetes mellitus and body mass. However, triglyceride levels are now considered an important independent predictor of cardiovascular risk.
Lipid-Modifying Therapy and Effects on Atherosclerotic Cardiovascular Events
Despite the wealth of epidemiologic and pathologic data linking serum cholesterol to atherosclerotic disease, proof of the cholesterol hypothesis required demonstration that reduction of cholesterol decreased atherosclerosis and clinical cardiovascular events. Over the last three decades, multiple trials have been reported that were designed to test this hypothesis.
Early Clinical Trials: Niacin, Bile Acid Sequestrants, and Partial Ileal Bypass
The early clinical trials used niacin, bile acid sequestrants, and even the surgical approach of partial ileal bypass to reduce serum cholesterol levels. The Coronary Drug Project demonstrated a modest benefit of niacin in reducing nonfatal MI after 6 years of treatment (1) and in reducing total mortality after 15 years of follow-up (2). The Program on the Surgical Control of Hyperlipidemias (POSCH) trial employed the surgical technique of partial ileal bypass surgery to reduce LDL-C levels and demonstrated a significant 35% relative reduction in fatal CHD and nonfatal MI, although not in total mortality (the primary endpoint of the trial) (3). In the Lipid Research Clinics Coronary Primary Prevention Trial (LRC-CPPT) with cholestyramine in hypercholesterolemic men (4,5), combined fatal CHD and nonfatal MI (the primary endpoint) was reduced by 19%.
3-Hydroxy-3-methylglutaryl Coenzyme A Reductase Inhibitor (Statin) Trials
The cholesterol hypothesis was finally definitively proven as a result of the clinical outcome trials with hydroxy-3-methylglutaryl-coenzyme A (HMG-CoA) reductase inhibitors (statins). In the Scandinavian Simvastatin Survival Study (4S) (6), 4,444 patients with CHD and elevated cholesterol were randomized to placebo or simvastatin 20 mg titrated to 40 mg as needed. Treatment with simvastatin was associated with a 30% relative risk reduction in total mortality and a 44% reduction in CHD death or MI. It is important that it was not only the subjects with the highest cholesterol levels who experienced a reduction in risk; the quartile with the lowest LDL-C levels at baseline had proportionately as much benefit from treatment as the highest quartile (7). This is important because least one third of persons with CHD have total cholesterol levels less than 200 mg/dL (8). In the Cholesterol and Recurrent Events (CARE) study (9), 4,159 patients who were post-MI and had total cholesterol levels less than 240 mg/dL were randomized to placebo or pravastatin 40 mg daily and followed for an average of 5 years. Treatment with pravastatin was associated with a significant 24% reduction in relative risk. In the Long-Term Intervention with Pravastatin in Ischemic Disease (LIPID) trial (10), 9,014 patients with CHD and a broad range of cholesterol levels were randomized to placebo or pravastatin 40 mg daily and followed for an average of 6 years. Treatment with pravastatin significantly reduced CHD mortality by 24% and total mortality by 22%. These three statin studies in patients with preexisting CHD reported in the 1990s definitively established the efficacy of statin therapy in reducing cardiovascular events and total mortality in patients with established CHD over a very wide range of baseline LDL-C levels.
Two additional large outcome studies with statins in the 1990s were carried out in patients without CHD. In the West of Scotland Coronary Prevention Study (WOSCOPS) (11), 6,595 healthy Scottish men with total cholesterol levels of less than 252 mg/dL were randomized to placebo or pravastatin 40 mg/day and followed for an average of 5 years. Treatment with pravastatin was associated with a significant 31% reduction in relative risk of nonfatal MI or CHD death. The Air Force/Texas Coronary Atherosclerosis Prevention Study (AFCAPS/TexCAPS) (12) extended these findings into a population with modestly elevated to average cholesterol levels. A total of 6,608 men and women with LDL-C level 130 to 190 mg/dL and relatively low HDL-C levels were randomized to lovastatin 20 to 40 mg or placebo and followed for an average of 5.2 years. Treatment with lovastatin was associated with a 37% relative risk reduction in the primary endpoint of combined cardiovascular events in the lovastatin-treated group. These studies definitively confirmed that the benefits of cholesterol reduction extend to the primary prevention setting.
Subsequent studies have focused less on the issue of primary versus secondary prevention and instead have defined groups of patients based on their future risk of CHD events. The Heart Protection Study (HPS) was a more recent study in which 20,536 patients aged 40 to 80 years old with CHD, other atherosclerotic vascular disease, or diabetes were randomized to simvastatin 40 mg or placebo (13). For inclusion, total cholesterol needed only be greater than 135 mg/dL, ensuring that many subjects in this trial had average and even below-average cholesterol levels. Treatment with simvastatin was associated with a highly significant 24% reduction in major coronary events, 25% reduction in stroke, and 13% reduction in total mortality. Remarkably, the relative benefit of simvastatin therapy was similar across tertiles of baseline LDL-C, and even the group with LDL-C less than 100 mg/dL at baseline demonstrated benefit with simvastatin. The results of HPS led to the widespread concept that there are benefits of statin therapy in high-risk individuals regardless of baseline cholesterol levels.
In the Prospective Study of Pravastatin in the Elderly at Risk (PROSPER), 5,804 patients aged 70 to 80 years with vascular disease or risk factors were randomized to pravastatin 40 mg or placebo and followed for an average of 3.2 years (14). Pravastatin treatment was associated with a significant 15% reduction in a composite cardiovascular endpoint, demonstrating the benefit of statin therapy in the elderly. In the Antihypertensive and Lipid-Lowering Treatment to Prevent Heart Attack Trial (ALLHAT), 10,355 hypertensive patients were randomized to pravastatin 40 mg or usual care (in which up to about 30% of patients were receiving statin therapy prescribed by their physician by the end of the trial) (15). There was a trend but not a statistically significant reduction in coronary events associated with randomization to pravastatin in this trial, but the difference between the two groups in total cholesterol was only 9%. In the Anglo-Scandinavian Cardiac Outcomes Trial Lipid-lowering Arm (ASCOT-LLA), 19,342 hypertensive patients with at least three other risk factors and with total cholesterol levels less than 242 mg/dL were randomized to atorvastatin 10 mg or placebo (16). The study was stopped early after 3.3 years when a highly significant 36% relative risk reduction associated with atorvastatin was found. In the Collaborative Atorvastatin Diabetes Study (CARDS), 2,838 patients with type 2 diabetes were randomized to atorvastatin 10 mg or placebo (17). This study was also terminated early due to a significant 37% reduction in major cardiovascular events.
The first trial to directly compare two different doses of the same statin with regard to cardiovascular (CV) outcomes was the Treat to New Targets (TNT) trial (18). A total of 10,001 patients with CHD and LDL-C less than 130 mg/dL were randomized to atorvastatin 10 mg or 80 mg daily. The higher dose of atorvastatin resulted in a mean on-treatment LDL-C of 77 mg/dL (compared with 101 mg/dL for the lower dose) and a significant 22% reduction in major cardiovascular events. This trial conclusively proved that a higher dose of atorvastatin reduced CV events to a greater extent than a lower dose. The studies from HPS to TNT reviewed here substantially moved the field toward a “lower is better” approach to reducing LDL-C.
Statin trials have also been performed in patients presenting with acute coronary syndromes (ACS). In the Myocardial Ischemia Reduction with Aggressive Cholesterol Lowering (MIRACL) trial, patients presenting with ACS were randomized to immediate therapy with atorvastatin 80 mg or placebo and followed for 4 months (19). There was a 16% reduction in recurrent coronary events associated with immediate atorvastatin therapy that just achieved statistical significance (p = .48). Two more-recent ACS studies compared different statin regimens or doses and followed subjects for a longer period of time. In the Pravastatin or Atorvastatin Evaluation and Infection Therapy (PROVE-IT) study, ACS patients were randomized to atorvastatin 80 mg or pravastatin 40 mg and followed for a mean of 2 years (20). The more intensive regimen of atorvastatin 80 mg (mean on-treatment LDL-C of 62 mg/dL) was associated with a significant 16% relative risk reduction in major cardiovascular events compared with the less intensive pravastatin 40 mg regimen (mean on-treatment LDL-C of 95 mg/dL). In contrast, the Aggrastat to Zocor (A to Z) trial randomized ACS patients to simvastatin 40 mg versus placebo for 4 months, followed by titration to simvastatin 80 mg versus simvastatin 20 mg, and followed subjects for up to 2 years (21). This study failed to demonstrate a significant difference between the two arms with regard to the primary endpoint, but did demonstrate reduction in cardiovascular events during the follow-up period starting at 4 months. Overall the ACS studies have resulted in the standard practice of starting a statin in the hospital prior to discharge in patients admitted for ACS.
Fibrate Trials
There have been fewer clinical endpoint trials with fibrates than with statins. The Helsinki Heart Study (HHS) compared gemfibrozil 1,200 mg to placebo in men with elevated non-HDL-C greater than 200 mg/dL and demonstrated a significant 34% reduction in combined fatal and nonfatal MI (the primary endpoint) but no difference in total mortality (22). The Veteran Affairs High-Density Lipoprotein Cholesterol Intervention Trial (VA-HIT) tested the benefit of gemfibrozil 1,200 mg versus placebo in patients with CHD and average LDL-C levels (mean 112 mg/dL) but low HDL-C levels (mean 32 mg/dL) (23). Gemfibrozil therapy was associated with a significant 22% reduction in the primary endpoint (nonfatal myocardial infarction and coronary death) compared to placebo. In contrast, the Bezafibrate Infarction Prevention (BIP) trial in patients with established CHD, LDL-C less than 180 mg/dL and HDL-C less than 45 mg/dL randomized to either bezafibrate 400 mg daily or placebo (24) failed to demonstrate a significant reduction in the primary endpoint of fatal or nonfatal MI. A post hoc analysis in the subgroup with high baseline triglycerides (>200 mg/dL) suggested a reduction in events of 39%. These two latter trials differed in a number of important ways, including the fibrate used and the baseline LDL-C levels, which in BIP were 150 mg/dL compared with only 111 mg/dL in VA-HIT. A reasonable conclusion might be that fibrates are effective as monotherapy in reducing events in secondary prevention if the LDL-C is low, but not if it is elevated.
Physiology and pathophysiology of lipoprotein metabolism
Appropriate clinical management of patients with lipid disorders requires a general working knowledge of normal lipoprotein metabolism. Lipoproteins are large macromolecular complexes that transport cholesterol and triglycerides within the blood. They contain a hydrophobic neutral lipid core consisting of triglycerides and cholesteryl esters, which is surrounded by phospholipids and specialized proteins known as apolipoproteins. Apolipoproteins are required for the structural integrity of lipoproteins and direct their metabolic interactions with enzymes, lipid transport proteins, and cell surface receptors. The five major families of lipoproteins are chylomicrons, very low density lipoproteins, intermediate-density lipoproteins, low-density lipoproteins, and high-density lipoproteins Chylomicrons are the largest and most lipid-rich lipoproteins, whereas HDL are the smallest lipoproteins and contain the least amount of lipid. The major metabolic pathways of lipoproteins are shown in Figures 5.1,5.2,5.3 and are described in detail in the accompanying legends.
Disorders of lipoprotein metabolism involve perturbations that cause elevation of triglycerides and/or cholesterol, reduction of HDL-C, or alteration of properties of lipoproteins such as their size or composition. These perturbations can be genetic (primary) or occur as a result of other diseases, conditions, or drugs (secondary) (Table 5.4). Many (but not all) lipoprotein disorders have as their most important clinical consequence the increased risk of premature atherosclerotic cardiovascular disease. The next section of this chapter will focus specifically on the identification and diagnosis of specific lipid disorders, and the subsequent section will focus on the management of lipid disorders, especially regarding the prevention of atherosclerosis and its associated clinical events.
TABLE 5.1 Selected disorders associated with secondary dyslipidemia | ||||||||||||||||||||||||||||||||||||||||||||||||||||||||||||||||||||||||||||
---|---|---|---|---|---|---|---|---|---|---|---|---|---|---|---|---|---|---|---|---|---|---|---|---|---|---|---|---|---|---|---|---|---|---|---|---|---|---|---|---|---|---|---|---|---|---|---|---|---|---|---|---|---|---|---|---|---|---|---|---|---|---|---|---|---|---|---|---|---|---|---|---|---|---|---|---|
|
The mechanisms by which atherogenic lipoproteins promote atherosclerosis continue to be the subject of intensive research. In brief, LDL and other atherogenic lipoproteins probably must be aggregated, oxidized, or otherwise modified in order to be recognized by macrophage cell-surface receptors and be internalized, leading to foam cell formation (25). Atherogenic lipoproteins may influence macrophage function in ways that go beyond foam cell formation that also promote atherogenesis (26). On the other hand, HDL is believed to promote cholesterol efflux from macrophages and return it to the liver for excretion in the bile, a process known as reverse cholesterol transport (27). HDL may also have antioxidant and antiinflammatory effects that contribute to its antiatherogenic effects (28).
Secondary disorders of lipoprotein metabolism
Lipid disorders can be grouped into primary disorders (genetic or inherited) and secondary disorders (due to another disease or environmental factor). Clinically, it is useful to first consider and exclude secondary causes because they should be treated if found to be present. Some of the most important secondary causes of hyperlipidemia are reviewed briefly in what follows and are listed in Table 5.1.
Hypothyroidism
Hypothyroidism causes elevated LDL-C levels due primarily to downregulation of the LDL receptor. Because hypothyroidism can be subtle in its clinical presentation, patients presenting with hypercholesterolemia should be screened with a thyroid-stimulating hormone (TSH) test to rule out hypothyroidism. Thyroid replacement therapy usually results in resolution of the hypercholesterolemia. Hypothyroid patients who remain hypercholesterolemic after adequate replacement probably have an underlying lipoprotein disorder and may require lipid-lowering drug therapy.
Diabetes Mellitus and Insulin Resistance
Patients with type II diabetes mellitus (and insulin resistance even in the absence of overt diabetes) frequently have associated dyslipidemia (29). Insulin resistance results in impaired capacity to catabolize chylomicrons and VLDL, as well as excess hepatic triglyceride and VLDL production. Type II diabetes mellitus, insulin resistance, and glucose intolerance are often associated with a constellation of lipid abnormalities including elevated triglycerides and VLDL-C, increased small, dense LDL, and decreased HDL-C. Occasionally, the triglycerides can be extremely elevated (>1,000 mg/dL) and predispose to acute pancreatitis. Significant elevation of LDL-C in the diabetic patient often suggests the presence of an additional lipoprotein abnormality. All patients presenting with hyperlipidemia should be screened for diabetes using a fasting glucose. Aggressive control of diabetes often results in improved control of hyperlipidemia. Furthermore, hyperlipidemia is a major risk factor for diabetic patients, and lipid disorders in diabetes should be aggressively treated in order to decrease the risk of cardiovascular disease.
Renal Diseases
Chronic renal insufficiency and especially end-stage renal disease are often associated with moderate hypertriglyceridemia due to a defect in triglyceride lipolysis and remnant clearance (30). Nephrotic syndrome is associated with a more pronounced hyperlipidemia involving both elevated triglycerides and cholesterol due to hepatic overproduction of VLDL. Both types of renal disease should be considered and excluded if suspected in patients presenting with hyperlipidemia. Resolution of the nephrotic syndrome improves the lipid profile, but patients with chronic nephrotic syndrome often require lipid-lowering drug therapy. Although the lipid abnormalities in end-stage renal disease are more modest, cardiovascular risk is high, and lipid-modifying drug therapy should be seriously considered. There has also been interest in the concept that atherogenic lipoproteins are nephrotoxic and lipid-lowering therapy might slow the progression of chronic renal insufficiency.
Alcohol
Alcohol intake often exacerbates hyperlipidemia, but its effects are highly variable. The greatest effects of alcohol are on triglyceride levels. Alcohol consumption inhibits oxidation of free fatty acids by the liver, which stimulates hepatic triglyceride synthesis and secretion of VLDL. The usual lipoprotein pattern associated with alcohol consumption is moderate hypertriglyceridemia, although it can raise total and LDL-C levels as well. Regular alcohol use also raises the HDL-C level by a mechanism that is not completely understood. Patients with hyperlipidemia who drink alcohol regularly should be advised to reduce their alcohol intake.
TABLE 5.2 Frederickson and Levy classification of hyperlipoproteinemia phenotypes | |||||||||||||||||||||||||||||||||||||||||||||||||||||||||||||||
---|---|---|---|---|---|---|---|---|---|---|---|---|---|---|---|---|---|---|---|---|---|---|---|---|---|---|---|---|---|---|---|---|---|---|---|---|---|---|---|---|---|---|---|---|---|---|---|---|---|---|---|---|---|---|---|---|---|---|---|---|---|---|---|
|
Drugs
Several drugs are known to influence plasma lipid levels and are listed in Table 5.1. Retinoids such as isotretinoin and etretinate used for acne are known to induce substantial hypertriglyceridemia in susceptible persons. Immunosuppressive therapy used in the posttransplantation setting is well known to cause both hypercholesterolemia and hypertriglyceridemia. Finally, since the introduction of the protease inhibitors for therapy for HIV, it has become recognized that many of these patients develop hyperlipidemia, which appears to result from a complex and poorly understood interaction between the disease and the effect of the drugs.
Primary (Genetic) Disorders of Apolipoprotein B–Containing Lipoproteins Causing Hyperlipoproteinemia
The classification of lipoprotein disorders is useful as a guide to accurate diagnosis and effective treatment. The Frederickson and Levy classification (Table 5.2) is based on the type of lipoprotein that is elevated and has been used for several decades. It is now gradually being replaced by a classification based on the understanding of the molecular etiology and pathophysiology of the lipoprotein disorders (Table 5.3). In this section, lipoprotein disorders are presented in a way intended to facilitate a practical clinical approach. This approach is based on classifying patients first according to triglyceride levels and can lead to a more rational approach to differential diagnosis and choice of appropriate therapy.
TABLE 5.3 Primary (genetic) hyperlipoproteinemias | |||||||||||||||||||||||||||||||||||||||||||||||||||||||||||||||||||||||||||||
---|---|---|---|---|---|---|---|---|---|---|---|---|---|---|---|---|---|---|---|---|---|---|---|---|---|---|---|---|---|---|---|---|---|---|---|---|---|---|---|---|---|---|---|---|---|---|---|---|---|---|---|---|---|---|---|---|---|---|---|---|---|---|---|---|---|---|---|---|---|---|---|---|---|---|---|---|---|
|
Disorders Associated with Triglycerides Greater Than 1,000 mg/dL
Severe hypertriglyceridemia (fasting triglycerides >1,000 mg/dL) is virtually always an indication of hyperchylomicronemia in the fasting state and points to an underlying genetic predisposition, often exacerbated by another medical condition or a hormonal or environmental factor. The major clinical complication of severe hypertriglyceridemia is acute pancreatitis, and initial treatment is focused on decreasing the triglycerides below 1,000 mg/dL to prevent this serious complication. In addition, some but not all patients with severe hypertriglyceridemia are at risk for premature atherosclerotic CVD and require more aggressive therapy even once the triglycerides have been decreased below the 1,000-mg/dL threshold. The major disorders associated with severe hypertriglyceridemia are discussed in the following subsections.
Familial Chylomicronemia Syndrome: Lipoprotein Lipase Deficiency and Apolipoprotein C-II Deficiency
The familial hyperchylomicronemia syndrome is characterized by presentation in childhood with acute pancreatitis in the setting of triglyceride levels greater than 1,000 mg/dL. Recurrent abdominal pain is a common feature in children with this disorder. On physical exam, eruptive xanthomas (small papular lesions that occur in showers on the buttocks and back) are often seen. Lipemia retinalis (a pale appearance to the retinal veins) is a clue to the existence of severe hypertriglyceridemia, and hepatosplenomegaly due to ingestion of chylomicrons by the reticuloendothelial system is often found. Premature atherosclerotic cardiovascular disease is not a feature of this disease.
Two different genetic defects can cause the familial hyperchylomicronemia syndrome: lipoprotein lipase (LPL) deficiency and apolipoprotein C-II (apoC-II) deficiency. The hydrolysis of triglycerides in chylomicrons requires the action of LPL in tissue capillary beds, and apoC-II is a required cofactor for the activation of LPL (Fig. 5.1). Mutations in either the LPL gene or the apoC-II gene result in functional deficiency of LPL, inability to hydrolyze triglycerides in chylomicrons, and consequent massive hyperchylomicronemia. The disorder is autosomal recessive, meaning that both alleles of the LPL or apoC-II gene must be affected for the disorder to be present. Therefore, the parents of children with this disorder generally have normal or near-normal triglyceride levels. Both are rare disorders, but of the two, LPL deficiency is much more common (approximately 1 in 1 million persons) than apoC-II deficiency.
The diagnosis of the familial hyperchylomicronemia syndrome is usually made based on the clinical presentation and some key laboratory features. The plasma is often lactescent
plasma, and after overnight refrigeration a cake of chylomicrons forms on the surface. Triglyceride levels are usually greater than 1,000 mg/dL and may be as high as 10,000 mg/dL or greater. Total cholesterol levels are also elevated due to the presence of cholesterol in chylomicrons. Lipoprotein electrophoresis demonstrates markedly elevated chylomicrons at the origin, but is not essential for making the diagnosis. The diagnosis of LPL deficiency can be confirmed at specialized centers by the measurement of LPL activity in the plasma after intravenous heparin injection (postheparin LPL activity). Patients with suspected familial hyperchylomicronemia syndrome should be referred to a specialized lipid center for diagnosis and management.
plasma, and after overnight refrigeration a cake of chylomicrons forms on the surface. Triglyceride levels are usually greater than 1,000 mg/dL and may be as high as 10,000 mg/dL or greater. Total cholesterol levels are also elevated due to the presence of cholesterol in chylomicrons. Lipoprotein electrophoresis demonstrates markedly elevated chylomicrons at the origin, but is not essential for making the diagnosis. The diagnosis of LPL deficiency can be confirmed at specialized centers by the measurement of LPL activity in the plasma after intravenous heparin injection (postheparin LPL activity). Patients with suspected familial hyperchylomicronemia syndrome should be referred to a specialized lipid center for diagnosis and management.
The mainstay of therapy for familial hyperchylomicronemia syndrome is restriction of total dietary fat. Consultation with a registered dietician familiar with this disorder is essential. Caloric supplementation with medium-chain triglycerides, which are absorbed directly into the portal vein and therefore do not promote chylomicron formation, can be useful if necessary. If dietary fat restriction alone is not successful, some patients may respond to a trial of fish oils or fibrates. For patients with apoC-II deficiency, an attack of acute pancreatitis can be treated with infusion of fresh-frozen plasma to provide apoC-II in an attempt to clear severe hypertriglyceridemia and promote resolution of the pancreatitis.
Familial Type V Hyperlipoproteinemia
Type V hyperlipoproteinemia (HLP) is a common diagnosis, which continues to use the nomenclature of the Frederickson classification system. The label of type V HLP is generally used for an adult with triglyceride levels greater than 1,000 mg/dL who does not have known familial chylomicronemia syndrome due to LPL or apoC-II deficiency (see prior discussion). Type V HLP is also associated with risk of acute pancreatitis, which can be the initial presentation of this syndrome and is the major rationale for aggressive treatment of this condition. Type V HLP can also be associated with increased risk of cardiovascular disease, although some patients with type V HLP do not appear to be at significantly increased risk.
Most but not all patients with type V HLP have a family history of hypertriglyceridemia, although specific genetic mutations causing type V HLP have not yet been identified. Type 2 diabetes mellitus or glucose intolerance frequently accompanies type V hyperlipidemia, but type V also occurs in people with normal glucose tolerance. Some patients with nephrotic syndrome can develop severe hypertriglyceridemia. Estrogen replacement therapy, oral contraceptives, isotretinoin or etretinate, and alcohol use can exacerbate moderate hypertriglyceridemia and lead to severe hypertriglyceridemia and even acute pancreatitis. Rarely, patients with familial dysbetalipoproteinemia (type III hyperlipidemia; see later discussion) can have triglycerides greater than 1,000 mg/dL, particularly if another factor is superimposed on the apoE mutation. The diagnostic evaluation of an adult patient presenting with triglycerides greater than 1,000 mg/dL should be focused on a search for underlying predisposing factors and an attempt to establish a history of complications (pancreatitis and cardiovascular disease). A comprehensive personal and family history should be obtained and all medications should be reviewed. On physical exam, lipemia retinalis and tiny eruptive xanthomas on the back or buttocks are the most specific findings for type V hyperlipidemia. Diabetes and renal disease should always be excluded as potential contributing factors. No specific laboratory tests are required for the diagnosis of type V except possibly lipoprotein centrifugation to exclude familial dysbetalipoproteinemia (see later discussion).
The management of type V hyperlipidemia is first targeted to decreasing triglycerides in order to reduce the risk of pancreatitis, followed by further lipid lowering depending on the presence of CAD or other risk factors for cardiovascular disease. Women taking estrogens and patients taking isotretinoin or etretinate should be encouraged to discontinue them if triglycerides are greater than 1,000 mg/dL. Diabetes mellitus should be controlled as optimally as possible. Patients should be referred to a registered dietician for dietary counseling. In general, dietary management includes restriction of total fat as well as simple sugars in the diet. Alcohol should be avoided. Regular aerobic exercise can have a significant effect on triglyceride levels and should be actively encouraged. If the patient is overweight, weight loss can help to decrease triglycerides as well.
When fasting triglyceride levels remain greater than 1,000 mg/dL despite institution of appropriate dietary and lifestyle measures and control of secondary causes, drug therapy must be considered in order to decrease the risk of acute pancreatitis. Drug therapy is usually straightforward, although a subset of patients is remarkably resistant to standard treatment. There are three major drug classes for consideration in treating very high triglycerides: fibrates, nicotinic acid, and fish oils (see later discussion). In practice, fibrates are generally recommended as the first-line agent. Nicotinic acid or fish oils should be considered for patients who fail to respond adequately to fibrates.
Frequently, once the triglycerides are adequately controlled, patients remain significantly hypercholesterolemic. This often raises the difficult issue of the need for a second medication to better control the LDL-C. The National Cholesterol Education Program (NCEP) guidelines represent a useful guide to the decision to institute further drug therapy in this setting (31). If the triglyceride levels are greater than 200 mg/dL, the non-HDL-C should be calculated by subtracting the HDL-C from the total cholesterol. Targets for non-HDL-C are 30 mg/dL higher than LDL-C targets. Many type V patients who have had their triglycerides managed are candidates for the addition of a statin to further reduce LDL-C and non-HDL-C levels. Although it is important to recognize that there is an increased risk of myopathy associated with the combination of fibrate and statin, the risk can be minimized as described later.
Disorders Associated with Triglycerides 200 to 1,000 mg/dL
Fasting triglyceride levels less than 1,000 mg/dL are not generally associated with risk of acute pancreatitis, although patients with fasting TG greater than 500 mg/dL can often achieve TG levels greater than 1,000 mg/dL after a meal and therefore remain at some risk for pancreatitis. However, the importance of elevated triglyceride levels in the 200- to 1,000-mg/dL range is mostly related to their potential association with risk of atherosclerotic CVD. There has been increasing recognition of the importance of elevated fasting triglycerides in this range as an independent cardiovascular risk factor. The major primary causes of elevated triglycerides in the 200- to 1,000-mg/dL range are familial hypertriglyceridemia, familial dysbetalipoproteinemia (type III hyperlipidemia), and familial combined hyperlipidemia (FCHL). It is important to differentiate among them because familial dysbetalipoproteinemia and FCHL are both definitely associated with increased risk of premature atherosclerosis, whereas the risk associated with familial hypertriglyceridemia is variable. The clinical approach to a patient with triglycerides in the 200- to 1,000-mg/dL range is generally to rule out secondary causes (and treat them when present), differentiate among the major primary causes, assess the patient for the presence of ASCVD and other cardiovascular risk factors, and manage the lipid disorder based on the clinical assessment of cardiovascular risk. Because there are no clinical endpoint trials based on treatment of hypertriglyceridemia and no formal guidelines for the clinical management of patients with hypertriglyceridemia, the clinical approach to reducing cardiovascular risk remains focused on decreasing LDL-C. However, elevated triglycerides can make the determination of LDL-C difficult, can be associated with increased risk even in the presence of a normal LDL-C, and can complicate the therapeutic approach.
Familial Hypertriglyceridemia
Familial hypertriglyceridemia (FHTG) is a relatively common disorder characterized by moderately elevated triglycerides usually with average or only moderately elevated total cholesterol. FHTG occurs in approximately 1 in 500 persons. It is inherited as an autosomal dominant trait but is not usually expressed until adulthood. The molecular etiology is unknown. VLDL is elevated due either to increased production, impaired catabolism, or a combination of the two. LDL-C is generally not increased in this disorder. Increased intake of simple carbohydrates, a sedentary lifestyle, obesity, insulin resistance, alcohol use, and estrogens can all exacerbate the hypertriglyceridemia.
The diagnosis is suggested by elevated triglyceride levels (200 to 1,000 mg/dL) with normal or only mildly increased cholesterol levels (<240 mg/dL) and almost always a low HDL-C level. Hypertriglyceridemia in at least one first-degree relative is essential in making the diagnosis. It is important to consider and rule out secondary causes of the hypertriglyceridemia. In the differential diagnosis, both familial dysbetalipoproteinemia (type III hyperlipoproteinemia) and familial combined hyperlipidemia (FCHL) should be considered. This is not simply an academic exercise because these two conditions are associated with a significantly increased risk of atherosclerotic vascular disease, whereas FHTG often is not. The total cholesterol level relative to the triglyceride level is usually lower in FHTG compared with familial dysbetalipoproteinemia and FCHL, and the plasma apoB level is usually lower in FHTG than in the other two conditions.
Therapy for FHTG should begin with diet and lifestyle. Intake of simple carbohydrates should be reduced. Regular aerobic exercise can be very effective in decreasing triglyceride levels, as can weight loss. Alcohol use should be discouraged. Diabetes mellitus should be aggressively controlled. Lipid-lowering drug therapy can often be avoided with appropriate diet and lifestyle changes. However, patients who have triglycerides greater than 400 to 600 mg/dL after an adequate trial of diet and exercise should be considered for drug therapy. A fibrate is a reasonable first-line drug for FHTG, but niacin and fish oils can also be considered in this condition.
Familial Dysbetalipoproteinemia (Type III Hyperlipoproteinemia)
Familial dysbetalipoproteinemia is also commonly known as type III hyperlipidemia. It is the best understood of the genetic lipid disorders causing moderate triglyceride elevation and can usually be definitively diagnosed based on a combination of clinical and laboratory parameters. Patients with familial dysbetalipoproteinemia usually present in adulthood with distinctive xanthomas, premature atherosclerosis, or asymptomatic hyperlipidemia discovered on routine screening. Two types of xanthomas are seen in patients with familial dysbetalipoproteinemia. Tuberoeruptive xanthomas begin as clusters of small papules on the elbows, knees, or buttocks and can grow to the size of small grapes. Palmar xanthoma refers to orange-yellow discoloration to the creases of the palms and wrists. Either of these xanthomas is highly suggestive of familial dysbetalipoproteinemia. Premature atherosclerotic CVD is often seen in this disorder. Compared with other lipid disorders, peripheral vascular disease is particularly common in patients with familial dysbetalipoproteinemia.
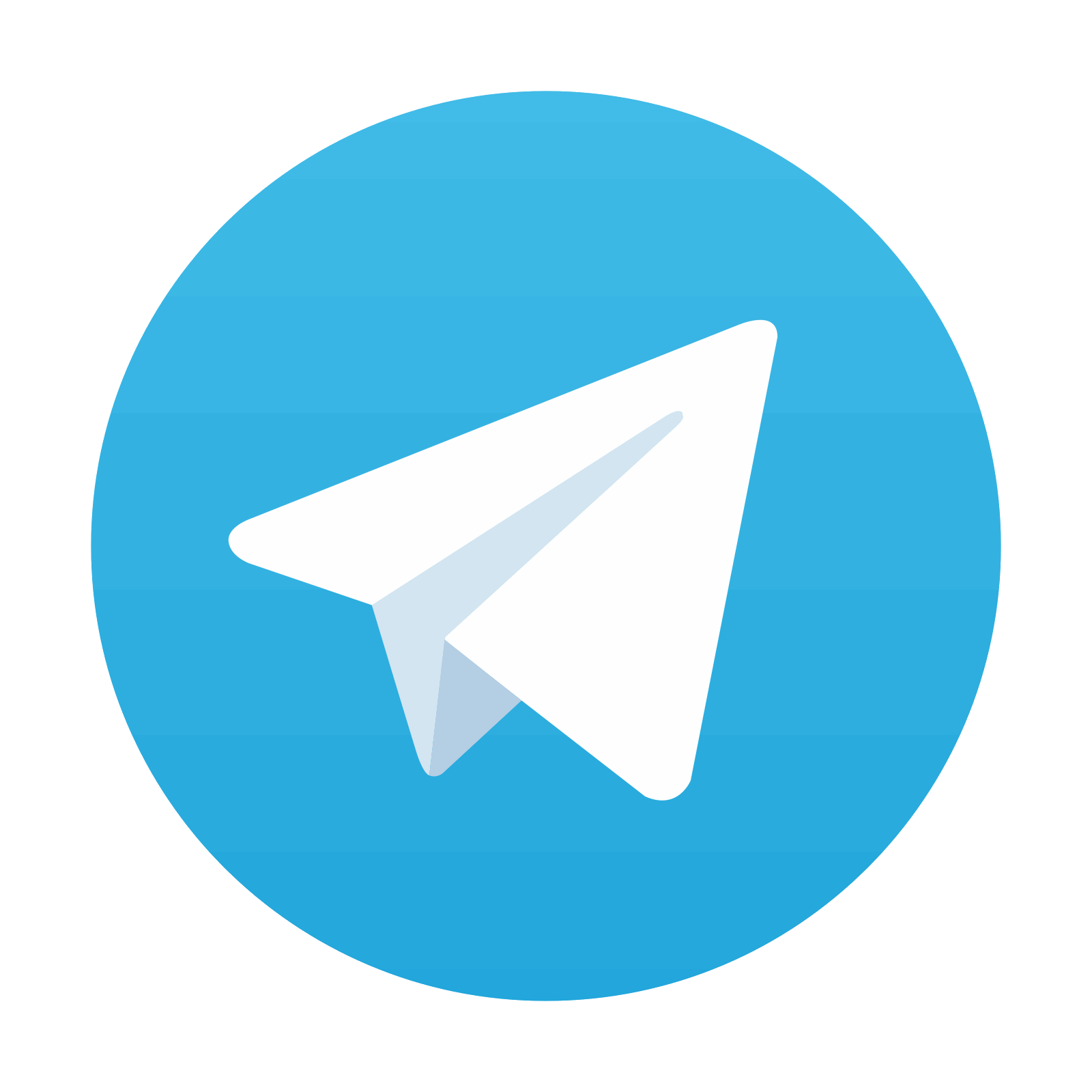
Stay updated, free articles. Join our Telegram channel

Full access? Get Clinical Tree
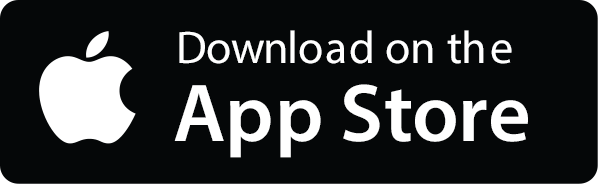
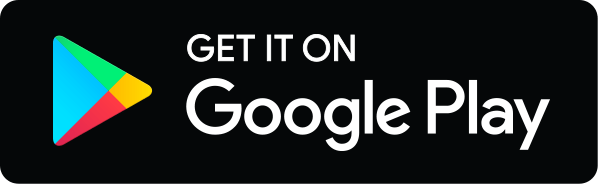
